Abstract
Biosecurity is emerging as one of the most important issues facing the agricultural and forestry communities, with the use of chemical pesticides being the main protocol for controlling plant pathogens. However, as a result of the emergence of resistance against these controls and the negative impact chemicals have on the environment, coupled with more stringent European Union regulations, this will have to change. The search for safer alternatives has begun, with seaweed becoming an interesting focus as a potential biopesticide due to its ability to produce a broad spectrum of chemically active secondary metabolites. These secondary metabolites possess biological properties including antimicrobial, antioxidant, anti-inflammatory, and antifungal. There is a need to develop reliable methods with the ability to rapidly screen and evaluate the potential pesticidal activity in seaweeds. This review focuses on the current methods used to investigate the fungicidal activity of seaweed extracts including in vitro methods namely disk diffusion and in vivo methods namely, the screen-house study and the advantages and disadvantages associated with each method. It was concluded that no one method is suitable for all test organisms or extracts after careful consideration of the literature. Additionally, this review confirms the promising potential of seaweeds as biopesticides with studies demonstrating that seaweeds are active against a wide variety of fungal diseases. It was noted that further research needs to be carried out on the isolation, purification, and identification of the bioactive compounds present in seaweeds in order to facilitate the future potential application of these novel biopesticides.
Similar content being viewed by others
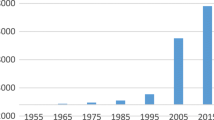
Avoid common mistakes on your manuscript.
Introduction
The economic impact arising from the spread of pests and diseases in plants is a major worldwide issue. The future biosecurity of our estate is being threatened as a result. Irish landscapes are relatively healthy with only a few serious pests threatening individual species. This is mainly due to Ireland island status and strict plant health regulations (McCracken 2013). However, with the increasing movement of plant materials and wood products between countries such as logs, timber pallets, and packing cases, together with the growing movement of people, the likelihood of the spread of more potentially damaging pests and diseases will increase. The international plant trade has been tightly linked to biological invasions (Santini et al. 2013), with more than half of the emerging infectious plant diseases in the last few decades occurring as a result of the plant trade (Bandyopadhyay and Frederiksen 1999). Climate change predictions may also put trees and other plants under increased stress, making them more susceptible to infection from pests and diseases (Tubby and Webber 2010), even from species that are usually considered harmless. The endophytic fungus Biscogniauxia mediterranea (De Not.) Kuntze can cause charcoal disease on drought-stressed cork oaks (Quercus spp.) and other hardwood hosts (Henriques et al. 2015). Additionally, changes in temperature and moisture availability may have a negative impact on the population of certain species, leading to an epidemic increase of certain pests (Tubby and Webber 2010). Therefore, it is clear that controlling plant pathogens is important, especially in the initial stages of invasion. Once a pathogen is established, there are very few treatments that can help a diseased tree to recover (Macpherson et al. 2017). This not only impacts the region’s biodiversity but also increases the costs associated with long-term control and management. For example, the butt and root rot pathogen Heterobasidion annosum is considered one of the most destructive diseases to conifers in northern temperate regions with estimated annual losses to Europe’s timber industry standing at €800 million (Asiegbu et al. 2005).
Currently, the use of chemical pesticides has been the main protocol used in dealing with invasive species. However, many studies demonstrate that the indiscriminate and excessive use of synthetic pesticides poses serious risks to the environment through water contamination and soil contamination (Jallow et al. 2017) and has also resulted in the development of resistance among pests (Buch et al. 2013). The large-scale application of chemical agents can have an impact on non-target species (Chagnon et al. 2015). Woodcock et al. (2017) completed a study on the effect of neonicotinoid seed treatment on the environment and found that such treatment had a negative impact on the interannual reproductive potentials of both wild and managed bees, including honey bees, across certain countries. More pressingly, chemical pesticides have had a negative effect on human health (Lah 2011; Nicolopoulou-Stamati et al. 2016). For these reasons, Ireland and many other European countries have adopted Sustainable Forest Management (SFM) policies which include reducing pesticide use (Department of Agriculture 2013). Countries also seek certification from agencies such as the Forest Stewardship Council (FSC) to certify that forests are sustainably managed. It is under these certification bodies that agreements have been reached in relation to chemical usage (Karmann 2016).
Plant extracts are an attractive alternative as they appear to be biodegradable resulting in a reduced environmental impact (Cespedes et al. 2014; Bilal et al. 2017). This is because plant compounds have been known to break down rapidly into non-toxic residues that have reduced environmental longevity, hence, reducing their effect on the surrounding ecosystem (Grzywacz et al. 2014). Furthermore, plant biodiversity has provided a great source of biologically active compounds for use in crop protection (Singh 2014). Seaweeds demonstrate an effective innate defence system, with the pathogenic attack being a rare occurrence (Jimenez et al. 2011). It is speculated that this defence could be due to the production of selective active secondary metabolites, such as those that function as antimicrobial chemical defences (Peres et al. 2012). This activity has led to researchers paying more attention to seaweeds in the hope of exploiting these bioactive(s) as eco-friendly fungicides as demonstrated by (Asha et al. 2012). These secondary metabolites have also been found to exhibit a broad spectrum of other biological activities such as anticancer (Pádu et al. 2015), anti-inflammatory (Vázquez et al. 2011), etc. In addition, seaweeds have a long history of safe use in habitual diets (Brownlee et al. 2011, 2012) and as a source of medicines in the coastal regions (Allen and Hatfield 2004).
This review article focuses on the fungicidal activity illustrated by seaweeds and seaweed extracts against common plant pathogens and the test methods used to determine this activity both in vitro and in vivo. The purpose of this review article is to give readers access to information that has critically analysed the various test methods providing researchers with helpful tips and considerations so as to avoid the time-consuming process of searching for the most applicable method. This article also evaluates whether seaweed extracts are a viable source of potential novel eco-friendly bioactives for controlling fungal plant pathogens.
Collection and processing of seaweeds
The collection and processing of seaweeds are typically carried out following the same protocol which involves collecting the fresh seaweed at low tide times or Scuba diving for seaweeds located at lower depths. A specific time is selected as different seaweed species are found in different tidal conditions. For instance, Fucus vesiculosus, Fucus serratus, Ascophyllum nodosum, Kappaphycus alvarezii, Caulerpa scalpelliformis, Sargassum polycystum, Gracilaria arcuata, Hypnea pannosa, and Ulva spp. are found at low tidal zones (Hernández-Ledesma and Herrero 2014). Freshly collected seaweeds are then transferred back to the lab with each species being washed with distilled water to remove epiphytes and sand particles (Peres et al. 2012; Hernández-Ledesma and Herrero 2014; Sujatha et al. 2014). This ensures the compounds extracted are from the seaweed rather than the epiphytes and sand.
The next step is to prevent the microbial and enzymatic degradation of these samples during storage by the removal of water. This can be achieved through drying the seaweeds, mainly through shade drying (Barreto et al. 1997; Kausalya and Narasimha 2015), which can take a number of days for completion. Chanthini (2012) shade dried seaweed species for ten days at room temperature. However, this extended drying period may affect the biological activity of bioactives. Mei Ling et al. (2015) found that extended drying periods of three or four days affected the phytochemical content and antioxidant activity of the red alga, K. alvarezii. This was suspected to be as a result of a leaching effect. A number of studies have also dried seaweeds in an oven to ensure complete dryness and to speed up the process (Sujatha et al. 2014). This method is not without its drawbacks, as when high temperatures were employed, for example, 80 °C for 24 h for K. alvarerzii samples, the phytochemical content and antioxidant activity were negatively affected (Mei Ling et al. 2015). High oven temperatures were reported to readily deactivate polyphenol oxidases present in plant materials and also had the potential to lose many of the volatile compounds present which could be responsible for the bioactive effect (Ponmari et al. 2011).
Reports have suggested that freeze-drying (lyophilisation) is a superior drying approach with a comparative study completed by Cruces et al. (2015) finding that freeze-drying was the optimum method with regards extraction of phlorotannin yields and retention of antioxidant activity. However, freeze-drying is also said to have its disadvantages, mainly through the potential removal of volatile compounds under high vacuum (Shukla 2011). Though most studies report data generated from seaweeds that have been dried, some research has used fresh seaweed samples and, therefore, avoided potential degradation/loss of thermolabile or highly volatile bioactives. This was observed by Kolanjinathan (2009) where air-dried samples exhibited lower antimicrobial activity compared with fresh samples as a result of the loss of volatile compounds during the drying process. However, since these samples are fresh, testing must be completed in a relatively short time period to avoid microbial and enzymatic degradation, which may not always be possible. Therefore, there are a number of different drying techniques available where this becomes necessary, with the choice predominantly depending on the stability of the bioactive compounds targeted.
Seaweeds dried or fresh are generally blended to achieve a coarse powder in order to facilitate solvent diffusion into the cells as the smaller particle size increases the surface area of the seaweed exposed to the solvent and, hence, ensures optimum extraction of bioactives. Norra et al. (2016) reported that the smaller particle size of 0.25 mm gave higher total phenolic content compared with the larger particle size of 2.0 mm as a result of the enhanced surface area allowing for the greater mass transfer of solutes between phases. Some studies including Chanthini (2012) also sieved the blended seaweed through sieve plates to produce a standardised particle size which ensured more consistent extraction yields were obtained. Once the seaweeds are processed, they are generally stored under refrigerated conditions at 4 °C (Pandithurai et al. 2015; Ameer et al. 2016) or – 20 °C (Stein et al. 2011) to ensure the preservation of the samples until further analysis.
Extraction of antifungal bioactives
The desired antifungal components must be extracted and isolated from the seaweed using methodologies which optimise the recovery of the target bioactive compounds. There are a number of different extraction methods available but for the extraction to be efficient, it is important to choose a solvent that will penetrate the plant cell and will solubilise the desired compounds (Hernández-Ledesma and Herrero 2014). Hydrophilic compounds will be extracted by polar solvents such as water, methanol, ethanol, and acetone, whereas hydrophobic compounds will be extracted by non-polar solvents including hexane, chloroform, and dichloromethane. However, if the polarity of the antifungal bioactives is unknown, a number of different solvents with a range of polarities must be used. This broad spectrum solvent approach is a common method of choice, as the identity of the desired bioactive is typically unknown. A large number of studies have utilised a range of solvents including methanol, ethanol, chloroform, and hexane (Manivannan et al. 2011; Rajesh et al. 2011; Kausalya and Narasimha 2015). Manivannan et al. (2011) screened three brown seaweeds for antimicrobial activity and antifungal activities. The solvent system consisted of both polar (methanol) and non-polar (petroleum ether) solvents with all generated extracts exhibiting some degree of activity. This indicated that the desired bioactives present also ranged from polar to non-polar in character.
Solvent extraction typically involves the addition of dried (blended) seaweed into a specific ratio of solvent which is then stirred for a specific length of time (Sultana et al. 2008; Stein et al. 2011; Zhang et al. 2013; Sujatha et al. 2014; Pandithurai et al. 2015). The solvent is typically recovered by rotary evaporation at specific temperatures so as to yield dried extracts, as degradation of thermolabile bioactives is possible over an extended period of exposure to the extracting solvent (Joana Gil-Chávez et al. 2013). A number of studies that have utilised solvent extraction as their method of choice chose not to remove the solvent via rotary evaporation but instead left the extract as a concentrate in order to avoid the loss of volatile bioactives (Chanthini 2012; Ameer et al. 2016). Assessment of the antifungal potential of these newly generated extracts must be completed, however, within a short period of time so as to avoid the degradation of unstable bioactives. Solvent extraction has the advantages of being a cheap and simple method, but extraction over extended periods and/or at high temperatures can result in the degradation of unstable compounds and is also prone to high solvent usage (Zhang et al. 2018). Water extraction is considered the same methodology to solvent extraction with the water extracts reduced to dryness via evaporation (Stein et al. 2011; Kausalya and Narasimha 2015). However, as water has a relatively high boiling point, rotary evaporation can be a slow process; therefore, it is preferable to remove water through lyophilisation (freeze-drying) (Guedes et al. 2012; Kulshreshtha et al. 2016). Similar to solvent extraction, a number of studies have also chosen not to remove the water but instead the solid seaweed particles are removed through filtration or centrifugation so as to avoid the loss of volatile bioactives (Ambika and Sujatha 2015; Khan et al. 2017). Water extraction is commonly used for the extraction of ulvan, a water-soluble sulphated polysaccharide which is found in the intercellular space and in the fibrillar wall of green seaweeds mainly Ulva spp. (Pérez et al. 2016). This extraction of ulvan is of interest due to its reported antifungal properties suspected to be as result of its ability to act as elicitors on the plant’s defence response (de Freitas and Stadnik 2012; Araujo and Stadnik 2013). Water extraction exhibits all the same benefits as solvent extraction but has the added bonus of being a green extraction technique with most organic solvents being flammable, toxic, and requiring specialised disposal which can be quite costly (Chemat et al. 2012).
