Abstract
The total and fraction concentrations of heavy metals (Mn, Cu, Ni, Pb, Co, and Cd) were analyzed in some sediment fractions (Φ2, Φ3, Φ4, Φ5) of selected mangrove ecosystems collected from the Egyptian Red Sea shoreline. The results revealed that manganese had the highest mean value (133 ± 97 mg/kg) followed by copper (49.9 ± 46.0 mg/kg), nickel (28.1 ± 11.8 mg/kg), lead (19 ± 13 mg/kg), cobalt (6.7 ± 4.0 mg/kg), and cadmium (3.327 ± 1.280 mg/kg). The concentrations of heavy metals in the different sediment fractions showed that there was a preferential accumulation of Cu, Co, Mn, and to a lesser degree Cd in the silt and clay fractions rather than in the sand-sized. The sediment quality was performed by using some sediment quality guidelines. Additionally, the contamination and the risk assessment of these heavy metals were achieved by different methods including, potential ecological risk index, contamination factor, pollution load index, and geoaccumulation index. According to the Sediment Quality Guidelines comparisons, the concentrations of Mn and Pb were low and showed no possibility of detrimental effects on the local environment. The levels of Cu and Ni were high, however, could not be considered to present serious threat to the mangrove ecosystem. The data showed that the mangrove ecosystems were affected by the Cd risk.
Similar content being viewed by others
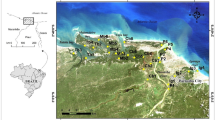
Explore related subjects
Discover the latest articles, news and stories from top researchers in related subjects.Avoid common mistakes on your manuscript.
Introduction
Mangroves are salt-tolerant plants (Shriadah 1999). They have long been recognized as important habitats for fishes, supporting a high diversity of juvenile estuarine and coral reef fish (Dorenborsch et al. 2004; Mumby et al. 2004). The beds of mangroves are important habitats due to the structural complexity, food, shelter, and the protection from predators that they provide (Nagelkerkan et al. 2000). Generally, the communities of mangrove are widely acknowledged to be important elements in coastal ecosystems in the tropics. Moreover, they stabilize mobile sediments and act as a buffer against coastal erosion (Tam and Wong 1996). In order to protect and conserve these fragile mangroves ecosystems, a complete understanding of their environment and the human impacts upon them is essential. However, sediment quality has been recognized as an important indicator of water pollution, since they act as sink of contaminants (Santos Bermejo et al. 2003). This fact converts the sediments in a permanent record of anthropogenic pollutants inputs (Chen et al. 2010). The examination and study of sediment quality can reveal the pollutant variations, degradations and cycles, and their chronic effects on water pollution. In addition, the accumulation of heavy metals in sediments can produce harmful effects on the biota living in them (Harikumar and Nasir 2010; Joksimovic et al. 2011). Since, heavy metals are among the most serious pollutants within the natural environment due to their toxicity, persistence, and bioaccumulation problems (MacFarlane and Burchett 2000); a number of studies were carried out all over the world to assess the levels of different heavy elements in mangrove sediments (Mackey et al. 1992; Rivail et al. 1996; Perdomo et al. 1998; Shriadah 1999; Tam and Wong 2000; Mremi and Machiwa 2003; Defew et al. 2005; Otero et al. 2009; Marchand et al. 2011). Despite the environment significance of mangroves, the scientific efforts on their ecosystems are scarce in Egypt (Okbah et al. 2005; Dar and El-Saharty 2006). The effect of grain size on the adsorption of heavy metals in sediments was studied (Greaney 2005). Bioavailable sediment-bound metals depend, to a significant extent, on the particle size fraction with which a metal is associated (Greaney 2005). Since the metals in this fine-grained fraction are more likely to be biologically available than those in bulk sediments (Bryan and Langston 1992).
The present paper attempts to provide information concerning the levels of some heavy metals (Cd, Co, Cu, Pb, Mn, and Ni) in different sediment fractions (Φ2, Φ3, Φ4, and Φ5) of selected mangrove ecosystems along the Egyptian Red Sea shoreline. Also, the ecological pollution risk assessment of these heavy metals can be predicted.
