Abstract
Eight different surface sediment samples (K1–K8) were collected from two separate areas of Lake Koumoundourou and two samples (E1 and E2) from one area of Elefsis Bay, Athens, Greece. The level of pollution attributed to heavy metals was evaluated using several pollution indicators. Degree of Contamination, Modified Contamination Degree and Geoaccumulation Indexes were applied in order to determine and assess the anthropogenic contribution of the selected six elements (Cr, Ni, Cu, Zn, As and Pb). Moreover, the adverse effects of the sediments to aquatic organisms, from both heavy metals and polycyclic aromatic hydrocarbons (PAHs), were determined by using Sediment Quality Guidelines. The results indicated that Lake Koumoundourou is contaminated with heavy metals in a moderate degree and almost 50 % of the sediments are associated with frequent observation of adverse effects, when it comes to Ni and occasional observation of adverse effects, when it comes to Cu, Zn and Pb. As far as PAHs are concerned, around 60 % of the samples can be occasionally associated to toxic biological effects according to the effect-range classification for phenanthrene, benzo(a)anthracene, chrysene and pyrene. Finally, samples taken from the north side of the lake are more contaminated with PAHs than the ones taken from the east side probably due to the existence of the water barrier which acts as a reservoir of PAHs.
Similar content being viewed by others
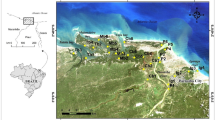
Explore related subjects
Discover the latest articles, news and stories from top researchers in related subjects.Avoid common mistakes on your manuscript.
Introduction
Unfortunately, nowadays, the presence of heavy metals in lake and sea sediments has created an alarming situation that requires immediate attention. Careless or inappropriate disposal of industrial wastes, pipeline leaks, faulty drainage systems and insufficient maintenance of petroleum tanks are some of the reasons that often lead to contamination of the surrounding areas not only by organic compounds (e.g. polycyclic aromatic hydrocarbons (PAHs)) but also by heavy metals such as Cr, Ni, Cu, Zn, As and Pb (Fiedler et al. 2009; Ugochukwu 2004; Chindah et al. 2004).
Heavy metals occur naturally in the ecosystem in a variety of concentrations. Some of them are dangerous to human health or to the environment (e.g. mercury, cadmium, lead and chromium), some may cause corrosion (e.g. zinc and lead) and some are harmful in other ways (e.g. arsenic may pollute catalysts) (Hogan 2009). The knowledge of total concentration of a metal in soils or sediments is undoubtedly of great significance, but it is a rather poor indicator of its bioavailability, mobility and toxicity. In order to assess the environmental impact of a contaminated area, the knowledge of the available fraction of a metal in the sediment is a necessity. The metal properties depend, therefore, not only on their total concentration but also on the physicochemical form they appear, a phenomenon characterised by the term “speciation” (Giacalone et al. 2005; Ure et al. 1993)
The distribution and speciation of heavy metals in sediments is a very complex procedure that depends on various parameters such as the concentration of the metal ions, pH and redox potential of the sediment, the anthropogenic impact on aquatic ecosystems caused by the discharge of urban and industrial wastewaters and the natural erosion processes of coastal and seafloor (Tsai et al. 2003; Carman et al. 2007). One of the largest problems associated with the persistence of heavy metals is their low rate of biodegradation causing even greater exposure for some organisms than is present in the environment alone (Diagomanolin et al. 2004).
On the other hand, PAHs are common constituents in aquatic sediments and their origin can be either natural or anthropogenic (Sklivagou et al. 2001). The naturally formed PAHs are biosynthesis products of oil and usually occur in marine sediments in low concentrations (e.g. 0.01–1 ng/g dw) whereas the human origin PAHs occur in higher values and can be easily found in estuaries and coastal areas as well as areas associated with the disposal of oil or petroleum wastes. Some of them such as benzo(a)anthracene, benzo(b)fluoranthene, chrysene and benzo(a)pyrene are considered to be carcinogenic for human (Anyakora et al. 2003; Orecchio and Papuzza 2009). They are present in both dissolved and particulate phases. Due to their low solubility and hydrophobic nature, they can be associated with both organic and inorganic particles, therefore accumulated to relatively high concentrations in sediments (Orecchio 2010; Giacalone et al. 2004). Sediments, therefore, represent the most important recipients of PAHs in the aquatic environment (Culotta et al. 2006).