Soxhlet extraction is another conventional bioactive isolation technique and follows the principle of refluxing and siphoning to allow the bioactives to be continuously extracted with fresh solvents for a specific length of time followed by evaporation in order to remove the solvent (Aruna et al. 2010; Manivannan et al. 2011; Rajesh et al. 2011; Kausalya and Narasimha 2015). It is considered more efficient than conventional solvent extraction and generally requires less time and solvent (Zhang et al. 2018). Zakaria et al. (2011) reported that their Soxhlet extracts exhibited more profound radical scavenging activity compared with the extracts generated from solvent partitioning (liquid-liquid extraction). The main limitation of this technique and similar conventional methods is that it is not suitable for the extraction of thermolabile compounds as the sample is constantly heated (Sosa-Hernandez et al. 2018).
Maceration is another extraction technique that has been applied to seaweed systems and involves the maceration of powdered seaweed in a specific solvent mixture at room temperature (Rocha et al. 2011; Shobier et al. 2016). The solvent is then removed using rotary evaporation. However, this technique also suffers from the same limitations as solvent extraction.
Non-conventional extraction techniques are growing in popularity as a result of their green and efficient conditions. Ultrasound-assisted extraction (UAE) is a simple, relatively low-cost, efficient alternative to conventional techniques suitable for both small and large phytochemical extractions (Mandal et al. 2015). UAE employs ultrasound ranging from 20 to 2000 kHz which disrupts the plant cell wall releasing bioactive compounds, while the mass transport of the extracting solvent into the plant cells is also enhanced (Azwanida 2015). It is not widely applied to the extraction of bioactives from seaweeds but used instead for extraction of bioactives from natural compounds. Kadam et al. (2015) was the first study to investigate the use of UAE for the extraction of bioactives (total phenolics, fucose, and uronic acid) from the brown seaweed Ascophyllum nodosum. It was found that UAE was capable of extracting the highest yields of bioactive compounds in a relatively short time of 25 min, further illustrating the advantages offered by this novel technology. However, studies have reported a fall in yield when a longer extraction time was used. Melecchi et al. (2006), who investigated the effect of extraction parameters on the yield of Hibiscus tiliaceus, discovered that an extraction time above 300 min (5 h) resulted in a decrease in mass yield. This was suspected to be as a result of the decomposition of organic compounds by the sound waves. Garcia-Vaquero et al. (2018) also reported similar effects on the ferric reducing antioxidant power (FRAP) and 1,1-diphenyl-2-picryl-hydrazyl radical scavenging activity (DPPH) from extracts generated from the brown seaweed, Laminaria digitata. It was found that the optimum UAE conditions to preserve these antioxidant activities (FRAP and DPPH) were mild extraction conditions (temperature 40 °C, time 30 min, and amplitude 40%) with harsher conditions leading to a reduction in activity. This was suspected to be as a result of the loss of thermolabile compounds such as proteins and polyphenols. Additionally, the formation of free radicals above 20 kHz may have affected the active phytochemicals in both referenced studies (Azwanida 2015).
Other novel techniques including microwave-assisted extraction (MAE) and supercritical fluid extraction (SFE) can also offer advantages over conventional techniques including lower solvent consumption, shorter extraction time, and higher selectivity. Such approaches have become popular extraction options for natural products (Wrona et al. 2017; González de Peredo et al. 2018; Tyskiewicz et al. 2018), including seaweeds (Rodriguez-Jasso et al. 2011; Kim et al. 2012; McKennedy et al. 2016). MAE applies microwave radiation that causes the motion of polar compounds and the rotation of dipoles to heat solvents, and enhances the mass transfer of the desired compounds from the seaweed matrix into the liquid phase (Ying et al. 2011). The seaweed samples are typically mixed with the extracting solvent and the solution is irradiated over 2000 MHz for short periods of time (Ibañez et al. 2012). Despite the advantages MAE offers over conventional techniques such as lower solvent consumption, short extraction times, and homogeneous thermoregulation of the medium, there is very little literature on the isolation of bioactive compounds from marine sources (Ibañez et al. 2012). Li et al. (2012) reported that conventional techniques using a range of solvents resulted in extracts with less antioxidant activity and phenolic content from tomatoes compared with that from MAE. MAE has its shortcomings in that it is not suitable for the extraction of tannins and anthocyanins due to potential degradation at high temperatures (Azwanida 2015), and poor extraction efficiency is observed when either the target compounds or extracting solvent are non-polar or the viscosity of this solvent is extremely high (Wang and Weller 2006; Cravotto et al. 2008).
SFE is considered to be a “green” extraction technique and is based on the use of solvents, mainly carbon dioxide (CO2) at temperatures and pressure above its critical point (Ibañez et al. 2012). CO2 is cost-efficient and is an environmentally friendly solvent. De Corato et al. (2017) investigated the use of SFE for the isolation of antifungal compounds assessed against three fungal pathogens from brown and red seaweeds using the following conditions: compressed CO2; pressure = 37,900 kPa, density = 0.701 g mL-1, and temperature 50 °C. The generated extracts demonstrated antifungal activity and, hence, verified the potential of SFE as a bioactive extraction technique. The main drawback of SFE is that CO2 is non-polar and, therefore, not suitable for the extraction of polar compounds. This low polarity problem can be overcome by the introduction of co-solvents (methanol, ethanol) to change its polarity and in turn increase its solvating power. A major constraint with both MAE and SFE techniques is the initial high start-up cost of the equipment which may not always be justifiable for small companies.
In summary, before a reliable extraction technique is selected, one must consider the cost and time involved and, most critically, the properties of the desired bioactives. However, if these bioactives are unknown, as in many cases, a simple solvent extraction with short extraction times can be the solution to avoid the loss of unstable compounds as a result of temperature, pressure, and sonication.
In vitro assessment of antifungal activity from macroalgae
In order to evaluate the antifungal capacity of seaweed extracts, an effective test method must be utilised. Unfortunately, there is no single method that is suitable for screening extracts against fungal species. This has resulted in a variety of in vitro antifungal test methods being developed to determine the potential activity of seaweed extracts against plant pathogens with no uniform technique for comparison purposes. These in vitro studies are generally conducted in a Petri dish.
Disk diffusion
The disk diffusion assay, also referred to as the paper disk assay or agar diffusion method, involves impregnating 5–6 mm sterile paper disks with seaweed extracts at various concentrations (Manivannan et al. 2011; Machado et al. 2014b). The disks are allowed to dry before being placed onto a Petri dish that has previously been inoculated with the test fungi. Antifungal agents such as Amphotericin B serve as the positive control, and the respective solvent used to solvate the extract initially serves as the negative control (Saleh and Al-Mariri 2018). The plates are incubated under suitable growth conditions for the specific fungi and the zones of inhibition around each disk are measured (mm) after a set amount of time has elapsed. The disk diffusion assay is one of the most popular methods encountered in this review. This was not surprising since it is the official method used in the majority of clinical laboratories for routine antimicrobial susceptibility testing (Balouiri et al. 2016). Disk diffusion exhibits many advantages including low cost, flexibility, it allows visibility of growth with a high screening capacity, and also utilises low extract and reagent volumes (Coorevits et al. 2015). It also has one other advantage over another commonly used method known as well diffusion in that the solvating solvent is evaporated off the sterile paper disks prior to introduction to the inoculated Petri dishes, and therefore, the toxicity of the organic solvents towards the test fungi is avoided. The effectiveness of this method was illustrated by Chanthini (2012), who investigated the antifungal activity of six different seaweeds collected along the coast of India against the phytopathogen Alternaria solani, responsible for causing early blight disease in tomatoes. The seaweeds were extracted by liquid extraction using a range of solvents: methanol, ethyl acetate, and hexane. The antifungal activity was evaluated by disk diffusion assays, with the dried extracts re-dissolved in 5% dimethyl sulfoxide (DMSO) to give a final concentration of 50 μg of the particular seaweed extract which was then added to the sterile paper disks (Chanthini 2012). Of the six seaweeds tested, five produced biocidal activity against A. solani. The methanol and ethyl acetate extracts from the green seaweeds Ulva (Enteromorpha) flexuosa and Chaetomorpha antennia exhibited the most potent activity with 94 and 84% reduction of the fungi, respectively (Chanthini 2012). However, disk diffusion also has limitations such as diffusion problems, in that the polarity of natural compounds can affect the diffusion process of compounds into the culture medium, with the less polar the compounds the slower their diffusion. Hence, an extract exhibiting good antifungal activity but made up of non-polar compounds might exhibit a small zone of inhibition in a disk diffusion assay (Jiang 2011).
Well diffusion
The well diffusion method is also widely used to evaluate the antifungal activity of seaweed extracts (Rajesh et al. 2011; Shobier et al. 2016; Saleh and Al-Mariri 2018). The technique used is similar to that of disk diffusion in that the agar plate is inoculated with the test fungi before a hole is then punched 6–8 mm in diameter into the agar. The seaweed extract at a desired concentration is aseptically added to each well (20–100 μL) and allowed to diffuse (Vimala and Poonghuzhali 2017). Antifungal agents such as fluconazole can serve as the positive control, while the respective solvent used to solvate the extract serves as the negative control (Kausalya and Narasimha 2015). The agar plates are incubated under suitable growth conditions for the test fungi. The diffusion of the extract into the agar inhibits the growth of the test microorganism and produces a zone of inhibition around the well, which is measured in millimeters. One of the first studies on the fungicidal activity exhibited by seaweeds was conducted via the well diffusion assay, also known as the well-cut technique. In this method, the extract was added to wells before the plate was inoculated (Crasta et al. 1997) while all other studies followed the general procedure of inoculating the plates prior to extract introduction (Aruna et al. 2010; Kausalya and Narasimha 2015; Shobier et al. 2016). However, regardless of the procedure followed, well diffusion proved to be a highly effective method. For example, Rajesh et al. (2011) focused their study on one seaweed, the green seaweed Caulerpa scalpelliformis, against the fungal plant pathogen Fusarium oxysporum. The seaweed was extracted in three different solvents, hexane, chloroform, and methanol, using the Soxhlet apparatus with agar well diffusion assays employed as the method of determining antifungal activity. The concentration of extracts was also varied from 0.05 to 0.8% and was prepared using dimethyl sulphoxide (DMSO). Plates containing DMSO served as the negative control, while carbendazium served as the positive control. The plates were incubated at 27 °C for three days. The zone of inhibition was then measured with methanol exhibiting inhibition zones of 12.66 ± 0.33 mm, hexane 11.33 ± 0.33 mm, and chloroform 10.33 ± 0.33 mm.