Area of study
Red Sea is a semi-enclosed narrow water body with no river inputs. It extends for approximately 1,936 km, has a surface area of about 43,970 km2 and attains a depth of nearly 1,800 m. It lies in a very arid zone, where the climate is hot and the rainfall is scarce. The water of the sea is exceptionally clear and the eutrophic zone extends to a depth of about 80–90 m (Shriadah et al. 2004). Red Sea is apparently subjected to the rapid and increasing levels of human activities due to the recreational (swimming and diving), industrial, and fishing activities (Shriadah et al. 2008). Along the Egyptian Red Sea coast, a number of mangrove forests are present. Avicenna marina is the lonely known mangrove species, observed from the western coast of Aqaba Gulf to Hamata, about 400 km southern of Hurghada (Dar and El-Saharty 2006). The present sampling sites (Fig. 1) represent the sediments of six mangrove forests from the tidal zone and the study area extends from the western coast of Aqaba Gulf to Hamata. The northern stations (1 and 2) which lie near the entrance of the Gulf of Aqaba (Fig. 1), receive sediments by outflowing currents coming from the gulf. The source of the sediments along the area from Safaga (station 3) to Hamata (station 6) is largely autochthonous, mainly biogenic or chemical precipitation of different mud facies (Okbah et al. 2005).
Materials and methods
Sampling
Triplicate sediment samples from the upper 5 cm of sediment were collected from six mangrove sites along the Egyptian coast of the Red Sea during 2003. Sampling was carried out using a clean, acid-washed plastic scoop. The three replicate samples were composed and placed in self-sealed acid precleaned plastic bags. All samples were immediately stored in an ice-cooled box and transferred to the laboratory. In the laboratory, the samples were air dried. The dried samples were sieved in the beginning through a 2 mm plastic sieve to remove mangrove leaves and roots, gravel or large particles, visible shells, shell fragments, and pebbles.
Grain size analysis
Grain size analysis was carried out by dry sieving for sand and by pipette technique for silt and clay fractions (Krumbein and Pettijohn 1938) and the data were treated following Folk 1980. For all sediment samples, the separated powdered fractions of similar grain size (Φ2, Φ3, Φ4, Φ5) were oven dried at 70°C to a constant weight and stored in dry acid-washed plastic bags for metal analysis. These fractions were selected according to their almost existence in all studied samples.
Sediment heavy metals analysis
Of dried and fine-ground sediment fraction, 0.25–0.50 g was put into a closed Teflon vessel with 3 ml HNO3, 2 ml HClO4, and 1 ml hydrofluoric acid (HF) acids for total digestion of heavy metals. To obtain high pressure, the vessels were placed inside a well-closed stainless steel block and heated on a thermostatically controlled hot plate at 70°C for about 12 h (Oregioni and Aston 1984). To eliminate HF, the digestion mixture was nearly evaporated with 2 ml H2SO4. The residue was redissolved in 10 ml diluted HNO3 and preserved in acid-clean polyvinyl chloride bottles for analysis. For each digestion program, a blank was prepared using the same procedure. All reagents were of analytical grade and contained very low concentrations of trace metals. All the glassware and the Teflon vessels were previously soaked overnight with 20% HNO3 and then rinsed with metal-free distilled water. Sample solutions and reagent blanks were analyzed for the heavy metals using a Perkin Elmer 2830 flame atomic absorption spectrophotometer. Working standards of studied metals were prepared by diluting concentrated stock solutions (Merck, Germany) of 1,000 mg/l in metal-free distilled water. Each metal concentration was estimated quantitatively according to the standard conditions described in the instrument manual. Duplicate measurements were made for each sample, by direct aspiration into air acetylene flame of the instrument.