Pollution indicators
There are a number of methods for calculating the degree of metal enrichment in sediments (Ridgway and Shimmield 2002). Various authors have proposed contamination impact scales in order to affiliate the numerical results provided from the field to descriptive tables of contamination ranging from low to high intensity (Hakanson 1980). These methods do not take into consideration the speciation of heavy metals in sediments, but indicate the level of contamination of a particular area from heavy metals or PAHs, based on their total concentrations. The three methods used in this study are discussed in the following sections.
Degree of contamination
This method is based on the calculation of a contamination factor (C f) for each pollutant. The individual contamination factors are calculated according to the following equation:
where M x and M b respectively refer to the mean concentration of the target metal in at least five sub-samples and the pre-industrial “baseline” sediments.
Then, Hakanson’s (1980) study suggests that the numeric sum of all the aforementioned contamination factors should express the overall degree of contamination in the sediment by using the following formula:
where \( C_{\text{f}}^i \) are the individual contamination factors for the selected element and n is the number of the C fs examined for a specific sediment.
Modified degree of contamination
Unfortunately, Hakanson’s proposal requires seven specific metals plus a polychlorinated biphenyl, hence, all eight species must be analysed in order to calculate the correct degree of contamination (C d). As a consequence of these limitations, Abrahim and Parker (Abrahim and Parker 2008) proposed a variation of this method which takes into consideration all the Cfs for a given set of pollutants divided by the number of the analysed pollutants, e.g. heavy metals (Abrahim and Parker 2002).
where n is the number of analysed elements, i the element and C f the contamination factor.
The mean concentration of a metal requires the analysis of at least three samples of the contaminated sediment and the reference (“baseline”) concentrations are determined from uncontaminated sediments of the surrounding area (Abrahim and Parker 2008).
The classification of the sediments according to the modified degree of contamination (mC d) is the following:
mC d < 1.5 | Zero to very low degree of contamination |
1.5 < mC d < 2 | Low degree of contamination |
2 < mC d < 4 | Moderate degree of contamination |
4 < mC d < 8 | High degree of contamination |
8 < mC d < 16 | Very high degree of contamination |
16 < mC d < 32 | Extremely high degree of contamination |
mC d ≥ 32 | Ultra-high degree of contamination |
Geoaccumulation Index
The geoaccumulation index (I geo), originally introduced for bottom sediments by Muller in 1969 (Abrahim and Parker 2002), enables the assessment of contamination by comparing current and pre-industrial concentrations, using the following equation:
where C n and B n are respectively the measured concentration of the selected element in the sediment and the geochemical background reference concentration value. The constant 1.5 allows us to take into consideration natural fluctuations that may have occurred in the environment of the sediment including possible small anthropogenic inputs (Yaqin et al. 2008).
The classification of the sediments according to the I geo values is the following (Abrahim and Parker 2008; Anagnostou et al. 1997):
I geo | Pollution status |
>5 | Extremely polluted |
4–5 | Strongly to extremely strongly polluted |
3–4 | Strongly polluted |
2–3 | Moderately to strongly polluted |
1–2 | Moderately polluted |
0–1 | Unpolluted to moderately polluted |
<0 | Unpolluted |
Sediment Quality Guidelines
The Sediment Quality Guidelines (SQGs) were originally intended for use by National Oceanic and Atmospheric Administration (NOAA) scientists in ranking areas that warranted further detailed study on the actual occurrence of adverse effects such as toxicity. They were also intended for use in ranking chemicals that might be of potential concern. SQGs developed by NOAA include a large set of effect range guidelines derived from a large series of chemical and biological data collected from North American coastal regions (McCready et al. 2006). SQGs provide concentrations limits of contaminants (heavy metals and PAHs) in sediments in order to ensure safe living of organisms in or near them (Hinkey and Zaidi 2007; Violintzis et al. 2009).
Two sets of guidelines are commonly used, the effects range low/median (ERL/ERM) and threshold/probable effect level (TEL/PEL). The low-range values (ERL or TEL) represent concentrations below which adverse effects upon sediment dwelling organisms are not likely to occur. Upper range values (ERM or PEL) represent concentrations above which adverse effects are expected to appear.