This study and the others referenced above illustrate the effectiveness of well diffusion as an antifungal test method, with some added advantages over certain methods. These include the fact that it does not require as much extract compared with the poisoned food technique (described later) which requires 25 mg of dried extract when incorporated into 5 mL of agar to give the desired concentration of 5 mg mL−1 while the same dose for the well diffusion assay requires only 5 mg of dried extract dissolved in 50 μL of the solvating solvent. Also, in well diffusion triplicates and both controls are carried out on the same Petri dish which reduces the amount of media needed. In addition to this, well diffusion (similar to disk diffusion) is considered a simple, low cost, rapid method of screening, with a high-throughput sample capacity (Hernández-Ledesma and Herrero 2014). However, as with all methods, it has its limitations specifically around diffusion issues, with the polarity of the solvating solvent affecting the ability of compounds to diffuse through the polar culture medium (Hernández-Ledesma and Herrero 2014). For example, non-polar solvents could potentially diffuse slower through the agar, meaning such non-polar extracts with good antifungal activity might exhibit a small zone of inhibition in the well diffusion assay. For these reasons, the use of polar solvents such as water is considered optimum. However, from examination of the studies that employed the well diffusion method, some utilised non-polar solvents including Kausalya and Narasimha (2015) who found that chloroform extracts of Sargassum polycystum at 100 mg mL−1 exhibited relatively strong antifungal activity against Aspergillus niger (16 mm) and Rhizoctonia solani (18 mm). The methanol extracts of S. polycystum at the same concentration achieved slightly higher activity with a 19-mm inhibition recorded for both A. niger and R. solani. Similarly, organic solvents can be toxic to the test fungi. However, all of these studies included a negative control ensuring the organic solvents did not negatively affect the growth of the fungi (Balouiri et al. 2016).
Poisoned food technique
The poisoned food technique is commonly used to determine the antifungal potential of seaweed extracts (Ameer et al. 2016). In the work by Sujatha et al. (2014), the seaweed extract was incorporated into liquid media to produce a desired final concentration before being allowed to solidify in a Petri dish. The plate was inoculated with a 5-mm disk of an actively growing fungal culture which was deposited in the centre of the plate. The medium without extract served as the control. The plates were incubated under suitable growth conditions for the test fungus. After a specific amount of time, the radial growth of mycelium was measured and compared with that of the control in order to determine the mean inhibition percentage (Sujatha et al. 2014). One of the first studies to illustrate the antifungal potential of seaweed extracts was conducted by Barreto et al. (1997) who investigated the effects of ethanol extracts of selected South African seaweeds against the plant pathogens, Rhizoctonia solani and Verticillium sp. The pour plate method, a variation of the poisoned food technique, was used to determine the activity of the extracts previously extracted into ethanol by liquid extraction. This work involved the incorporation of the extracts into molten agar at a desired final concentration, followed by inoculation with a plug of either R. solani or Verticillium sp. Extracts from Caulerpa filiformis, Ulva rigida, Zonaria tournefortii, Hypnea spicifera, Gelidium abottiorum, and Osmundaria serrata all inhibited the growth of both fungi by more than 50%. However, the effect of fungal growth was found to be concentration dependent, with the lower concentrations (1:16 and 1:32 dilutions) of H. spicifera exhibiting growth promotion of R. solani (Barreto et al. 1997). The poisoned food technique is a popular method of choice (Coşoveanu et al. 2010; Ameer et al. 2016; Esserti et al. 2016; De Corato et al. 2017; Khan et al. 2017) which is not surprising as it is widely used to investigate the antifungal capacity of natural products against moulds (Kumar et al. 2013; Salhi et al. 2017). This technique is classified as an agar dilution method which is considered relatively quick and easy and does not involve the use of complicated equipment (Panda 2012).
Sujatha et al. (2014) investigated two root rot and seedling rot causing fungi R. solani and M. phaseolina. A seaweed from each species was evaluated, including the green algae Caulerpa racemosa, brown algae Sargassum myricocystum, and the red algae Gracilaria edulis, all collected from beaches in India. The seaweed underwent liquid extraction in alcohol and was re-dissolved in water to give 10, 20, and 30% concentrations. The poisoned food technique was applied to determine the antifungal activity, with the colony diameter measured after 48, 72, and 96 h incubation at 25 °C. It was found that a 30% concentration of S. myricocystum after 96 h incubation was able to reduce the mycelial growth of both R. solani and M. phaseolina by 61.44 and 58.53%, respectively. These studies demonstrate the ability of the poisoned food technique to be applied as an effective method for the determination of antifungal activity of crude seaweed extracts. This method suffers from a number of drawbacks including the use of larger volumes of extract compared with other methods. For example, 25 mg of dried extract dissolved in 5 mL of molten agar will achieve a 5 mg mL−1 concentration, whereas the same concentration can be achieved using 5 mg of dried extract in disk diffusion. Additionally, hydrophobic extracts can separate out in the agar leading to false negative results, volatile compounds present in the extracts can potentially affect the growth of the fungi, and finally, the size of inoculum and incubation conditions can affect the rate of growth, hence, percentage inhibition observed (Panda 2012).
Dry weight analysis
Dry weight analysis is not as widely used as the other methods mentioned above. In the work by Galal et al. (2011), the sterilised media was inoculated with a fungal disk that had been taken from a five-day-old colony. The flask was supplemented with seaweed extract and incubated under suitable growth conditions for the test fungi. An antibiotic agent such as Nystatin served as the positive control, while the respective solvent used to solvate the extract served as the negative control. The mycelial growth of fungi was filtered through a pre-weighed filter paper. The agar inoculum was removed from the mycelium which was allowed to dry in an oven at 85 °C for 24 h before the dry weight was obtained and compared with that of the controls. Galal et al. (2011) used mycelial dry weight analysis as the means to determine the antifungal capacity of methanol and ethyl acetate crude extracts of seaweeds collected from the Red Sea against pathogenic fungi. This method was used in early studies to monitor the growth of fungi (Sutton and Starzyk 1972; Opgenorth and Endo 1983; Yamada et al. 1992). However, this method has been widely replaced with spectrophotometric techniques such as broth dilution assays (Puglisi et al. 2004; Stein et al. 2011). This method proved effective in this study, with the brown seaweed Padina gymnospora found to be the most potent. Dry weights for all of the tested fungi showed complete inhibition, except against Alternaria brassicicola. This is very much a standalone method in that it has no interlinking features with diffusion methods, bioautography methods, or dilution methods. Mycelial dry weight analysis is considered a low-cost, accurate method that does not require elaborate equipment. However, it also has its drawbacks in that it is a tedious and time-consuming process, making it unsuitable for large screening volumes.
Broth dilution assay
Broth dilution is considered one of the most basic antimicrobial susceptibility testing procedures. In the work by Balouiri et al. (2016), the seaweed extracts were prepared to their desired concentration using liquid growth medium as the diluent and added to tubes containing 2 mL or above for macro-dilution or with smaller volumes for micro-dilution. Each tube was inoculated with the test fungi that had been adjusted to 0.5 McFarland units. The solutions were mixed and the tubes incubated under suitable growth conditions for the fungus. An inoculated tube consisting of medium and the solvating solvent served as the negative control and an antimicrobial agent, such as Fluconazole, served as the positive control (Stein et al. 2011). Spectrophotometric readings were taken of each tube and compared with that of the negative control. The minimum inhibitory concentration is generally recorded as the lowest concentration of seaweed extract that fully inhibits the growth of the test fungi in the tubes. The broth dilution method has been standardised by the National Committee for Clinical Laboratory Standards (NCCLS) and the European Committee on Antimicrobial Susceptibility Testing (EUCAST) (Balouiri et al. 2016). Dilution methods were employed in two studies (Puglisi et al. 2004; Stein et al. 2011). Stein et al. (2011) screened for antifungal activity by the Brazilian red seaweed genus Laurencia against three strains of the fungal pathogen: Candida albicans, Candida parapsilosis, and Cryptococcus neoformans. The seaweeds were extracted in a number of different solvents including hexane, methanol, chloroform, and water. The extracts were re-dissolved in 10% ethanol to give a final concentration of 4 mg mL−1. Seaweed was also extracted in water and concentrated on a Speed Vac to produce solvent-free extract. The broth micro-dilution technique (96-well plates) was used to determine the effect of the extracts on the growth of the above three pathogenic fungi with the concentration of inoculum between 5.0 × 102 and 2.5 × 103 CFU mL−1. From the results, it was found that the apolar extracts of several species of Laurencia exhibited interesting antifungal properties with the chloroform extracts of Laurencia intricata being the most active with a minimum inhibitory concentration higher than 220 μg mL−1. This study illustrates the effectiveness of the broth dilution method not only in identifying the antifungal activity of seaweed extracts but also in determining MIC. This is a major limitation for certain methods such as the poisoned food technique where MICs cannot be accurately defined since it is impossible to quantify the amount of extract that has diffused into the media (Balouiri et al. 2016). Broth dilution is also highly reproducible and low cost because of the low volume of reagents and space required, making it suitable for large sample throughput. However, broth dilution has some technical constraints such as it is tedious, time-consuming, and technically demanding with poor end-point precision, in particular when the fungistatic agents namely azole and five-FC are tested (Kumar et al. 2010).
Direct bioautography
This is one of the simplest methods for the detection of antifungal compounds and also exhibits reproducible results for spore-producing fungi including Aspergillus, Cladosporium, and Penicillium (Balouiri et al. 2016). Rocha et al. (2011) selected direct bioautography to investigate the potential of new antifungal agents present in the red seaweed, Centroceras clavulatum. The seaweed extracts were dissolved in polarity-compatible solvents and applied to a pre-coated TLC plate and developed in a specific mobile phase. After separation, the plates were allowed to dry in order to remove all solvent residues. The TLC plates were sprayed with a microbial suspension of the test fungi in a salt and glucose solution which aided in the visualisation of microbial growth. The plates were incubated under suitable conditions with a clear zone of inhibition appearing against the dark background. Nystatin was used as the standard control. A number of other studies have employed thin-layer chromatography (TLC)-bioautography, specifically direct bioautography (Barreto and Meyer 2006; De Felicio et al. 2010; Rocha et al. 2011). For instance, Barreto and Meyer (2006) investigated the antifungal activity of lanosol ethyl ether (LEE), a biologically active compound from the red seaweed Osmundaria serrata against Alternaria alternata (plant pathogen) and Candida albicans (human pathogen). The bioactive was first extracted using liquid extraction in ethanol before the material was re-extracted in ethanol/ethyl acetate (1:1, v/v) and again in ethyl acetate. All extracts were combined and purified by column chromatography with the activity of each band assessed by bioautography. The results demonstrated that lanosol ethyl ether (LEE) is more effective in slowing growth as opposed to killing the fungus, since the mean fungicidal (causes fungal death) concentration was 0.69 ± 0.15 mg mL−1 compared with the fungistatic value (inhibits the growth of the fungus) of 0.27 ± 0.07 mg mL−1. This demonstrates the high fungistatic activity of LEE from O. serrata against both plant and human pathogens, indicating a broad range of activity from this bioactive. Direct bioautography offers a rapid technique for the screening of a large volume of samples for bioactivity with the use of minimum equipment. It also has the added bonus of achieving final clean-up of fractions in order to obtain pure compounds, since the bioactive(s) separate out based on their relative affinities for the stationary phase (TLC plate) and mobile phase (Dewanjee et al. 2015; Balouiri et al. 2016). Additionally, bioautography can be both qualitative and quantitative with Machado et al. (2014a) completing qualitative analysis on their crude Ochtodes secundiramea extracts against the two plant pathogens, Cladosporium sphaerospermum and Colletotrichum lagenarium. They also conducted quantitative analysis on purified compounds by applying known doses of extract to the TLC plate with a detection limit of 5 μg determined for both pathogens, i.e., minimum inhibitory concentration. A limitation with bioautography is that a number of different mobile phase compositions may need to be investigated in order to obtain the optimum separation which can be a time-consuming process and wasteful.