Quality assurance
To monitor the quality of chemical analysis and examine the accuracy of the data, sediment reference materials (IAEA-433, International Atomic Energy Agency, Austria) were analyzed with the sediment samples during the course of analysis. The analytical results of the selected metals of interest indicated a good agreement between the reference and analytical values of the reference materials. In addition, the recovery rates for the selected metals from the standard reference material were 93.34–105.49% (Table 1).
Sediment quality
The sediment quality was done using some Sediment Quality Guidelines and potential ecological risk index (RI) (Hakanson 1980; Wang et al. 2011), contamination factor (CF) (Turekian and Wedepohl 1961), pollution load index (PLI) (Tomlinson et al. 1980) and geoaccumulation index (I geo) (Muller 1969) methods.
Potential ecological risk index
With the aim of achieving a broader assessment of heavy metal pollution in the mangrove sediments in terms of ecological risks, Hakanson (1980) developed the following quantitative approach. The potential ecological risk factor of a given contaminant (E i ) is defined as:
where, T i is the toxic response factor for a given substance (i.e., Hg = 40, Cd = 30, As = 10, Pb = Cu = Ni = 5, Cr = 2, Zn = 1), C i represents the metal content in the sediments, and C 0 is the regional background value of heavy metals in the sediments, based on values from relatively nonpolluted bottom sediments (Adami et al. 2000; Adamo et al. 2005).
The sum of the individual E i presents the potential ecological RI for a region and can be expressed as:
According to Hakanson (1980), the following Ei and RI values give an indication of ecological risk:
-
E i < 40, RI < 150 = low potential ecological risk
-
40 ≤ E i < 80, 150 ≤ RI < 300 = moderate potential ecological risk
-
80 ≤ E i < 160, 300 ≤ RI < 600 = considerable potential ecological risk
-
160 ≤ E i < 320, RI ≥ 600 = very high potential ecological risk
-
E i ≥ 320 = dangerous
Contamination factor
The level of contamination of sediment by a metal is often expressed in terms of a contamination factor as follows (Turekian and Wedepohl 1961):
Where CF < 1 refers to low contamination, 1 ≥ CF ≥3 means moderate contamination, 3 ≥ CF ≥6 indicates considerable contamination and CF > 6 indicates very high contamination.
Pollution load index
The extent of pollution by trace metals has been assessed by employing the method based on PLI developed by Tomlinson et al. (1980) and the relation is shown below:
where CF = contamination factor and n = number of metals. PLI provides a simple, comparative means for assessing a site or estuarine quality: a value of 0 indicates perfection, a value of 1 indicates only baseline levels of pollutants present and values above 1 would indicate progressive deterioration of the site and estuarine quality.
Geoaccumulation index
The geoaccumulation index I geo values were calculated for different metals as introduced by Muller (1969) as follows:
where, C n is the measured concentration of element n in the sediment sample and B n is the geochemical background for the element n (Adamo et al. 2005). The factor 1.5 is introduced to include possible variation of the background values that are due to lithogenic variations. Muller (1981) proposed seven grades or classes of the geoaccumulation index. Different geoaccumulation index classes along with the associated sediment quality are given (Table 2).
Statistical analysis
Using the Minitab 13.1 software program, a simple correlation and the hierarchical cluster analyses were done to assess the relationships between various parameters.
Results and discussions
Characteristics of sediments
Table 3 shows that all the studied sediments contain high sand fractions (51.50–79.77%). This indicates that the sand is the dominant component in the collected samples. Additionally, the components of clay and silt fluctuate between 0–24.27% and 6.23–40.62%, respectively. Accordingly, it seems that the sediment type varies along the studied stations. However, these sediments contain varieties of sediment types including clayey sand (station 2), silty sand (stations 1, 3 and 6) and clayey silty sand (stations 4 and 5).