The ERL/ERM indicators use percentiles of the 10th and 50th of metal concentrations that create adverse biological effects respectively. On the other hand, TEL/PEL indicators use the geometric mean of the aforementioned percentiles of concentration values that create or not adverse effects, respectively (Violintzis et al. 2009). Each set of guidelines delineates three ranges of metal concentrations where adverse effects are observed rarely (<ERL or <TEL), occasionally (ERL-ERM or TEL-PEL) and frequently (>ERM or >PEL).
In order to obtain a more realistic measure of the sediments’ toxicity, mean quotients were introduced according to the following equations:
where M i is the concentration of element i in sediments, ERM i and PEL i the guideline values for the element i and n the number of metals. These mean quotients can be used in case of existence of multiple contaminants in the sediment where the adverse effects caused by each chemical are additive and not antagonistic (Violintzis et al. 2009). The sediment quality criteria for eight metals and fourteen PAHs are shown in Table 1 (Burton 2002) and the classification of sediments according to ERMQ and PELQ is as follows:
ERMQ values of <0.1, 0.11–0.5, 0.51–1.5 and >1.5 are related to 12, 30, 46 and 74 %, respectively, that sediments present toxicity in amphipod survival bioassays. Similarly, PELQ, values of <0.1, 0.11–1.5, 1.51–2.3 and >2.3 coincide with 10, 25, 50 and 76 %, of toxicity, respectively (Long and MacDonald 1998; McCready et al. 2006). Consequently, four relative levels of contamination have been created (high, medium high, medium low and low) (McCready et al. 2006).
The objectives of this study were: (1) the use of pollution indicators, such as mC d and geoaccumulation indexes, in order to evaluate the anthropogenic participation in the contamination of surface sediments of Lake Koumoundourou from heavy metals, (2) the use of SQGs, so as to evaluate the pollution status and the adverse biological effects to aquatic organisms due to heavy metals and PAHs in sediments of the lake and of Elefsis bay and (3) the comparison of contaminated sediments from lake Koumoundourou and Elefsis Bay. A more comprehensive assessment of the contamination of an area requires also the knowledge of the speciation of heavy metals, something that will be addressed in another study of ours.
Materials and methods
Study area
Koumoundourou Lake is located inshore of Elefsis Bay (Saronikos Gulf, Greece; Fig. 1), and it is surrounded by many contamination sources, most of which are oil refineries. Moreover, the lake is the main recipient of groundwater leaching from the major landfill of Athens (Karageorgis et al. 2009). The lake is separated from Elefsis Bay by the Athens-Corinth national road, but it is linked to the gulf through a narrow weir and a pipeline. It covers an area of 147,000 m2, and it is approximately 600 m long and 400 m wide. The shoreline perimeter is about 1,700 m and the surface elevation is 1.4 m above sea level (Karageorgis et al. 2009). Water depths do not exceed 3.3 m in the North East part of the lake, while in the South East part, water depths are less than 1 m, thus giving a mean of 1.5 m (Roussakis 2003). Koumoundourou Lake is bordered by oil refinery companies, a marble cutting factory and a military camp used as an oil supply depot station, the latter one located on the east side of the lake (Conides et al. 1996).
Sediment sampling
On May 2011, eight sediment samples labelled as K1–K8 were collected from Lake Koumoundourou. Sediments K1, K3, K5 and K7 were collected from the surface of the east side of the lake, near the military camp and sediments K2, K4, K6 and K8 were collected from the surface of the north side of the lake, near the existing water barrier. Moreover, two samples with the code names E1 and E2 were taken from the surface of Elefsis bay, as shown in Fig. 1. Sampling sites were selected to cover an impacted area based on known anthropogenic sources. All samples were collected using a Zn-plated Petersen grab sampler and were transported to the laboratory at −4 °C. During sampling, the weather conditions were calm winds, shiny, with no rain and ground temperature of approximately 25 °C. The sediments were then slowly air dried at 105 °C, gently homogenised and dry sieved through stainless steel sieves of 2 mm.
Physical properties of sediments
The physical properties of all sediment samples are presented in Table 2. Moisture and organic matter were calculated using method ASTM D2974, specific weight according to method ASTM D854-92 whereas pH and redox potential according to method ASTM D4972 with the use of a Crison pH meter. Cation exchange capacity was calculated according to EPA method 9081. Moreover, an X-ray diffraction technique was applied in order to determine the structure of the sediments. The results showed that the main minerals of all sediments were calcite (50–54 %), quartz (15–29 %) and dolomite (6–14 %) and their classification according to the Unified Soil Classification System was clayey sands for lake’s Koumoundourou sediments and sands for the sediments of Elefsis. For the XRD analysis, a Siemens 5000 refractometer was used.