Plate stripping method
The plate stripping method is a similar procedure to the poisoned food technique, except in this case the extract is not incorporated into the media. The seaweed extracts were initially dissolved in their respective solvents and four blotting paper strips were then dipped into this solution (Kabir Khanzada et al. 2007). The solvent was then allowed to evaporate off in a vacuum chamber. Mycelial disks, 5 mm in diameter, were obtained from an actively growing fungal culture and aseptically transferred onto fresh agar. These impregnated strips were then placed around the mycelial disk of the test fungi while sterilised strips served as the control. The colony diameter was recorded after 72 h of incubation and compared with that of the control plate in order to calculate percentage inhibition. Kabir Khanzada et al. (2007) used the strip plating method to evaluate the antifungal activity of the red seaweed Solieria robusta against five fruit spoiling fungi. The seaweed was extracted in 100% ethanol and reduced to a viscous residue before being fractionated into ethanol, ethyl acetate, chloroform, methanol, and aqueous fractions. This was the only study that employed this test method, with concentrations of each fraction ranging from 20 to 0.02 mg mL−1. All five solvent fractions were found to be active against at least one of the fruit spoiling fungi, with the aqueous fractions demonstrating a 99% inhibition of growth against A. niger at 20 mg mL−1. This method is similar to the Etest which is a commercially available method for antimicrobial susceptibility testing (Rex et al. 2001). However, in the Etest method, a calibrated strip impregnated with a gradient of antimicrobial concentration is used and is placed on a lawn of microbial growth. The MICs are determined from the point of intersection of a growth inhibition zone around this calibrated strip (Rex et al. 2001). Kabir Khanzada et al. (2007) employed four blotting strips dipped in 1 mL of extract and the solvent was allowed to evaporate off before being placed around a plug of fungi, and the % inhibition was determined after 72 h of incubation. Since this method has a similar methodology to that of disk diffusion, it shares many of the same advantages namely low cost and simplicity, and since the solvent is evaporated off prior to introduction, the impact of organic solvents on the growth of the fungi is avoided (Balouiri et al. 2016). However, as with disk diffusion, it also suffers from diffusion issues and the fact that volatile bioactives may evaporate off during this drying process.
Spore streaking method
The spore streaking method is similar to disk diffusion except that the Petri dishes are inoculated with a non-adjusted spore suspension. Zhang et al. (2013), applied this specific test method with the suspension obtained from a ten-day-old culture that was harvested by scraping a sterile blade over the surface of this mycelia culture containing 15 mL of sterile water. The crude suspension was filtered through three layers of sterile gauze in order to achieve a pure spore suspension. A total of 100 μL of this suspension was then spread over the surface of a plate. Sterile paper disks were impregnated with seaweed extracts and the solvating solvents were allowed to evaporate off to give the desired final concentration. Sterile paper disks served as the control. The plates were incubated under suitable growth conditions for the test fungi and the zones of inhibition measured (mm) after 24–48 h. This approach, therefore, investigated the ability of the seaweed extracts to inhibit the spore germination of P. oryzae. Positive results were observed for this spore plate method with Symphyocladia latiuscula and Rhodomela confervoides exhibiting zones of inhibition of 35 mm and 20 mm, respectively (Zhang et al. 2013). This method is very similar to disk diffusion with the only difference being that the fungi are adjusted in disk diffusion and, therefore, shares the same advantages and limitations of disk diffusion namely low cost, rapid analysis, and avoidance of organic solvents. However, this method suffers from the potential loss of volatile compounds during the drying process and potential diffusion issues surrounding non-polar compounds (Balouiri et al. 2016).
Plate conformation method
The plate conformation method is also a hybrid of the disk diffusion method. Zhang et al. (2013) used a plate inoculated in the centre with a mycelia disk of the test fungi and then incubated the plate under suitable conditions until the colony reached 1 cm in diameter. Paper disks impregnated with seaweed extracts were placed 1 cm from the edge of the colony with sterile paper disks serving as the control. The plate was incubated again for 24–48 h and the inhibition of mycelium growth was recorded. Zhang et al. (2013) also applied this antifungal test method to investigate the antifungal potential of eight seaweeds found along the Yellow Sea against the rice pathogen, Pyricularia oryzae. However, it was found that all eight seaweeds exhibited no inhibitory activity, meaning the crude extracts did not inhibit the growth of P. oryzae, whereas the spore streaking method demonstrated the ability of the seaweed extracts to inhibit the spore germination of P. oryzae. The plate conformation method has similar characteristics to the poisoned food technique and the strip plate method in that the plate is inoculated with a plug of fungi with the % inhibition determined, and therefore, it has the advantage of not requiring adjustment of fungi. It also has characteristics of the disk diffusion assay and, therefore, requires a low volume of extract and has the added bonus of removing organic solvent prior to contact with the test fungi, against which it may exhibit a toxic effect (Balouiri et al. 2016). Consequently, since this is a diffusion method, it has the same limitations exhibited by such methods namely diffusion issues associated with non-polar compounds being unable to diffuse through the polar culture medium and the loss of volatile compounds during the drying process (Balouiri et al. 2016). Nonetheless, the plate conformation method is a simple, low-cost, rapid test method for determining the fungicidal activity of crude seaweed extracts.
All of the studies reviewed above demonstrate the potential of seaweed extracts in controlling fungal plant pathogens, potentially reducing the usage of current synthetic fungicides. Table 1 summarises the advantages and disadvantages associated with each test method. For example, the most commonly used test method is disk diffusion due to the low volume of extract required and the ease of interpretation of results making it the method of choice generally. However, disk diffusion is not always suitable particularly with regards to fungi that do not form an even lawn of growth or take a number of days to achieve this, as by then the potential bioactive compounds could breakdown leading to false negative results. A number of other of methods including the poisoned food technique can overcome this problem and, therefore, factors to consider when deciding on the most appropriate antifungal test method to be used include extract solubility properties, fungal species, cost, and labor. In order to ensure the use of seaweeds as a promising alternative in vivo studies must also be conducted in order to fully establish the efficacy of the seaweeds. The “In vivo studies on the antifungal activity of macroalgae” section details some of the commonly used in vivo antifungal assays.
In vivo studies on the antifungal activity of macroalgae
In vivo studies are generally conducted on a whole plant to determine the effectiveness of the seaweed in “real life” and also evaluates the toxicity of the seaweed to the plant itself or other non-target species, if any exists. For this reason, a vast number of studies conduct an initial in vitro screen followed by an in vivo study (Abouraïcha et al. 2016; Esserti et al. 2016; Ramkissoon et al. 2017; Mani and Nagarathnam 2018). For instance, Ibraheem et al. (2017) investigated the antifungal potential of three brown seaweeds against soil-borne plant pathogens through the in vitro disk diffusion assay. The methanolic extracts of Padina gymnospora and Sargassum latifolium were found to exhibit strong activity; however, Hydroclathrus clathratus extracts demonstrated no degree of antifungal activity (Ibraheem et al. 2017). This was followed by in vivo application of the powders of P. gymnospora and S. latifolium and H. clathratus as a soil conditioner for Solanrum melongena (eggplant) in a pot experiment. It was found that the seaweeds significantly decreased the percentage of root rot caused by Fusarium solani by 83, 56, and 27%, respectively.
The majority of in vivo studies investigate the possible elicitor activity of seaweeds. Elicitors are a class of molecules that can enhance the disease resistance of a host. The effect of elicitors is regulated by signalling pathways such as salicylic acid, jasmonic acid, and ethylene pathways, and can induce local and systemic defence responses in plants (Ramkissoon et al. 2017). There are numerous reports of the enhanced resistance demonstrated by crops against pathogens following treatment with commercial elicitors such as benzo(1,2,3)-thiadiazole-7-carbothioic acid-S-methyl ester (Thakur and Sohal 2013). Seaweeds contain a large quantity of polysaccharides which are believed to function as elicitors for plant defence responses against microbial pathogens (Abouraïcha et al. 2015). El Modafar et al. (2012) reported the bioelicitor activity of ulvan and oligoulvans isolated from the green seaweed, U. lactuca, in stimulating both a natural systemic defence and a systemic acquired resistance in tomato seedlings against Fusarium oxysporum f. sp. lycopersici.
Fruit decay evaluation
This method investigates the ability of seaweeds to control fungal infections in fruit mainly apples (Abouraïcha et al. 2015, 2016). Abouraïcha et al. (2015) evaluated the natural defence and protectant activity in apple fruit against Botrytis cinerea through the introduction of two saccharides, ulvan and oligoulvans, isolated from the green seaweed, U. lactuca, through fruit decay evaluation. The apples were wounded at two locations and then treated with the seaweed extract. After 12 h of treatment, the wound sites were inoculated with spore suspensions of the test fungi. The inoculated fruits were incubated in a plastic bag in order to maintain a relative humidity of 95% for six days. Disease incidence and lesion diameter on each wound site were recorded after two, four, and six days (Abouraïcha et al. 2015). Once the visible rot zone became wider than 1 mm, the apple was regarded as a decayed fruit with the decay incidence (%) recorded.
This method differed from the spray method discussed below in that the protectant activity of seaweed compounds were tested on pieces of fruit. It was found that the seaweed-treated apples exhibited a 30% lower decay incidence compared with the control fruit. The oligoulvan treated apples demonstrated no decay incidences, meaning oligoulvan resulted in 100% inhibition against B. cinerea (Abouraïcha et al. 2015). This method has the advantage of being a low-cost, simple, rapid method with the added bonus of testing “real” samples. However, the method is not very quantitative with the interpretation of results between researchers.
Foliar spray treatment
In this technique, a liquid solution of seaweed extract is sprayed onto the aerial parts of a plant (Jayaraj et al. 2008; Jayaraman et al. 2011; Hernández-Herrera et al. 2014; Abkhoo and Sabbagh 2015; Esserti et al. 2016; Mani and Nagarathnam 2018) or applied on leaves obtained from a susceptible host in a Petri dish (Roberti et al. 2016) in order to protect against fungal diseases. Ramkissoon et al. (2017) investigated the phytoelicitor activity of three Caribbean seaweed species on the suppression of Alternaria solani in tomato plants via a foliar application (Ramkissoon et al. 2017). Healthy tomato seedlings were planted in peat moss and garden soil and fertilised every five days. After 25 days, the plants were sprayed until run-off with aqueous seaweed extract solution at a 0.5% concentration with treatments repeated twice at 15-day intervals (Ramkissoon et al. 2017). Kocide the copper-based fungicide served as the control. After treatment, the tomato plant leaves were slightly wounded and inoculated with a conidial suspension of A. solani. The pathogen suspension was sprayed onto the plants until run-off (drenched), and they were incubated in a humid chamber for 48 h to initiate infection before being returned to the greenhouse. Disease severity was assessed using a six-point disease rating scale and was based on the percentage of leaf area infected, i.e., 1 = 0%, 2 = 1–10%, 3 = 11–25%, 4 = 26–50%, 5 = 41–55%, and 6 = > 56% with the percent disease index (PDI) calculated using the following equation:
A disease severity scale was applied by a number of other studies to allow for numerical interpretation of results (Hernández-Herrera et al. 2014; Abkhoo and Sabbagh 2015). These studies also demonstrated the antifungal activity of seaweeds against fungal pathogens with Ramkissoon et al. (2017) reporting that all three seaweeds investigated limited the infection of A. solani to varying degrees in tomato plants. This antifungal activity was suspected to be a result of elicitor activity since a reduction in disease severity was coupled with increased levels of defence enzymes and expression levels of marker genes for defence signalling pathways. This method is low cost and, since the extract is tested on a whole plant, it determines possible toxicity towards the host plant at an early stage. However, similar to the fruit decay assay, interpretation of results may vary between researchers and can require large quantities of dried seaweeds in order to obtain a sufficient volume of seaweed extract. Additionally, since the seaweeds are applied as a spray, the solvent used must be safe to the plant but also capable of extracting the required bioactives. For this reason, the majority of studies apply water as the solvating solvent for foliar applications (Esserti et al. 2016; Ramkissoon et al. 2017) with some adding Tween 20 in order to improve absorption by the plants of the seaweed extract solution (Hernández-Herrera et al. 2014; Mani and Nagarathnam 2018).