Spatial variation of the metals in the mangrove sediments
The levels of heavy metals in different mangrove fractions in the studied area are presented in Table 4 and Fig. 2. The heavy metals concentration fluctuates not only within different stations but also within different fractions. Mn and Ni are the only heavy metals that show relatively similar distribution trend along the different fractions in the different stations. Generally, no particular trend is observed in the distribution of most metals with respect to the sampling locations. So, for each station, the mean of determined metal concentrations within studied fractions (Φ2, Φ3, Φ4, and Φ5) are calculated and hierarchical cluster analysis is applied for grouping of samples according to their similarities. The obtained cluster analysis dendogram for these means (Fig. 3) reveals that the sediment mangrove communities from stations 1, 3, 5, and 2 differ from those in stations 4 and 6. These variations may be attributed to the effects of biological and physical phenomena, such as tidal inundation, salinity changes, wind, and waves. These phenomena allow the processes of bioturbation, resuspension, and erosion that are known to affect the metal concentrations in surface sediments (Bellucci et al. 2002). A simple correlation analysis was done to clarify the relationships between various metals. Pearson’s correlation coefficient matrix (Table 5) shows that there are significant correlations between the metals of Mn and Ni (r = 0.777, with confidence limit = 100%), Mn and Cu (r = 0.583, with confidence limit = 95%), Mn and Co (r = 0.465, with confidence limit = 95%), and Cu and Co (r = 0.412, with confidence limit = 95%). This means that these metals probably originate from a common source, and are transported and deposited by common carriers (particles).
Grain size and its effect on metal concentration
The average concentration of heavy metals in different fractions (Φ2, Φ3, Φ4, and Φ5) along the studied locations is presented (Fig. 4). The present study reveals that the highest percentages of Mn and Co (38% and 34%, respectively) are recorded in the finest fraction Φ5, nearly doubles those in the greatest fraction Φ2. The percentage of Cu in the finest fraction Φ5 (60%) is considerably higher than that of the other fractions. The greatest fraction Φ2 shows the lowest percentage of Cd (20%), whereas Φ4 accumulates the highest percentage of Cd follows by Φ5. In contrast, Φ2 contains the highest percentage of Pb (36%), follows by the finest fraction Φ5 (27%). However, Ni shows no significant variation with changes in grain size. These results indicate that Cu, Co, Mn, and to a lesser degree Cd increased in concentration with finer size (Φ5). The results suggest that these metals are bound in the silt and clay fractions than the sand-sized fraction of the sediment. Indeed, it is well-established that grain size is one of the important controlling factors in the abundance of trace metals in natural sediments (Horowitz and Elrick 1987). In most sediment, the fine-grained fraction contained higher concentrations of heavy metals than the sand-sized fraction and has been used to examine metal contamination in the whole sediment sample (Tam and Wong 2000). However, the phenomenon of heavy metals sorption by clays was due to the high specific surface area of the smaller particles (Loring 1991; Summers et al. 1996; Ragbirsingh and Norville 2005). Accordingly, this enrichment is probably due to the surface adsorption and the ionic attraction (Horowitz and Elrick 1987). Interestingly, Tam and Wong (2000) designed a study to compare the concentrations of heavy metals bound in the fine-grained fraction (<63 μm) and the sand-sized fraction (2 mm-63 μm) of the sediments. They found that the highest percentage of heavy metals in sediment (43%) was in the fine-grained fraction. They suggested that the concentrations of organic matter in the fine-grained fraction of the sediment were often higher than that in the sand-sized. Regarding Ni and Pb, the preferential accumulation of metals in the silt and clay fractions rather than the sand-sized did not exist in the present study. This is in accordance with Tam and Wong (2000), who stated that the trend of more metal being accumulated in the fine-grained of the sediment may not be universal for all metals and may be varied between metal species.