Determination of heavy metals and PAHs in sediments
The determination of the total concentrations of six heavy metals in all ten sediment samples was done according to EPA method 3051Α for total digestion of the samples and the calculation of the heavy metals concentrations was made with the use of inductively coupled plasma mass spectrometry.
The determination of PAHs in the samples was made according to DIN ISO 11465 for the dry matter and 18287 for SUM PAHs (EPA) by the use of a gas chromatography–mass spectrometry. In accordance with this method, approximately 10 g of air-dried sediment sample (8 h at 35 °C) which is formerly crushed/sieved to/at 2 mm are extracted by 30 ml of acetone/hexane in an ultrasonic bath for 1 h. If it is necessary, a clean-up is conducted using acetonitrile. A secondary PAH solution serves as internal standard.
The results for both heavy metals and fourteen PAHs in all ten sediment samples are shown in Table 3. Because of collecting the samples from three main different geographical areas it was considered necessary to separate the samples into three categories and investigate each one separately, when needed, hence the creation of Table 4.
Results and discussion
Sediment characteristics
In general, all sediment samples have a low cation exchange capacity (CEC) of approximately 4 meq/100 g for the sediments collected from the Lake and around 1.3 meq/100 g for the sediments collected from Elefsis bay. This is totally acceptable since sandy and sandy clay soils with high percentages of quartz and calcite have such low values of CEC (Appelo and Postma 1993). Moreover, sediments collected from the east side of the Lake have lower values of moisture and organic matter than those collected from the north side. All the sediment samples collected from the Lake have similar specific weights, around 4, whereas sediments from the bay have values around 1.
General observations of heavy metals and PAHs in all sediment samples
The total metal content of all the sediments and the total concentrations of PAHs detected in all samples are shown in Table 3. In general, sediments from Elefsis Bay are contaminated in a higher degree than the ones from Lake Koumoundourou, both in heavy metals and PAHs. This can be attributed to several industrial activities in the Elefsis basin and to the point where the sediments were collected. Concentrations of Cr, Cu and Zn in Elefsis sediments are higher than in any sediment taken from the Lake. Furthermore, nickel concentrations are predominant in samples K3 and K7 and generally in higher values than in the sediments come from the north side of the Lake.
As far as PAHs are concerned, it is obvious from Table 3, that phenanthrene, fluorene, fluoranthene and pyrene are abundant in Elefsis sediments and benzo(a)anthracene is present in significant concentrations (regarding its carcinogenic nature) (Anyakora et al. 2003) in all samples from Elefsis bay and in samples collected from the north side of the Lake.
Application of mC d
The modified degree of contamination for all Koumoundourou sediment samples was calculated based on Table 4 and it was found to be 3.05, a number which, according to Abrahim and Parker, classifies the contamination of the lake to “moderate degree of contamination” (Abrahim and Parker 2008). For the calculation of the C fs, the reference concentrations of the selected elements were taken from similar studies done in the surrounding area (Kokovides et al. 1992).
Application of geoaccumulation index
The I geo results are shown in Table 5. Samples from the east side of the Lake present higher values in chromium concentration than the others from the north side, whereas the last have higher concentrations of lead in comparison with the first ones. Samples K3 and K7 showed increased presence of Arsenic but not in an alarming level.
The sediments taken from Elefsis Bay are more contaminated in Cr, Cu, Zn and As compared with the Lake’s sediments, and as far as copper and zinc are concerned can be characterised as “moderately to strongly polluted”, whereas in case of chromium, even “strongly polluted”.
Generally, the sediments can be characterised from “unpolluted” (as far as Ni is concerned) to “moderately polluted” (as far as Cu, Zn and As are concerned). However, taking into consideration that the I geo arbitrarily introduces the constant 1.5 in the equation and the average reference values for the elements are taken without serious consideration in any possible geological variation or the existing background, it is clear that it should not be used as a unique assessment tool for this area (Christophoridis et al. 2009). Especially for nickel, as we shall see in the next chapter of the present study, the application of SQGs indicates that the I geo is rather unreliable, when used alone and only.
Nevertheless, the I geo shows the general tendency of the sediments that is already proved by the mC d.