Screen-house study
In the screen-house experiment, dry powdered seaweed was mixed with sandy loam soil (pH 8.1) to give the desired final concentration (Ehteshamul-Haque et al. 2013). The seaweed-treated soil was transferred to plastic pots and maintained at a 50% water-holding capacity. The soil was naturally infested with the test fungi at a known concentration. Four seeds that were susceptible to infection from the test fungi were planted in each pot, with pots containing no seaweed serving as the control. The plants were uprooted six weeks later in order to assess the efficacy of the seaweed treatment (Ehteshamul-Haque et al. 2013). A 1-cm long root piece was removed from tap roots and the surface disinfested with 1% Ca(OCl)2 (calcium hypochlorite) and plated onto media that can sustain the growth of the test fungi. The plates were incubated under suitable growth conditions with results recorded after five days and compared with that of the control plate. The screen-house study is the most popular method of choice in determining the antifungal activity of seaweeds against soil-borne fungi (Sultana et al. 2005), unlike spray treatment which evaluates the activity of seaweeds in protecting the aerial parts of the plant against pathogens such as A. solani which generally enters through wounded leaves (Ramkissoon et al. 2017).
The majority of screen-house studies apply a combination of biocontrols such as Pseudomonas fluorescens, chemical fertilisers such as urea, and finally chemical pesticides such as Topsin-M, either all three together or alone (Sultana et al. 2005, 2008, 2009, 2011a, b; Jayaraj et al. 2008; Uppal et al. 2008; Ehteshamul-Haque et al. 2013). For instance, Sultana et al. (2011b) investigated the fungicidal potential of the red algae Solieria robusta against three common fungal pathogens Macrophomina phaseolina, Rhizoctonia solani, and Fusarium solani either alone or in combination with chemical fertilisers and/or pesticides on soybeans. The pesticide used was Topsin-M and urea and potash served as the chemical fertilisers. Pots without fertiliser and seaweed served as the negative control while Topsin-M served as the positive control. The plants were uprooted six weeks later in order to determine the efficacy of the seaweed, fertilisers, and pesticide against the root pathogens, and to assess their effect on plant growth. The results from the study demonstrated that soil with S. robusta, fertilisers or pesticides alone caused significant reduction of M. phaseolina and R. solani, with a percentage of infection of only 37.5 and 18.7%, respectively, when S. robusta was used alone (Sultana et al. 2011b). A mixed application of S. robusta with potash or both potash and urea led to F. solani being completely suppressed. However, the highest reduction of F. solani, by 68.8% was achieved by S. robusta alone. The use of Topsin-M alone or in combination with S. robusta was found to significantly increase the number of flowers as well as greater shoot length. Overall, it was found that the seaweed S. robusta was not only as effective as the two chemical fertilisers in enhancing plant growth but had more or less equivalent effects in suppressing the fungal pathogens as the pesticide Topsin-M. Two hypotheses for this suppression was that the seaweed served as food for antagonistic bacteria or that the seaweed contained bioactives that were capable of elicitor activity (Sultana et al. 2011b). This study successfully illustrated the antifungal potential of seaweeds either alone or in combination on “real” samples. The screen-house study, therefore, has the advantage of testing a seaweeds activity as a powder and, hence, avoids the time-consuming process of extractions with solvents and also allows it to be combined with both fertilisers and chemical fungicides. However, the disadvantages associated with this method are that it requires a large amount of dried seaweed and the method is only applicable to fungi that are soil-borne pathogens.
Sand solution technique
The sand solution technique is a method used to determine clubroot infection caused by the obligate soil-borne parasite Plasmodiophora brassicae in brassica crops including broccoli, cabbage, and the oilseed plant canola. In Australia, seaweed extracts have been applied as part of a management strategy against clubroot (Wite et al. 2015), but few studies have been reported on this specific control strategy (Stewart 2008). Wite et al. (2015) applied this method to evaluate the ability of commercial seaweed extracts in suppressing P. brassicae in broccoli. In this technique, acid-washed, coarse sand was sterilised via autoclaving (121 °C/100 kPa) and loosely packed into tapered pipette tubes. Broccoli seeds were surface sterilised in 70% ethanol and 10% sodium hypochlorite solution for 3 min, and were rinsed in triplicate in sterile distilled water (Wite et al. 2015). The seeds were allowed to dry under sterile conditions. A single seed was then sown in each of the sand-packed pipette tubes. The tubes were placed in a buffered nutrient solution to avoid nutrient stress to the broccoli and maximising P. brassicae infection. After five days, 2 mL of the seaweed extract which had been previously sterilised through UV exposure was applied to the sand (Wite et al. 2015). The commercial seaweed extract solutions were an alkaline hydrolysis product from the seaweeds Durvillaea potatorum and Ascophyllum nodosum (Seasol Commercial®) and also contained low concentration of nutrients such as laminarins, auxins, and cytokinins. The seaweed extracts were applied at 1:25 and 1:200 dilutions with sterile distilled water serving as the control. After 28 days, the plants were inoculated with 0.2 mL spore suspension of P. brassicae into the sand (Wite et al. 2015). The degree of infection was assessed on days 14, 28, and 45 after inoculation. On each assessment day, the roots of six plants per treatment were removed and rinsed free of sand. The roots were fixed with formyl acetic acid and stained with Phloxine B in order to visually observe the development of P. brassicae infection. Twenty root sections per plant also underwent a microscopic examination under a compound microscope to determine the number of plasmodia in the root hairs and cortical cells. It was found that the seaweed extracts reduced the number of plasmodia in the root hairs in the initial stages of infection by 55%, and in the secondary stages of infection, this increased to 84% in the cortical cells (Wite et al. 2015). These results demonstrate the effectiveness of this established technique in quantifying the suppressive effect of seaweed extracts on infection of brassica crops by P. brassicae. As with similar in vivo methods, such antifungal activity was suspected to be as a result of the stimulation of resistance mechanism (elicitor activity) in the host possible related to the presence of laminarins in the extract (Wite et al. 2015). This method is a similar methodology to the screen-house study and therefore only suited to fungi that are soil-borne pathogens. This approach has the added advantage of being conducted in 5-mL tubes and therefore requires less seaweed extract.
Such in vivo studies further confirm the potential of seaweeds to be applied as an effective biofungicide. It also demonstrates the wide variety of in vivo assays designed to evaluate this elicitor activity possessed by seaweeds. However, as with in vitro tests, there is no accurate method for quantification with the selection heavily based on the route of infection of the pathogen. As a result, soil-borne pathogens are best suited to the screen-house study whereas pathogens that effect fruit directly would be more suited to fruit decay evaluation. Table 2 summarises the test methods plus their associated advantages and disadvantages.
Statistical analysis
All of the reviewed methods involved work being carried out in at least duplicate (Kausalya and Narasimha 2015) with the majority being conducted in triplicate and results presented as the mean ± standard deviation (Stein et al. 2011; Machado et al. 2014b; Abouraïcha et al. 2015; Pandithurai et al. 2015). A number of studies employed statistical software such as the one-way ANOVA using a 5% statistical significant level (p < 0.05) in order to determine whether there was a statistical difference between seaweeds, solvents, seasons, geographical locations, etc. with regards to antifungal activity (Machado et al. 2014b; Abouraïcha et al. 2015; De Corato et al. 2017).
Current prospects and challenges of biopesticides
The advantages of using naturally derived pesticides have been well documented and include low toxicity compared with conventional pesticides, and are biodegradable, which minimises exposure to human and non-target species (Ivase et al. 2017). Therefore, there is great interest in the phasing out/reducing of toxic synthetic pesticides by extensive research into new molecules which are non-synthetic and biodegradable with reduced toxicity. This is further encouraged by the negative environmental and health effects associated with conventional pesticides leading to stringent pesticide regulations and pressure from organic producers (Chanthini 2012). Public awareness about the adverse effects of pesticides on food safety and the environment has become more prominent in recent years, leading to research for safer alternatives including biofungicides (Damalas and Koutroubas 2018).
The studies reported in this review illustrates the strong potential of seaweeds to be used as an eco-friendly fungicide. For instance, in the screen-house studies, seaweeds used in combination with a biocontrol agent and/or conventional pesticides were extremely effective in controlling disease and at the same time aided in reducing the use of chemical controls. In addition to this, seaweeds have been shown not only to exhibit fungicidal activity but also other pesticidal activities such as insecticidal. The application of seaweeds as an insecticide has recently become a popular concept, especially due to their non-toxic nature when used in agricultural situations. A number of studies on the insecticidal potential of seaweeds have been carried out (Ravikumar et al. 2011; Bianco et al. 2013; Pasdaran et al. 2016). For instance, Abbassy et al. (2014) evaluated both the fungicidal and insecticidal activities of the green seaweed, U. lactuca. Petroleum ether and methanol extracts were found to exhibit potent insecticidal activity with the methanol extracts also found to exhibit strong antifungal activity against three phytopathogenic fungi.
In spite of their positive prospects, the biopesticide market has a relatively small market share accounting for only 2.5% of the total world pesticide market (Balog et al. 2017). In the United States (USA) market, more than 200 biopesticide products are available compared with only 60 analogous products in the European Union (EU) (Damalas and Koutroubas 2018). This is as result of the EU applying the same regulations when assessing synthetic active substances requiring new provisions in the current legislation and preparation of new registration guidelines which in turn increases the complexity of EU-based biopesticide regulations (Balog et al. 2017). Additionally, biopesticides have been reported to exhibit a short shelf life and field persistence leading to repetitive applications required for the effective eradication of a pest (Ivase et al. 2017). This increases the costs of using biopesticides as an integrated pest management strategy. Additionally, the specificity exhibited by biopesticides is considered an advantage in reducing toxicity to non-target species, but on the contrary, it typically narrows the range of activity such as the fungus Verticillium lecanii against cereal aphids meaning one may require the use of a number of different biopesticide products or in conjunction with conventional agrochemicals (Villaverde et al. 2014).
Future work
Detection of the antifungal potential from seaweeds is not enough to meet the demands of the global pesticide market without these novel antifungal compounds undergoing industrial scale up. In order to achieve this, a sufficient amount of such bioactive compounds must be extracted which can be extremely difficult due to the low quantities produced by the plant itself with activity depending on biotic and abiotic factors (Saha et al. 2018). Consequently, a vast amount of seaweed would be required with such removal from natural habitats results in destructive effects on the ecology of the area (Raimundo da Fonseca et al. 2012). The mass extraction of seaweeds from bloom events may be possible with the added advantage of removing the toxic build-up of rotten seaweed from the coastline (Aziza et al. 2018). Aquaculture of seaweed is a potential method to allow for large-scale production in a sustainable and environmentally friendly manner. The brown seaweed, Ascophyllum nodosum is sustainably harvested in Norway, Ireland, and Iceland due to its commercial potential as a source of alginates and use as agricultural fertiliser, and has been incorporated into animal feed (Algaebase 2019). Therefore, it is not surprising that A. nodosum is one of the most widely studied seaweed species with its potential application as a protectant against plant diseases been tested on several crops (Wite et al. 2015; Ali et al. 2016).
A potential solution to facilitate the application of seaweeds as a novel fungicide involves the identification of these bioactive(s) which would allow for the compound responsible for such antifungal activity to be synthesised in the laboratory. This would allow large-scale production of these novel compounds without the removal of unsustainable quantities of seaweed from coastal areas and would also give a valuable insight into their mode of action. Few authors have definitively identified such bioactive compounds with the majority of researchers highlighting the requirement for further purification. Such studies generally only indicate a number of key compounds which may be responsible for such activity, including phenol compounds whose mode of action is believed to lie in their ability to disrupt the cytoplasmic membrane of the pest, causing cell leakage (Martinez 2012). Steroids such as terpenes composed of a specific ring structure of four cycloalkane rings, often known as glycosylated, are potent compounds that interact with sterols in the pest’s membranes (Martinez 2012). A number of studies have reported specific compounds responsible for antifungal activity in seaweeds (Puglisi et al. 2004; Kim et al. 2017). Barreto and Meyer (2006) isolated the biologically active compound lanosol ethyl ether (C9H10O3Br2) from the red seaweed Osmundaria serrata which exhibited fungicidal activity against the leaf spot causing fungus, Alternaria alternata. Kamada et al. (2018) also isolated compounds from a red seaweed, Laurencia nangii: (1) tricyclic brominated ditepenoid, neoiriepentaol, (2) C15-acetogenin, nangenyne and two other known compounds of (3) neoiriepentaol, and (4) dactyloxene A. Compounds 1–3 were found to be potent antifungal compounds against marine fungi. Machado et al. (2014a) evaluated the chemical profile of Ochtodes secundiramea with C10H12Br2Cl identified as the major compound with the biomass exhibiting activity against Cladosporium sphaerospermum and Colletotrichum lagenarium. In in vivo studies, the bioactive compounds are believed to exhibit elicitor activity through enhancing the expression of defence enzymes in the host. Abouraïcha et al. (2016) isolated two saccharides, glucuronan and oligoglucuronans, from the green seaweed, U. lactuca, which were found to effectively control blue mould caused by Penicillium expansum and grey mould caused by Botrytis cinerea in apple fruit. These compounds were found to trigger a rapid and transient buildup of hydrogen peroxide and activation of antioxidant-related enzymes including catalase and superoxide dismutase. An increase in the activity of phenylalanine ammonialyase, polyphenoloxydase, and peroxydase was also observed and was accompanied by heightened levels of ligins and phenols (Abouraïcha et al. 2016). These changes in the apple fruit therefore suggested that the saccharide compounds had the ability to protect the fruit through activation of defence enzymes and metabolites rather than direct antifungal activity on the pathogens. Therefore, from the comparison of the studies, it can be seen that there is a difference between the proposed mode of actions for in vitro and in vivo studies.