Comparison between the levels of metals in the studied mangrove sediments with those in the previous studies
Table 6 shows the ranges and the mean concentrations of the present studied metals, some previous studies, and the Sediment Quality Guidelines. According to these data, manganese has the highest mean value (133 ± 97 mg/kg), followed by copper (49.9 ± 46.0 mg/kg), nickel (28.1 ± 11.8 mg/kg), lead (19 ± 13 mg/kg), cobalt (6.7 ± 4.0 mg/kg), and cadmium (3.327 ± 1.280 mg/kg). This trend differs partially than that observed by Defew et al. (2005; Mn > Pb > Ni > Cu > Cd) for mangrove sediments collected from Punta Mala Bay, Panama. Our results of Mn concentrations appear considerably lower than those reported for the contaminated mangrove environments (Perdomo et al. 1998; Ramanathan et al. 1999; Defew et al. 2005). However, the ranges of Cu concentration in the present study are higher than those reported previously (Shriadah 1999; Dar and El-Saharty 2006) and its mean concentration was about 1 order of magnitude higher than the values reported for the clean mangrove sediments of Brazil (Harris and Santos 2000). Our Ni values are lower than those observed for the Arabian Gulf mangrove sediments (Shriadah 1999) and the mean value is close to the levels reported for the contaminated areas (Perdomo et al. 1998; Defew et al. 2005). The Pb values obtained are considerably higher than the literature value of MacFarlane (2002) and similar to the other literature values (Shriadah 1999; Perdomo et al. 1998; Ramanathan et al. 1999). There is a consistency between the ranges of Co in the present study with those obtained earlier for the Arabian Gulf mangrove sediments by Shriadah (1999). The values of Cd in the present study are somewhat comparable with those reported by Shriadah (1999) and Defew et al. (2005) but are higher than those reported by Perdomo et al. (1998) and Dar and El-Saharty (2006).
Sediment quality
According to background values from the Hong Kong Environment Protection Department (clean mangrove environments; Tam and Wong 2000; Table 6), concentrations of Mn and Pb measured in the studied mangrove sediments are low and do not cause hazard to the mangrove system. However, the concentrations of Cu, Ni, and Cd are high and may consider as a high level of contamination to the mangrove ecosystem. The Swedish Environmental Protection Agency stated that the level of Ni contamination is >100 mg/kg (Greaney 2005), which is considerably higher than the levels of Ni in the present study. This agency is responsible for a wide range of issues including pollution control, nature conservation, etc.
The mean concentrations of Cu, Pb, and Cd in the present study are also compared with those of the Canadian Sediment Quality Guidelines. However, these guidelines are used as an interim measure to assess whether the concentrations of heavy metals in sediments could have adverse biological impacts (Table 6). Two levels are generally considered: the threshold effect level (TEL), below which adverse biological effects are expected to occur rarely, and the probable effect level (PEL), above which adverse effects are expected to occur frequently. These concentrations were established by the Canadian Council of Ministers of the Environment, and are routinely used as screening tools by different stakeholders involved in sediment management activities (Canadian Council of Ministers of the Environment 2002). It is observed that the mean concentrations of Cu and Cd are higher than those of the TEL values, whereas the mean concentration of Pb is lower. However, the PEL values are higher than those of Cu, Pb, and Cd. The present results shows that the levels of Cu, Cd, and Ni are high, however, could not be considered to present serious threat to the mangrove ecosystem. It is suggested that any further investigations within the Egyptian mangrove sediments should include organic pollutants and a lot of metals measurements as an aid for better understanding of mangrove ecosystems and, hopefully, to enable us to mitigate any detrimental effects.
For the calculations of potential ecological risk index (Hakanson 1980; Wang et al. 2011), Ni and Pb show low potential ecological risk of E i and RI, values smaller than 40 and 150, respectively (Table 7). On the other hand, Cu gives moderate potential ecological risk along almost the stations, however, E i and RI, values are of values within the ranges 40–80 and 150–300, respectively. Only station 1 is of low potential ecological risk. Generally, Cd shows a dangerous case in all stations sediments; however, E i and RI, values are greater than 320 and 600, respectively (Adami et al. 2000).
Contamination factor and pollution load index calculations are illustrated (Table 8). Mn and Ni heavy metals have moderate contamination values, since their average CF values are of 1.1 and 2.3 values within 1 ≥ CF ≥3 range. Pb and Co show considerable contaminated sediments with values of 3 ≥ CF ≥6. Cu and Cd are of CF > 6 values along all the studied stations. Accordingly, these two heavy metals are dangerously contaminated and their disparate behavior is affected by the anthropogenic sources. PLI values above 1 may indicate the progressive deterioration of these sites (Tomlinson et al. 1980).