Application of SQGs
The results from the application of SQGs for the selected heavy metals and PAHs are shown in Fig. 2. Concentrations below detection limit were considered as half of the limit and values for TEL/PEL on the substance dibenzo(a,h)anthracene are not reported, hence the gap on the TEL/PEL diagram of PAHs in Fig. 2.
Regarding heavy metals, as it is seen from Fig. 2 and taking into consideration the mean quotients, PELQ diagram indicates a “medium low to medium high” contamination for the sediments collected from Koumoundourou Lake and “medium high” for the sediments of Elefsis. Therefore, the results from the application of the SQGs for the Lake’s sediments are in absolute agreement with the results obtained from the application of the mC d for these particular sediments. However, we cannot claim the same agreement between the SQGs and the I geo results, as far as nickel concentrations are concerned, since the results of the first show remarkable contamination of nickel unlike the results of the latter that show no contamination at all. This is due to the high reference concentration of nickel taken for the calculation of the I geo in the area of Elefsis and Koumoundourou (Kokovides et al. 1992), in comparison with the concentration values proposed by the SQGs for that particular element (Table 1). Furthermore, the ERMQ diagram, Fig. 2, indicates “medium-low” contamination for Koumoundourou Lake and “medium high” for Elefsis Bay. Moreover, lead is present in significant concentrations in all sediment samples collected from the north side of the lake (all their concentrations exceed the PEL limit value) and in the sediments collected from the Bay. Finally, zinc and copper are also present in noticeable concentrations in the sediments from the Bay with values some of which exceed the PEL limit (concentrations of zinc and copper in E2 sample and copper in E1 sample) and others close to the PEL limit (concentration of zinc in E1 sample).
As far as PAHs are concerned, both ERMQ and PELQ diagrams, shows “low” to “medium-low” contamination for the Lake and “medium-low” to “medium-high” contamination for the bay, with the tendency, sediments collected nearby the water barrier (north side of the Lake) being more contaminated with PAHs than the sediments collected nearby the military camp (east side of the Lake). Moreover, PELQ diagram indicates that the first group of samples (K2, K4, K6 and K8) is in the upper limit of “medium low” contamination whereas the latter group of samples (K1, K3, K5 and K7) is in the lower limit of the same class. The apparent tendency that wants the sediments come from the north side of the Lake being more contaminated with PAHs than the ones come from the east side, can be explained from the existence of the water barrier which acts as a collector of PAHs in that area.
TEL/PEL diagram in Fig. 2, shows clearly that in half of the sediment samples, benzo(a)anthracene is abundant in concentration values higher than the PEL limit which is noteworthy regarding its carcinogenic nature (Anyakora et al. 2003). Furthermore, fluorene, phenanthrene and pyrene are present in significant concentrations but in much lower percentages (20, 30 and 10 %, respectively).
Conclusions
In general, the sediments collected from Elefsis Bay have shown a more elevated content in heavy metals and PAHs compared to those collected from Lake Koumoundourou. Furthermore, samples taken from the north side of the lake are more contaminated with PAHs than the ones taken from the east side. More specific, Cr, Cu and Zn are the predominant elements in sediments collected from Elefsis, whereas Ni is abundant in half of the sediments collected from the east side of the lake, near the military camp. Phenanthrene, fluorene, fluoranthene and pyrene are the main PAHs present in significant concentrations in all Elefsis sediments and benzo(a) anthracene is the predominant one in all samples taken from Elefsis and in all those taken from the north side of the lake, nearby the water barrier.
The application of mC d classifies the contamination of Lake Koumoundourou in “moderate degree” and it is chromium, zinc and lead the elements which mainly contribute to the mC d. Had it been only Cr, the sediments would have been characterized as “highly polluted”.
The I geo is not in total agreement with the other pollution indicators, as far as Ni and Pb are concerned. It classifies the sediments to “unpolluted”, when it comes to nickel and “unpolluted to moderately polluted”, when it comes to Pb. However, the mean I geo (Table 5) shows the tendency of the sediments (“moderately polluted”) which is the same as the one we concluded by the use of mC d.
Regarding heavy metals, the application of SQGs and toxicity mean quotients show that almost 50 % of the sediments are associated with frequent observation of adverse effects, when it comes to Ni and occasional observation of adverse effects, when it comes to Cu, Zn and Pb (judging from the TEL/PEL diagram Fig. 2). No particular tendency was observed between sediments collected from the two different areas of the lake, based on the effect-range approach.