The identification of the bioactive compounds would also allow the relative toxicity of these compounds to be evaluated. This is highly important since these seaweeds will be exposed to the environment with their impact on health whether it be human, animal, or environmental determined through toxicity tests and studying their effects on non-target species. Subsequently, it is important for commercialisation purposes to have a product with consistent efficacy, which will involve determining the stability of the particular bioactives (Uppal et al. 2008).
Conclusions
From this review, it can be seen that there are many steps involved in the isolation of the compounds of interest from seaweeds including collection, drying, extraction, and finally assessment of the antifungal potential of these generated extracts. The choice of methods is generally restricted by the properties of the bioactives, cost, or simply from a lack of expertise or equipment. Significant progress in the area of identification is still required for full commercial use of these novel compounds. Nonetheless, the reported studies indicate that seaweeds have a broad range of activity from fungicidal (Ameer et al. 2016; Esserti et al. 2016; Righini et al. 2018) to larvicidal (Guedes et al. 2014; Valentina et al. 2015; Salvador-Neto et al. 2016) with the added advantage of being biopesticides, including their safety to non-target organisms, their environmental fit, and relative ease of registration, especially in the USA compared with the EU (Balog et al. 2017). Establishing the potential of low-risk alternatives to conventional fungicides is gaining increased interest among those aiming to develop safe, eco-friendly products for Integrated Crop Management (ICM) (Kumar and Singh 2014).
References
Abbassy AM, Marzouk AM, Rabea IE, Abd-Elnabi DA (2014) Insecticidal and fungicidal activity of Ulva lactuca Linnaeus (Chlorophyta) extracts and fractions. Annu Res Rev Biol 4:2252–2262
Abkhoo J, Sabbagh SK (2015) Control of Phytophthora melonis damping-off, induction of defense responses, and gene expression of cucumber treated with commercial extract from Ascophyllum nodosum. J Appl Phycol 28:1333–1342
Abouraïcha E, El Alaoui-Talibi Z, El Boutachfaiti R, Petit E, Courtois B, Courtois J, El Modafar C (2015) Induction of natural defense and protection against Penicillium expansum and Botrytis cinerea in apple fruit in response to bioelicitors isolated from green algae. Sci Hortic 181:121–128
Abouraïcha EF, El Alaoui-Talibi Z, Tadlaoui-Ouafi A, El Boutachfaiti R, Petit E, Douira A, Courtois B, Courtois J, El Modafar C (2016) Glucuronan and oligoglucuronans isolated from green algae activate natural defense responses in apple fruit and reduce postharvest blue and gray mold decay. J Appl Phycol 29:471–480
Ali N, Ramkissoon A, Ramsubhag A, Jayaraman J (2016) Ascophyllum extract application causes reduction of disease levels in field tomatoes grown in a tropical environment. Crop Prot 83:67–75
Allen ED, Hatfield G (2004) Medicinal plants in folk tradition. An ethnobotany of Britain and Ireland. Timber Press, Portland 431 pp
Algaebase (2019) The seaweed site: information on marine algae http://www.seaweed.ie. Accessed 18 Sept 2015
Ambika S, Sujatha K (2015) Antifungal activity of aqueous and ethanol extracts of seaweeds against sugarcane red rot pathogen (Colletotrichum falcatum). Sci Res Essays 10:232–235
Ameer JBM, Selvaraju P, Vijayakumar A (2016) Evaluation of antifungal activity of seaweed extract (Turbinaria conoides) against Fusarium oxysporum. J Appl Nat Sci 8:60–62
Araujo L, Stadnik MJ (2013) Cultivar-specific and ulvan-induced resistance of apple plants to Glomerella leaf spot are associated with enhanced activity of peroxidases. Acta Sci Agron 35:287–293
Aruna P, Mansuya P, Sridhar S, Kumar SJ, Babu S (2010) Pharmacognostical and antifungal activity of selected seaweeds from Gulf of Manner Region. Recent Res Sci Technol 2:115–119
Asha A, Rathi MJ, Raja PD, Sahayaraj K (2012) Biocidal activity of two marine green algal extracts against third instar nymph of Dysdercus cingulatus (Fab.) (Hemiptera: Pyrrhocoridae). J Biopesticid 5:129–134
Asiegbu OF, Adomas A, Stenlid J (2005) Conifer root and butt rot caused by Heterobasidion annosum (Fr.) Bref. s.l. Mol Plant Pathol 6:395–409
Aziza HS, Flower EM, Margareth SK, Aviti JM, Evalyn WM, Eystein S, Helena AN, Jan LL (2018) Health problems related to algal bloom among seaweed farmers in coastal areas of Tanzania. J Public Health Epidemiol 10:303–312
Azwanida NN (2015) A review on the extraction methods use in medicinal plants, principle, strength and limitation. Med Aromat Plants 04:1–6
Balog A, Hartel T, Loxdale HD, Wilson K (2017) Differences in the progress of the biopesticide revolution between the EU and other major crop-growing regions. Pest Manag Sci 73:2203–2208
Balouiri M, Sadiki M, Ibnsouda SK (2016) Methods for in vitro evaluating antimicrobial activity: a review. J Pharm Anals 6:71–79
Bandyopadhyay RF, Frederiksen RA (1999) Contemporary global movement of emerging plant diseases. Ann N Y Acad Sci 894:28–26
Barreto M, Meyer JJM (2006) Isolation and antimicrobial activity of a lanosol derivative from Osmundaria serrata (Rhodophyta) and a visual exploration of its biofilm covering. S Afr J Bot 72:521–528
Barreto M, Straker CJ, Critchley AT (1997) Short note on the effects of ethanolic extracts of selected South African seaweeds on the growth of commercially important plant pathogens, Rhizoctonia solani Kühn and Verticillium sp. S Afr J Bot 63:521–523
Bianco EM, Pires L, Santos GKN, Dutra KA, Reis TNV, Vasconcelos ERTPP, Cocentino ALM, Navarro DMAF (2013) Larvicidal activity of seaweeds from northeastern Brazil and of a halogenated sesquiterpene against the dengue mosquito (Aedes aegypti). Ind Crop Prod 43:270–275
Bilal H, Sahar S, Din S (2017) Bio-pesticides: new tool for the control of Aedes (Stegomyia) albopictus (Culicidae: Diptera) in Pakistan. J Arthropod Borne Dis 11:278–285
Brownlee IA, Fairclough A.C., Hall A.C., Paxman J.R. (2011) Dietary seaweed and human health. In: Culinary arts and sciences VII: global, national and local perspectives. Bournemouth University International Centre for Tourism and Hospitality Research. Bournemouth University, UK, pp 82–88
Brownlee AI, Fairclough CA, Hall CA, Paxmam RJ (2012) The potential health benefits of seaweed and seaweed extract. In: Pomin VH (ed) Seaweed: ecology, nutrient composition and medicinal uses. Marine Biology: Earth Sciences in the 21st Century. Nova Science Publishers, New York, pp 119–136
Buch AC, Brown GG, Niva CC, Sautter KD, Sousa JP (2013) Toxicity of three pesticides commonly used in Brazil to Pontoscolex corethrurus (Müller, 1857) and Eisenia andrei (Bouché, 1972). Appl Soil Ecol 69:32–38
Cespedes CL, Salazar JR, Ariza-Castolo A, Yamaguchi L, Avila JG, Aqueveque P, Kubo I, Alarcon J (2014) Biopesticides from plants: Calceolaria integrifolia s.l. Environ Res 132:391–406
Chagnon M, Kreutzweiser D, Mitchell EA, Morrissey CA, Noome DA, Van der Sluijs JP (2015) Risks of large-scale use of systemic insecticides to ecosystem functioning and services. Environ Sci Pollut Res Int 22:119–134
Chanthini K, Kumar SC, Kingsley JS (2012) Antifungal activity of seaweed extracts against phytopathogen Alternaria solani. J Acad Indust Res 1:86–90
Chemat F, Vian MA, Cravotto G (2012) Green extraction of natural products: concept and principles. Int J Mol Sci 13:8615–8627
Coorevits L, Boelens J, Claeys G (2015) Direct susceptibility testing by disk diffusion on clinical samples: a rapid and accurate tool for antibiotic stewardship. Eur J Clin Microbiol Infect Dis 34:1207–1212
Coşoveanu A, Axîne O, Iacomi B (2010) Antifungal activity of macroalgae extracts. Scient Pap Univ Agronom Sci Vet Med Bucharest 3:442–447
Crasta JP, Raviraja SN, Sridhar RK (1997) Antimicrobial activity of some marine algae of southwest coast of India. Indian J Mar Sci 26:201–205
Cravotto G, Boffa L, Mantegna S, Perego P, Avogadro M, Cintas P (2008) Improved extraction of vegetable oils under high-intensity ultrasound and/or microwaves. Ultrason Sonochem 15:898–902
Cruces E, Rojas-Lillo Y, Ramirez-Kushel E, Atala E, López-Alarcón C, Lissi E, Gómez I (2015) Comparison of different techniques for the preservation and extraction of phlorotannins in the kelp Lessonia spicata (Phaeophyceae): assays of DPPH, ORAC-PGR, and ORAC-FL as testing methods. J Appl Phycol 28:573–580
Damalas AC, Koutroubas DS (2018) Current status and recent developments in biopesticide use. Agriculture 8:1–6
De Corato U, Salimbeni R, De Pretis A, Avella N, Patruno G (2017) Antifungal activity of crude extracts from brown and red seaweeds by a supercritical carbon dioxide technique against fruit postharvest fungal diseases. Postharvest Biol Technol 131:16–30
De Felicio R, De Albuquerque S, Young MC, Yokoya NS, Debonsi HM (2010) Trypanocidal, leishmanicidal and antifungal potential from marine red alga Bostrychia tenella J. Agardh (Rhodomelaceae, Ceramiales). J Pharm Biomed Anal 52:763–769
de Freitas MB, Stadnik MJ (2012) Race-specific and ulvan-induced defense responses in bean (Phaseolus vulgaris) against Colletotrichum lindemuthianum. Physiol Mol Plant Pathol 78:8–13
Dewanjee S, Gangopadhyay M, Bhattacharya N, Khanra R, Dua TK (2015) Bioautography and its scope in the field of natural product chemistry. J Pharm Anals 5:75–84
Ehteshamul-Haque S, Baloch NG, Sultana V, Ara J, Tariq MR, Athar M (2013) Impact of seaweeds on fluorescent Pseudomonas and their role in suppressing the root disease of soybean and pepper. J Appl Biol Food Qual 86:126–132
El Modafar C, Elgadda M, El Boutachfaiti R, Abouraicha E, Zehhar N, Petit E, El Alaoui-Talibi Z, Courtois B, Courtois J (2012) Induction of natural defence accompanied by salicylic acid-dependant systemic acquired resistance in tomato seedlings in response to bioelicitors isolated from green algae. Sci Hortic 138:55–63
Esserti S, Smaili A, Rifai LA, Koussa T, Makroum K, Belfaiza M, Kabil EM, Faize L, Burgos L, Alburquerque N, Faize M (2016) Protective effect of three brown seaweed extracts against fungal and bacterial diseases of tomato. J Appl Phycol 29:1081–1093