The calculated geoaccumulation index values of all the studied metals in mangrove sediments are tabulated (Table 9). The mangrove sediments are of low pollution degree for Mn and Ni heavy metals. The I geo values for Pb and Co heavy metals show moderate pollution in most the sediments samples. Cu gives I geo values of moderate pollution. The I geoCd values show that the mangrove sediments are extremely polluted with Cd. The geoaccumulation index values for Cu and Cd possibly refer to the human activities.
Conclusions
The concentrations of some metals (Mn, Cu, Ni, Pb, Co, and Cd) were determined totally and fractionally (Φ2, Φ3, Φ4, and Φ5) in mangrove sediments selected from some ecosystems along the Egyptian Red Sea shoreline. Cu, Co, Mn, and Cd heavy metals were bounded in the silt and clay fractions than the sand-sized fraction of the sediment. Based on the average values, the concentrations of Mn and Pb were low and showed no possibility of detrimental effects on the local environment. On the other hand, the levels of the other metals (Cu and Ni) were high and their concentrations may not make any deleterious or harmful effects to the mangrove system. Among the studied heavy metals, Cd was the only heavy metal that may cause risk to the ecological systems. Accordingly, the heavy metals must be monitored to ensure their harmless levels along the mangrove ecosystems.
References
Adami, G., Barbieri, P., & Reisenhofer, E. (2000). An improved index for monitoring metal pollutants in surface sediments. Toxicological and Environmental Chemistry, 77(3–4), 189–197.
Adamo, P., Arienzo, M., Imperato, M., Naimo, D., Nardi, G., & Stanzione, D. (2005). Distribution and partition of heavy metals in surface and sub-surface sediments of Naples city port. Chemosphere, 61, 800–809.
Bellucci, L. G., Frignani, M., Paolucci, D., & Ravanelli, M. (2002). Distribution of heavy metals in sediments of the Venice lagoon: the role of the industrial area. The Science of the Total Environment, 295, 35–49.
Bryan, G. W., & Langston, R. J. (1992). Bioavailability, accumulation and effects of heavy metals in sediments, with special reference to United Kingdom estuaries: a review. Environmental Pollution, 76, 89–131.
Canadian Council of Ministers of the Environment (2002). Canadian Sediment Quality Guidelines for the protection of aquatic life: summary tables. Updated. In: Canadian environmental quality guidelines, 1999, Canadian Council of Ministers of the Environment, Winnipeg
Chen, T.-R., Yu, K.-F., Li, S., Price, G. J., Shi, Q., & Wei, G.-J. (2010). Heavy metal pollution recorded in Porites corals from Daya Bay, northern South China Sea. Marine Environmental Research, 70, 318–326.
Dar, M. A., & El-Saharty, A. A. (2006). Recycling and retention of some trace metals in the mangrove sediments, Red Sea, Egypt. Egyptian Journal of Aquatic Research, 32(2), 34–47.
Defew, L. H., Mair, J. M., & Guzman, H. M. (2005). An assessment of metal contamination in mangrove sediments and leaves from Punta Mala Bay, Pacific Panama. Marine Pollution Bulletin, 50, 547–552.
Dorenborsch, M., van Riel, M. C., Nagelkerken, I., & van der Velde, G. (2004). The relationship of reef fish densities to the proximity of mangrove and seagrass nurseries. Estuarine, Coastal and Shelf Science, 60, 37–48.
Folk, R. L. (1980). Petrology of sedimentary rocks (p. 182). Austin: Hemphill.
Greaney, K. M. (2005). An assessment of heavy metal contamination in the marine sediments of Las Perlas Archipelago, Gulf of Panama (p. 109). M.Sc. thesis. Edinburgh: School of Life Sciences, Heriot-Watt University.
Hakanson, L. (1980). An ecological risk index for aquatic pollution control: a sedimentological approach. Water Research, 14, 975–1001.