As far as PAHs are concerned, around 60 % of the samples can be occasionally associated to toxic biological effects according to the effect-range classification for phenanthrene, benzo(a)anthracene, chrysene and pyrene. In addition, more than 50 % of the samples in the TEL/PEL diagram (Fig. 2) show concentrations of benzo(a)anthracene higher than the PEL limit value and therefore are frequently associated with adverse effects.
References
Abrahim, G., & Parker, R. (2002). Heavy metal contaminants in Tamaki Estuary: impact of city development and growth, Auckland, New Zealand. Environmental Geology, 42, 883–89.
Abrahim, G., & Parker, R. (2008). Assessment of heavy metal enrichment factors and the degree of contamination in marine sediments from Tamaki Estuary, Auckland, New Zealand. Environmental Monitoring and Assessment, 136, 227–238.
Anagnostou, C., Kaberi, H., Karageorgis A. (1997). Environmental impact on the surface sediments of the bay and the gulf of Thessaloniki (Greece) according to the geoaccumulation index classification. Proceedings of the International Conference on Water Pollution, pp. 269–275
Anyakora, C., Ogbeche, A., Palmer, P., Coker, H., Ukpo, G., & Ogah, C. (2003). GC/MS analysis of polynuclear aromatic hydrocarbons in sediment samples from the Niger Delta region. Chemosphere, 60, 990–997.
Appelo, C. A. J., & Postma, D. (1993). Geochemistry, groundwater and pollution. Rotterdam: A.A. Balkema.
Burton, G. A. (2002). Sediment quality criteria in use around the world. Japanese Society of Limnology, 3, 65–75.
Carman, C. M., Li, X. D., Zhang, G., Wai, O. W. H., & Li, Y. S. (2007). Trace metal distribution in sediments of the Pearl River Estuary and the surrounding coastal area, South China. Environmental Pollution, 147, 311–323.
Chindah, A. C., Braide, A. S., & Sibeudu, O. C. (2004). Distribution of hydrocarbons and heavy metals in sediment and a crustacean (shrimps—Penaeus notialis) from the bonny/new Calabar river estuary, Niger Delta. Ajeam-Ragee, 9, 1–14.
Christophoridis, C., Dedepsidis, D., & Fytianos, K. (2009). Occurrence and distribution of selected heavy metals in the surface sediments of Thermaikos Gulf. N. Greece. Assessment using pollution indicators. Journal of Hazardous Materials, 168, 1082–1091.
Conides, A., Diapoulis, A., & Koussouris, T. (1996). Ecological study of an oil polluted coastal lake ecosystem. Fresenious Environmental Bulletin, 5, 324–332.
Culotta, L., De Stefano, C., Gianguzza, A., Mannino, M. R., & Orecchio, S. (2006). The PAH composition of surface sediments from Stagnone coastal lagoon, Marsala, Italy. Marine Chemistry, 99, 117–127.
Diagomanolin, V., Farhang, M., Ghazi-Khansari, M., & Jafarzadeh, N. (2004). Heavy metals (Ni, Cr, Cu) in the Karoon waterway river. Iran. Toxicology Letters, 151, 63–68.
Fiedler, S., Siebe, C., Herre, A., Roth, B., Cram, S., & Stahr, K. (2009). Contribution of oil industry activities to environmental loads of heavy metals in the Tabasco Lowlands, Mexico. Water Air and Soil Pollution, 197, 35–47.
Giacalone, A., Gianguzza, A., Mannino, M. R., Orecchio, S., & Piazzese, D. (2004). Polycyclic aromatic hydrocarbons in sediments of marine coastal lagoons in Messina, Italy: extraction and GC/MS analysis, distribution and sources. Polycyclic Aromatic Compounds, 24, 135–149.
Giacalone, A., Gianguzza, A., Orecchio, S., Piazzese, D., Dongarrà, G., Sciarrino, S., & Varrica, D. (2005). Metal distribution in the organic and inorganic fractions of soil: a case-study on soils from Sicily. Chemical Speciation and Bioavailbility, 17(3), 83–94.
Hakanson, L. (1980). Ecological risk index for aquatic pollution control, a sedimentological approach. Water Research, 14, 975–1001.