Forests, products and people Ireland’s forest policy – a renewed vision (2013) https://www.agriculture.gov.ie/media/migration/forestry/publicconsultation/forestpolicyreview/ForestPolicyReviewpublicconsult21Jun2013.pdf. Accessed 16 Aug 2016
Galal MRH, Salem MW, Naser El-Deen F (2011) Biological control of some pathogenic fungi using marine algae. Res J Microbiol 6:645–657
Garcia-Vaquero M, Rajauria G, Tiwari B, Sweeney T, O’Doherty J (2018) Extraction and yield optimisation of fucose, glucans and associated antioxidant activities from Laminaria digitata by applying response surface methodology to high intensity ultrasound-assisted extraction. Mar Drugs 16(8):E257
González de Peredo AV, Vazquez-Espinosa M, Espada-Bellido E, Jimenez-Cantizano A, Ferreiro-Gonzalez M, Amores-Arrocha A, Palma M, Barroso CG, Barbero GF (2018) Development of new analytical microwave-assisted extraction methods for bioactive compounds from Myrtle (Myrtus communis L.). Molecules 23:2992
Grzywacz D, Stevenson PC, Mushobozi LM, Belmain S, Wilson K (2014) The use of indigenous ecological resources for pest control in Africa. Food Sec 6:71–86
Guedes EA, Araujo MA, Souza AK, de Souza LI, de Barros LD, Maranhao FC, Sant’Ana AE (2012) Antifungal activities of different extracts of marine macroalgae against dermatophytes and Candida species. Mycopathologia 174:223–232
Guedes EA, de Carvalho CM, Ribeiro Junior KA, Lisboa Ribeiro TF, de Barros LD, de Lima MR, Prado Moura Fde B, Goulart Sant’ana AE (2014) Larvicidal activity against Aedes aegypti and molluscicidal activity against Biomphalaria glabrata of Brazilian marine algae. J Parasitol Res 2014:501328
Henriques J, Nóbrega F, Sousa E, Lima A (2015) Morphological and genetic diversity of Biscogniauxia mediterranea associated to Quercus suber in the Mediterranean Basin. Rev Ciên Agrár 38:166–175
Hernández-Herrera RM, Virgen-Calleros G, Ruiz-López M, Zañudo-Hernández J, Délano-Frier JP, Sánchez-Hernández C (2014) Extracts from green and brown seaweeds protect tomato (Solanum lycopersicum) against the necrotrophic fungus Alternaria solani. J Appl Phycol 26:1607–1614
Hernández-Ledesma B, Herrero M (2014) Bioactive compounds from marine foods: plant and animal sources. John Wiley & Sons, West Sussex
Ibañez E, Herrero M, Mendiola JA, Castro-Puyana M (2012) Extraction and characterization of bioactive compounds with health benefits from marine resources: macro and micro algae, cyanobacteria, and invertebrates. In: Hayes M (ed) Marine bioactive compounds. Springer, Boston, pp 55–98
Ibraheem BMI, Hamed MS, Abd Elrhman AA, Farag MF, Abdel-Raouf N (2017) Antimicrobial activities of some brown macroalgae against some soil borne plant pathogens and in vivo management of Solanum melongena root diseases. Aust J Basic Appl Sci 11:157–168
Ivase TJ-P, Nyakuma BB, Ogenyi BU, Balogun AD, Hassan MN (2017) Current status, challenges, and prospects of biopesticide utilization in Nigeria. Agriculture and Environment 9:95–106
Jallow MF, Awadh DG, Albaho MS, Devi VY, Thomas BM (2017) Pesticide knowledge and safety practices among farm workers in Kuwait: results of a survey. Int J Environ Res Public Health 14:E430
Jayaraj J, Wan A, Rahman M, Punja ZK (2008) Seaweed extract reduces foliar fungal diseases on carrot. Crop Prot 27:1360–1366
Jayaraman J, Norrie J, Punja ZK (2011) Commercial extract from the brown seaweed Ascophyllum nodosum reduces fungal diseases in greenhouse cucumber. J Appl Phycol 23:353–361
Jiang L (2011) Comparison of disk diffusion, agar dilution, and broth microdilution for antimicrobial susceptibility testing of five chitosans. MSc Thesis, Lousiana State University
Jimenez E, Dorta F, Medina C, Ramirez A, Ramirez I, Pena-Cortes H (2011) Anti-phytopathogenic activities of macro-algae extracts. Mar Drugs 9:739–756
Joana Gil-Chávez G, Villa JA, Fernando Ayala-Zavala J, Basilio Heredia J, Sepulveda D, Yahia EM, González-Aguilar GA (2013) Technologies for extraction and production of bioactive compounds to be used as nutraceuticals and food ingredients: an overview. Compr Rev Food Sci Food Saf 12:5–23
Kabir Khanzada KA, Shaikh W, Kazi GT, Kabir S, Soofia S (2007) Antifungal activity, elemental analysis and determination of total protein of seaweed, Solieria robusta (Greville) Kylin from the coast of Karachi. Pak J Bot 39:931–937
Kadam SU, Tiwari BK, Smyth TJ, O’Donnell CP (2015) Optimization of ultrasound assisted extraction of bioactive components from brown seaweed Ascophyllum nodosum using response surface methodology. Ultrason Sonochem 23:308–316
Kamada T, Phan C-S, Vairappan CS (2018) Neoiriepentaol and nangenyne, halogenated diterpenoid and C15-acetogenin from red alga Laurencia nangii Masuda collected in Borneo. J Appl Phycol 30:3379–3386
Karmann M, Miettinen P, Hontelez J (2016) Forest Stewardship Council indicators: Development by multi-stakeholder process assures consistency and diversity. In. Policy matters 2016: Certification and biodiversity. https://www.iucn.org/sites/dev/files/policy_matters_21_chapter_8_forest_stewardship_council_indicators_development_by_multi-stakeholder_process_assures_consistency_and_diversity.pdf. Accessed 29 Apr 2019
Kausalya M, Narasimha RMG (2015) Antimicrobial activity of marine algae. J Algal Biomass Utln 6:78–87
Khan AS, Abid M, Hussain F (2017) Antifungal activity of aqueous and methanolic extracts of some seaweeds against common soil-borne plant pathogenic fungi. Pak J Bot 49:1211–1216
Kim MS, Jung J-Y, Kwon N-O, Cha HK, Um H-B, Chung D, Pan H-C (2012) A potential commercial source of fucoxanthin extracted from the microalga Phaeodactylum tricornutum. Appl Biochem Biotechnol 166:1843–1855
Kim K-H, Yu D, Eom S-H, Kim H-J, Kim D-H, Song H-S, Kim D-M, Kim Y-M (2017) Fucofuroeckol-A from edible marine alga Eisenia bicyclis to restore antifungal activity of fluconazole against fluconazole-resistant Candida albicans. J Appl Phycol 30:605–609
Kolanjinathan KSD (2009) Antibacterial activity of marine macro algae against human pathogens. Recent Res Sci Technol 1:020–022
Kulshreshtha G, Borza T, Rathgeber B, Stratton GS, Thomas NA, Critchley A, Hafting J, Prithiviraj B (2016) Red seaweeds Sarcodiotheca gaudichaudii and Chondrus crispus down regulate virulence factors of Salmonella enteritidis and induce immune responses in Caenorhabditis elegans. Front Microbiol 7:421
Kumar S, Singh A (2014) Biopesticides for integrated crop management: environmental and regulatory aspects. J Biofert Biopesticid 5:1
Lah K (2011) Effects of pesticides on human health. In: Toxipedia. http://www.toxipedia.org/display/toxipedia/Effects+of+Pesticides+on+Human+Health. Accessed 19 Feb 2019
Kumar R, Shrivastava SK, Chakraborti A (2010) Comparison of broth dilution and disc diffusion method for the antifungal susceptibility testing of Aspergillus flavus. Amer J Biomed Sci. https://doi.org/10.5099/aj100300202:202-208
Kumar SN, Nambisan B, Sundaresan A, Mohandas C, Anto JR (2013) Isolation and identification of antimicrobial secondary metabolites from Bacillus cereus associated with a rhabditid entomopathogenic nematode. Ann Microbiol 64:209–218
Li H, Deng Z, Wu T, Liu W, Loewen S, Tsao R (2012) Microwave-assisted extraction of phenolics with maximal antioxidant activities in tomatoes. Food Chem 130:928–936
Machado LP, de Carvalho LR, Young MCM, Zambotti-Villela L, Colepicolo P, Andreguetti DX, Yokoya NS (2014a) Comparative chemical analysis and antifungal activity of Ochtodes secundiramea (Rhodophyta) extracts obtained using different biomass processing methods. J Appl Phycol 26:2029–2035
Machado LP, Matsumoto ST, Jamal CM, da Silva MB, Centeno Dda C, Colepicolo Neto P, de Carvalho LR, Yokoya NS (2014b) Chemical analysis and toxicity of seaweed extracts with inhibitory activity against tropical fruit anthracnose fungi. J Sci Food Agric 94:1739–1744
Macpherson MF, Kleczkowski A, Healey JR, Quine CP, Hanley N (2017) The effects of invasive pests and pathogens on strategies for forest diversification. Ecol Model 350:87–99
Mandal C, Mandal V, Das A (2015) Essentials of Botanical Extraction. Elsevier Science, San Diego
Mani SD, Nagarathnam R (2018) Sulfated polysaccharide from Kappaphycus alvarezii (Doty) Doty ex P.C. Silva primes defense responses against anthracnose disease of Capsicum annuum Linn. Algal Res 32:121–130
Manivannan K, Karthikai devi G, Anantharaman P, Balasubramanian T (2011) Antimicrobial potential of selected brown seaweeds from Vedalai coastal waters, Gulf of Mannar. Asian Pac J Trop Biomed 1:114–120
Martinez JA (2012) Natural fungicides obtained from plants. In: Dhanasekaran D (ed) Fungicides for Plants and Animal Diseases. Rijeka, Intech, pp 1–28
McCracken RA (2013) Current and emerging threats to Ireland’s trees from diseases and pests. Irish For 70:36–60
McKennedy J, Önenç S, Pala M, Maguire J (2016) Supercritical carbon dioxide treatment of the microalgae Nannochloropsis oculata for the production of fatty acid methyl esters. J Supercrit Fluids 116:264–270
Mei Ling LA, Yasir S, Matanjun P, Abu Bakar FM (2015) Effect of different drying techniques on the phytochemical content and antioxidant activity of Kappaphycus alvarezii. J Appl Phycol 27:1717–1723
Melecchi MI, Peres VF, Dariva C, Zini CA, Abad FC, Martinez MM, Caramao EB (2006) Optimization of the sonication extraction method of Hibiscus tiliaceus L. flowers. Ultrason Sonochem 13:242–250
Nicolopoulou-Stamati P, Maipas S, Kotampasi C, Stamatis P, Hens L (2016) Chemical pesticides and human health: the urgent need for a new concept in agriculture. Front Public Health 4:148
Norra I, Aminah A, Suri R (2016) Effects of drying methods, solvent extraction and particle size of Malaysian brown seaweed, Sargassum sp on the total phenolic. Int Food Res J 23:1558–1563
Opgenorth CD, Endo MR (1983) Evidence that antagonistic bacteria suppress Fusarium wilt of celery in neutral and alkaline soils. Phytopathology 73:703–708
Pádu D, Rocha E, Gargiulo D, Ramos AA (2015) Bioactive compounds from brown seaweeds: phloroglucinol, fucoxanthin and fucoidan as promising therapeutic agents against breast cancer. Phytochem Lett 14:91–98
Panda KS (2012) Screening methods in the study of antimicrobial properties of medicinal plants. Int J Biotechnol Res 2:1–35