Harikumar, P. S., & Nasir, U. P. (2010). Ecotoxicological impact assessment of heavy metals in core sediments of a tropical estuary. Ecotoxicology and Environmental Safety, 73, 1742–1747.
Harris, R. R., & Santos, M. C. F. (2000). Heavy metal contamination and physiological variability in the Brazilian mangrove crabs, Ucides cordatus and Callinectes danoe (Crustacea: Decapoda). Marine Biology, 137, 691–703.
Horowitz, A. J., & Elrick, K. A. (1987). The relation of stream sediment surface area, grain size and composition to trace element chemistry. Applied Geochemistry, 2(4), 437–451.
Joksimovic, D., Tomic, I., Stankovic, A. R., Jovic, M., & Stankovic, S. (2011). Trace metal concentrations in Mediterranean blue mussel and surface sediments and evaluation of the mussels quality and possible risks of high human consumption. Food Chemistry, 127, 632–637.
Krumbein, W. C., & Pettijohn, F. G. (1938). Manual of sedimentary petrology (p. 549). Appleton Century Crofts: New York.
Loring, D. H. (1991). Normalisation of heavy metal data from estuarine and coastal sediments. ICES Journal of Marine Science, 48, 101–115.
MacFarlane, G. R. (2002). Leaf biochemical parameters in Avicennia marina (Forsk) Vierh as potential biomarkers of heavy metal stress in estuarine ecosystems. Marine Pollution Bulletin, 44, 244–256.
MacFarlane, G. R., & Burchett, M. D. (2000). Cellular distribution of Cu, Pb and Zn in the grey mangrove Avicennia marina (Forsk) Vierh. Aquatic Botany, 68, 45–59.
Mackey, A. P., Hodgkionson, M., & Nardella, R. (1992). Nutrient levels and heavy metals in mangrove sediments from the Brisbane River, Australia. Marine Pollution Bulletin, 24(8), 418–420.
Marchand, C., Allenbach, M., & Lallier-Vergès, E. (2011). Relationships between heavy metals distribution and organic matter cycling in mangrove sediments (Conception Bay, New Caledonia). Geoderma, 160, 444–456.
Mremi, S. D., & Machiwa, J. F. (2003). Heavy metal contamination of mangrove sediments and the associated biota in Dares Salaam, Tanzania. Tanzania Journal of Science, 29(1), 61–76.
Muller, G. (1969). Index of geoaccumulation in sediments of the Rhine River. GeoJournal, 2(3), 108–118.
Muller, G. (1981). The heavy metal pollution of the sediments of Neckars and its tributary: a stocktaking. Chemiker-Zeitung, 105, 157–164.
Mumby, P. J., Edwards, A. J., Arias-Gonzalez, J. E., Lindeman, K. C., Blackwell, P. G., Gall, A., Gorczynska, M. I., Harborne, A. R., Pescod, C. L., Renken, H., Wabnitz, C. C. C., & Liewellyn, G. (2004). Mangroves enhance the biomass of coral reef fish communities in the Caribbean. Nature, 427, 533–536.
Nagelkerkan, I., Dorenbosch, M., Verberk, W. C. E. P., Cocheret de la Moriniere, E., & van der Velde, G. (2000). Day-night shifts of fishes between shallow-water biotopes of a Caribbean bay, with emphasis on the nocturnal feeding of Haemulidae and lutjanidae. Marine Ecology Progress Series, 194, 55–64.
Okbah, M. A., Shata, M. A., & Shriadah, M. M. A. (2005). Geochemical forms of trace metals in mangrove sediments—Red Sea (Egypt). Chemistry and Ecology, 21(1), 23–36.
Oregioni, B., & Aston, S. R. (1984). IAEA Monaco Laboratory, Internal Report (Cited from Reference Methods in Pollution Studies No.38, UNEP, 1986).