Hinkey, L. M., & Zaidi, B. R. (2007). Differences in SEM-AVS and ERM-ERL predictions of sediment impacts from metals in two US Virgin Islands marinas. Marine Pollution Bulletin, 54, 180–185.
Hogan, C. M. (2009). Heavy metal. In E. Monosson & C. Cleveland (Eds.), Encyclopedia of Earth. Washington, DC: National Council for Science and the Environment.
Karageorgis, A. P., Katsanevakis, S., & Kaberi, H. (2009). Use of enrichment factors for the assessment of heavy metal contamination in the sediments of Koumoundourou Lake. Greece. Water Air and Soil Pollution, 204, 243–258.
Kokovides, K., Loizidou, M., Haralambous, K. J., & Moropoulou, T. (1992). Environmental study of the marinas, Part I. A study on the pollution in the Marinas Area. Environmental Technology, 13, 239–244.
Long, E. R., & MacDonald, D. D. (1998). Recommended uses of empirically derived sediment quality guidelines for marine and estuarine ecosystems. Human and Ecological Risk Assessment, 4, 1019–1039.
McCready, S., Birch, G. F., & Long, E. R. (2006). Metallic and organic contaminants in sediments of Sydney Harbour, Australia and vicinity—a chemical dataset for evaluating sediment quality guidelines. Environment International, 32, 455–465.
Orecchio, S. (2010). Assessment of polycyclic aromatic hydrocarbons (PAHs) in soil of a Natural Reserve (Isola delle Femmine) (Italy) located in front of a plant for the production of cement. Journal of Hazardous Materials, 173(1–3), 358–368.
Orecchio, S., & Papuzza, V. (2009). Levels, fingerprint and daily intake of polycyclic aromatic hydrocarbons (PAHs) in bread baked using wood as fuel. Journal of Hazardous Materials, 164, 876–883.
Ridgway, J., & Shimmield, G. (2002). Estuaries as repositories of historical contamination and their impact on shelf seas. Estuarine Coastal and Shelf Science, 55, 903–928.
Roussakis, G. (2003). Bathymetry of Lake Koumoundourou. In A. Pavlidou (Ed.), Monitoring of the groundwaters, Lake Koumoundourou and the adjacent marine area in relation to the landfill of Western Attica. Technical report, pp. 17–22 (in Greek). Anavyssos: Hellenic Centre for Marine Research
Sklivagou, E., Varnavas, S. P., & Hatzianestis, J. (2001). Aliphatic and polycyclic aromatic hydrocarbons in surface sediments from Elefsis Bay, Greece (Eastern Mediterranean). Toxicological and Environmental Chemistry, 79, 195–210.
Tsai, L. J., Yu, K. C., Chen, S. F., & Kung, P. Y. (2003). Effect of temperature on removal of heavy metals from contaminated river sediments via bioleaching. Water Research, 37, 2449–2457.
Ugochukwu, C. N. C. (2004). Effluent monitoring of an oil servicing company and its impact on the environment. Ajeam-Ragee, 8, 27–30.
Ure, A. M., Quevauviller, Ph, Muntau, H., & Griepink, B. (1993). Speciation of heavy metals in solids and harmonization of extraction techniques undertaken under the auspices of the BCR of the Commission of the European Communities. International Journal of Environmental Analytical Chemistry, 51, 135.
Violintzis, C., Arditsoglou, A., & Voutsa, D. (2009). Elemental composition of suspended particulate matter and sediments in the coastal environment of Thermaikos Bay, Greece: delineating the impact of inland waters and wastewaters. Journal of Hazardous Materials, 166, 1250–1260.
Yaqin, J., Yinchang, F., Jianhui, W., Tan, Z., Zhipeng, B., & Chiqing, D. (2008). Using geoaccumulation index to study source profiles of soil dust in China. Journal of Environmental Sciences, 20, 571–578.
Author information
Authors and Affiliations
Corresponding authors
Rights and permissions
About this article
Cite this article
Hahladakis, J., Smaragdaki, E., Vasilaki, G. et al. Use of Sediment Quality Guidelines and pollution indicators for the assessment of heavy metal and PAH contamination in Greek surficial sea and lake sediments. Environ Monit Assess 185, 2843–2853 (2013). https://doi.org/10.1007/s10661-012-2754-2
Received:
Accepted:
Published:
Issue Date:
DOI: https://doi.org/10.1007/s10661-012-2754-2