Pandithurai M, Subbiah M, Vajiravelu S, Selvan T (2015) Antifungal activity of various solvent extracts of marine brown alga Spatoglossum asperum. Int J Pharm Chem 5:277–280
Pasdaran A, Hamedi A, Mamedoy N (2016) Antibacterial and insecticidal activity of volatile compounds of three algae species of Oman Sea. Int J Second Metab 3:66–73
Peres FCJ, Retz de Carvalho L, Gonçalez E, Berian SOL, Felicio DJ (2012) Evalution of antifungal activity of seaweed extracts. Ciênc Agrotec, Lavras 36:294–299
Pérez MJ, Falqué E, Domínguez H (2016) Antimicrobial Action of Compounds from Marine Seaweed. Mar Drugs 14(3):E52
Ponmari G, Sathishkumar R, Lakshmi PTV (2011) Effect of drying treatment on the contents of antioxidants in Cardiospermum halicacabum Linn. Int J Pharm Bio Sci 2:304–313
Puglisi MP, Tan LT, Jensen PR, Fenical W (2004) Capisterones A and B from the tropical green alga Penicillus capitatus: unexpected anti-fungal defenses targeting the marine pathogen Lindra thallasiae. Tetrahedron 60:7035–7039
Raimundo da Fonseca R, Ortiz-Ramírez AF, Cavalcanti ND, Ramos BJC, Teixeira LV, Pedro da Silva Sousa Filho A (2012) Allelopathic potential of extracts the from marine macroalga Plocamium brasiliense and their effects on pasture weed. Aust J Pharm 22:850–853
Rajesh S, Asha A., Kombiah P., Sahayaraj K (2011) Biocidal activity of algal seaweed on insect pest and fungal plant pathogens. National Seminar on Harmful/Benefical Insects of Agricultural Importance. https://doi.org/10.13140/RG.2.1.2226.2887:86-91
Ramkissoon A, Ramsubhag A, Jayaraman J (2017) Phytoelicitor activity of three Caribbean seaweed species on suppression of pathogenic infections in tomato plants. J Appl Phycol 29:3235–3244
Ravikumar S, Ali SM, Beula MJ (2011) Mosquito larvicidal efficacy of seaweed extracts against dengue vector of Aedes aegypti. Asian Pac J Trop Biomed 1:143–146
Rex JH, Pfaller MA, Walsh TJ, Chaturvedi V, Espinel-Ingroff A, Ghannoum MA, Gosey LL, Odds FC, Rinaldi MG, Sheehan DJ, Warnock DW (2001) Antifungal susceptibility testing: practical aspects and current challenges. Clin Microbiol Rev 14:643–658
Righini H, Roberti R, Baraldi E (2018) Use of algae in strawberry management. J Appl Phycol 30:3551–3564
Roberti R, Righini H, Reyes PC (2016) Activity of seaweed and cyanobacteria water extracts against Podosphaera xanthii on zucchini. Ital J Mycol 45:66–77
Rocha OP, De Felicio R, Rodrigues AH, Ambrosio DL, Cicarelli RM, De Albuquerque S, Young MC, Yokoya NS, Debonsi HM (2011) Chemical profile and biological potential of non-polar fractions from Centroceras clavulatum (C. Agardh) Montagne (Ceramiales, Rhodophyta). Molecules 16:7105–7114
Rodriguez-Jasso RM, Mussatto SI, Pastrana L, Aguilar CN, Teixeira JA (2011) Microwave-assisted extraction of sulfated polysaccharides (fucoidan) from brown seaweed. Carbohydr Polym 86:1137–1144
Saha M, Goecke F, Bhadury P (2018) Minireview: algal natural compounds and extracts as antifoulants. J Appl Phycol 30:1859–1874
Saleh B, Al-Mariri A (2018) Antifungal activity of crude seaweed extracts collected from Lattakia Coast, Syria. J Fish Aquat Sci 13:49–55
Salhi N, Mohammed Saghir SA, Terzi V, Brahmi I, Ghedairi N, Bissati S (2017) Antifungal activity of aqueous extracts of some dominant Algerian medicinal plants. Biomed Res Int 2017:7526291
Salvador-Neto O, Gomes SA, Soares AR, Machado FL, Samuels RI, Nunes da Fonseca R, Souza-Menezes J, Moraes JL, Campos E, Mury FB, Silva JR (2016) Larvicidal potential of the halogenated sesquiterpene (+)-obtusol, isolated from the alga Laurencia dendroidea J. Agardh (Ceramiales: Rhodomelaceae), against the Dengue vector mosquito Aedes aegypti (Linnaeus) (Diptera: Culicidae). Mar Drugs 14(2):E20
Santini A, Ghelardini L, De Pace C, Desprez-Loustau ML, Capretti P, Chandelier A, Cech T, Chira D, Diamandis S, Gaitniekis T, Hantula J, Holdenrieder O, Jankovsky L, Jung T, Jurc D, Kirisits T, Kunca A, Lygis V, Malecka M, Marcais B, Schmitz S, Schumacher J, Solheim H, Solla A, Szabo I, Tsopelas P, Vannini A, Vettraino AM, Webber J, Woodward S, Stenlid J (2013) Biogeographical patterns and determinants of invasion by forest pathogens in Europe. New Phytol 197:238–250
Shobier AH, Abdel Ghani SA, Barakat KM (2016) GC/MS spectroscopic approach and antifungal potential of bioactive extracts produced by marine macroalgae. Egypt J Aquat Res 42:289–299
Shukla S (2011) Freeze drying process: a review. Int J Pharm Sci Res 2:3061–3068
Singh D (2014) Advances in plant biopesticides. Springer India, New Delhi
Sosa-Hernandez JE, Escobedo-Avellaneda Z, Iqbal HMN, Welti-Chanes J (2018) State-of-the-art extraction methodologies for bioactive compounds from algal biome to meet bio-economy challenges and opportunities. Molecules 23(11):E2953
Stein EM, Colepicolo P, Afonso FAK, Fujii MT (2011) Screening for antifungal activities of extracts of the brazilian seaweed genus Laurencia (Ceramiales, Rhodophyta). Rev Bras Farm 21:290–295
Stewart KL (2008) Conventional and novel treatments for control of clubroot disease of brassicas. PhD Thesis, The University of Edinburgh
Sujatha K, Mahalakshmi P, Manonmani K (2014) Effect of antifungal activity of seaweed extracts against soil borne pathogens in pulses. Int J Agric Innov Res 3:135–137
Sultana V, Ehteshamul-Haque S, Ara J, Athar M (2005) Comparative efficacy of brown, green and red seaweeds in the control of root infecting fungi and okra. Int J Environ Sci 2:129–132
Sultana V, Ara J, Ehteshamul-Haque S (2008) Supression of root rotting fungi and root rot knot nematode of chili by seaweed and Pseudomonas aeruginosa. J Phytopathol 156:390–395
Sultana V, Ehteshamul-Haque S, Ara J, Athar M (2009) Effect of brown seaweeds and pesticides on root rotting fungi and root-knot nematode infecting tomato roots. J Appl Bot Food Qual 83:50–53
Sultana V, Baloch NG, Ara J, Ehteshamul-Haque S, Tariq MR, Athar M (2011a) Seaweeds as an alternative to chemical pesticides for the management of root diseases of sunflower and tomato. J Appl Bot Food Qual 84:162–168
Sultana V, Baloch NG, Ambreen, Ara J, Tariq RM, Ehteshamul-Haque S (2011b) Comparative efficacy of a red alga Solieria robusta, chemical fertilizers and pesticides in managing the root diseases and growth of soybean. Pak J Bot 43:1–6
Sutton ML, Starzyk JM (1972) Procedure and analysis of a useful method in determining mycelial dry weights from agar plates. Appl Microbiol 24:1011–1012
Thakur M, Sohal BS (2013) Role of elicitors in inducing resistance in plants against pathogen infection: a review. ISRN Biochem 2013:762412
Tubby KV, Webber JF (2010) Pests and diseases threatening urban trees under a changing climate. Forestry 83:451–459
Tyskiewicz K, Konkol M, Roj E (2018) The application of supercritical fluid extraction in phenolic compounds isolation from natural plant materials. Molecules 23(10):E2625
Uppal AK, El Hadrami A, Adam LR, Tenuta M, Daayf F (2008) Biological control of potato Verticillium wilt under controlled and field conditions using selected bacterial antagonists and plant extracts. Biol Control 44:90–100
Valentina J, Poonguzhali TV, Josmin L, Nisha LL (2015) Mosquito larvicidal and pupicidal activity of seaweed extracts against Aedes aegypti, Anopheles stephensi and Culex quinquefasciatus. Int J Mosquito Res 2:54–59
Vázquez FIA, Sánchez DMC, Delgado GN, Alfonso SMA, Ortega SY, Sánchez CH (2011) Anti-inflammatory and analgesic activities of red seaweed Dichotomaria obtusata. Braz J Pharm Sci 47:111–118
Villaverde JJ, Sevilla-Moran B, Sandin-Espana P, Lopez-Goti C, Alonso-Prados JL (2014) Biopesticides in the framework of the European Pesticide Regulation (EC) No. 1107/2009. Pest Manag Sci 70:2–5
Vimala TP, Poonghuzhali TV (2017) In vitro antimicrobial activity of solvent extracts of marine brown alga, Hydroclathrus clathratus (C. Agardh) M. Howe from Gulf of Mannar. J Appl Pharmaceut Sci 7:157–162
Wang L, Weller CL (2006) Recent advances in extraction of nutraceuticals from plants. Trends Food Sci Technol 17:300–312
Wite D, Mattner SW, Porter IJ, Arioli T (2015) The suppressive effect of a commercial extract from Durvillaea potatorum and Ascophyllum nodosum on infection of broccoli by Plasmodiophora brassicae. J Appl Phycol 27:2157–2161
Woodcock AB, Bullock MJ, Shore FR, Heard SM, Pereira GM, Redhead J, Ridding L, Dean H, Slepp D, Henrys P, Peyton J, Hulmes S, Hulmes L, Sárospataki M, Saure C, Edwards M, Genersch E, Knäbe S, Pywell FR (2017) Country-specific effects of neonicotinoid pesticides on honey bees and wild bees. Science 356:1393–1395
Wrona O, Rafinska K, Mozenski C, Buszewski B (2017) Supercritical fluid extraction of bioactive compounds from plant materials. J AOAC Int 100:1624–1635
Yamada S, Cao J, Sumita O, Kurasawa K, Kurata H, Oh K, Matsuoka H (1992) Automatic antifungal activity analyzing system on the basis of dynamic growth process of a single hypha. Mycopathologia 118:65–69
Ying Z, Han X, Li J (2011) Ultrasound-assisted extraction of polysaccharides from mulberry leaves. Food Chem 127:1273–1279
Zakaria AN, Ibrahim D, Sulaiman FS, Supardy AN (2011) Assessment of antioxidant activity, total phenolic content and invitro toxicity of Malaysian red seaweed, Acanthophora spicifera. J Chem Pharm Res 3:182–191
Zhang Y, Han J, Mu J, Feng Y, Gu X, Ji Y (2013) Bioactivity and constituents of several common seaweeds. Chin Sci Bull 58:2282–2289
Zhang QW, Lin LG, Ye WC (2018) Techniques for extraction and isolation of natural products: a comprehensive review. Chin Med 13:20
Funding
The authors would like to thank the Waterford Institute of Technology PhD Scholarship Programme for funding this research.
Author information
Authors and Affiliations
Corresponding author
Additional information
Publisher’s note
Springer Nature remains neutral with regard to jurisdictional claims in published maps and institutional affiliations.
Rights and permissions
About this article
Cite this article
O’ Keeffe, E., Hughes, H., McLoughlin, P. et al. Methods of analysis for the in vitro and in vivo determination of the fungicidal activity of seaweeds: a mini review. J Appl Phycol 31, 3759–3776 (2019). https://doi.org/10.1007/s10811-019-01832-7
Received:
Revised:
Accepted:
Published:
Issue Date:
DOI: https://doi.org/10.1007/s10811-019-01832-7