Otero, X. L., Ferreira, T. O., Huerta-Díaz, M. A., Partiti, C. S. M., Souza, V., Jr., Vidal-Torrado, P., & Macías, F. (2009). Geochemistry of iron and manganese in soils and sediments of a mangrove system, Island of Pai Matos (Cananeia, SP, Brazil). Geoderma, 148(3–4), 318–335.
Perdomo, L., Ensminger, I., Espinos, L. F., Elsters, C., Wallner-Kersanach, M., & Schnetters, M. L. (1998). The mangrove ecosystem of the Ciénaga Grande de Santa Marta (Colombia): observations on regeneration and trace metals in sediment. Marine Pollution Bulletin, 37, 393–403.
Ragbirsingh, Y., & Norville, W. (May 2005). A geographic information system (GIS) analysis for trace metal assessment of sediments in the Gulf of Paria, Trinidad. Revista de Biologia Tropical, 53(Suppl. 1), 195–206.
Ramanathan, A. L., Subramanian, V., Ramesh, R., Chidambaram, S., & James, A. (1999). Environmental geochemistry of the Pichavaram mangrove ecosystem (tropical), southeast coast of India. Environmental Geology, 37(3), 223–233.
Rivail, D. M., Lamotte, M., Donard, O. F. X., Soriano-Sierra, E. J., & Robert, M. (1996). Metal contamination in surface sediments of mangroves lagoons and southern Bay in Florianopolis Island. Environmental Technology, 17(10), 1035–1046.
Santos Bermejo, J. C., Beltrán, R., & Gómez Ariza, J. L. (2003). Spatial variations of heavy metals contamination in sediments from Odiel River (Southwest Spain). Environment International, 29, 69–77.
Shriadah, M. M. A. (1999). Heavy metals in Mangrove Sediments of the United Arab Emirates Shoreline (Arabian Gulf). Water, Air, and Soil Pollution, 116, 523–534.
Shriadah, M. M. A., Okbah, M. A., & El-Deek, M. S. (2004). Trace metals in the water columns of the Red Sea and the Gulf of Aqaba, Egypt. Water, Air, and Soil Pollution, 153, 115.
Shriadah, M. A., Said, T. O., El-Zokm, G., & Masoud, M. S. (2008). Physico-chemical characteristics of the surficial sediments along the Egyptian Red Sea coasts. Egyptian Journal of Aquatic Research, 34(2), 83–95.
Summers, J. K., Wade, T. L., Engle, V. D., & Malaeb, Z. A. (1996). Normalization of metal concentrations in estuarine sediments from the Gulf of Mexico. Estuaries, 19, 581–594.
Tam, N. F. Y., & Wong, Y. S. (1996). Retention and distribution of heavy metals in mangrove soils receiving wastewater. Environmental Pollution, 94(3), 283–291.
Tam, N. F. Y., & Wong, W. S. (2000). Spatial variation of heavy metals in surface sediments of Hong Kong mangrove swamps. Environmental Pollution, 110, 195–205.
Tomlinson, D. C., Wilson, J. G., Harris, C. R., & Jeffrey, D. W. (1980). Problems in the assessment of heavy-metal levels in estuaries and the formation of a pollution index. Helgoland Marine Research, 33, 566–575.
Turekian, K. K., & Wedepohl, K. H. (1961). Distribution of the elements in some major units of the earth’s crust. Bulletin Geological Society of America, 72, 175–192.
Wang, Y., Yang, Z., Shen, Z., Tang, Z., Niu, J., & Gao, F. (2011). Assessment of heavy metals in sediments from a typical catchment of the Yangtze River, China. Environmental Monitoring and Assessment, 172, 407–417.
Author information
Authors and Affiliations
Corresponding author
Rights and permissions
About this article
Cite this article
El-Said, G.F., Youssef, D.H. Ecotoxicological impact assessment of some heavy metals and their distribution in some fractions of mangrove sediments from Red Sea, Egypt. Environ Monit Assess 185, 393–404 (2013). https://doi.org/10.1007/s10661-012-2561-9
Received:
Accepted:
Published:
Issue Date:
DOI: https://doi.org/10.1007/s10661-012-2561-9