Abstract
Tomato is challenged by several pathogens which cause loss of production. One such pathogen is the oomycete Phytophthora infestans which is able to attack all the aerial parts of the plant. Although a wide range of resistance sources are available, genetic control of this disease is not yet successful. Pyramiding R-genes through genetic transformation could be a straightforward way to produce tomato and potato lines carrying durable resistance to P. infestans. In this work the R1 potato gene was transferred into tomato lines. The tomato transgenic lines were analyzed by using q-RT-PCR and progeny segregation to determine the gene copy number. To test the hypothesis that R1 represents a specifically regulated R-gene, transgenic tomato plants were inoculated with P. infestans isolate 88133 and IPO. All the plants containing the R1 gene were resistant to the late blight isolate IPO-0 and susceptible to isolate 88133. These results provide evidence for specific activation of the R1 gene during pathogen challenge. Furthermore, evidence for enhancement of PR-1 gene expression during P. infestans resistance response was obtained.
Similar content being viewed by others
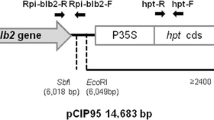
Avoid common mistakes on your manuscript.
Introduction
Late blight caused by Phytophthora infestans is a major disease in the Solanaceae family, attacking leaves, stems, and fruits of tomato (Rubin et al. 2001). Disease may be initiated by air-borne sporangia or by oospores harboured in the soil (Rubin and Cohen 2004). Within-season spread occurs via sporangia. Following the attack of this oomycete, the production of tomato fruits is seriously compromised; hence the pathogen is controlled with chemicals, the effect of which is reduced by selection for fungicide-resistant P. infestans isolates (Fry and Goodwin 1997).
For these reasons, much effort in recent years has been applied to discover resistance genes (R-genes) conferring complete and broad-spectrum resistance against P. infestans in the Solanum genus. Four sources of resistance to late blight have been discovered in tomato wild relatives and transferred into tomato breeding lines: Ph-1, located on chromosome 7; Ph-2 located on chromosome 10 (Moreau et al. 1998); Ph-3 located on chromosome 9 (Chunwongse et al. 2002) and Ph-5 (Foolad et al. 2008). Additional studies were performed to discover tomato QTLs giving resistance to P. infestans (Foolad et al. 2008). Despite the wide range of resistance sources available, genetic control has not yet been successful due to the partial resistance conferred by such genes and to the rapid evolution of new pathogen strains which are able to overcome the R-genes.
Extensive studies have been performed within potato germplasm, and cultivars resistant to different isolates as well as broad-resistance cultivars have been released. Several of the R-genes in question have been cloned and characterized in potato (Hein et al. 2009). The first was the R1 gene originated from S. demissum (Ballvora et al. 2002). The R1 resistance gene is a member of the leucine zipper/NBS/LRR class of plant resistance genes and is located on potato chromosome V in a cluster of several R1 homologues (Ballvora et al. 2002). A QTL is mapped in the neighbourhood of the R1 cluster and it confers broad resistance to P. infestans (Ballvora et al. 2007). The co-localization of R-QTLs and R-genes is frequently observed (Gebhardt and Valkonen 2001; Timmerman-Vaughan et al. 2001; Bai et al. 2003). Some evidence shows that, in potato, the R1 gene might act synergistically with the QTL enhancing the durability of resistance (Ercolano et al. 2004; Ballvora et al. 2007). Pyramiding R-genes by conventional breeding is laborious, time-consuming and sometimes impossible. Making use of transgenic plants could be a straightforward approach to achieve durable resistance to P. infestans.
Pathogenesis-related (PR) proteins are reported to be important players in the P. infestans defence response (Hein et al. 2009). Several plant PR proteins are involved in potentially important activities in defence mechanisms through both direct degradation of the pathogen mycelia wall (Hoegen et al. 2002) and during effector-triggered immunity (Jones and Dangl 2006). Assessment of their expression during the infection process can be useful to understand the resistance mechanism activated by the R1 gene and to elucidate reasons for the establishment of an incompatible plant pathogen interaction.
In this work, we transformed tomato plants with the potato R1 gene and in attempting to identify its functionality we verified the correct genomic introgression and expression. We then assessed the primary transformants (T0) copy number by using a relative Real Time PCR. Finally, we tested whether R1 gene confers resistance to P. infestans isolate IPO-0 in tomato and if it is able to activate a specific PR-1 gene reported as expressed during effector-receptor interaction.
Material and methods
Plant materials
Solanum lycopersicum cv Moneymaker and cv San Marzano seeds were used for transformation experiments. Seeds were placed in 50 ml Falcon tubes and were then rinsed, twice in 70% ethanol, for 5 min and 1 min respectively. The solution was then decanted from the tubes. The seeds were washed in 10% of commercial NaOCl (4.95 active chlorides) with 0.1% of SDS twice for 10 min, and then rinsed five times in sterile dd water. The seeds were germinated in a Magenta box containing a germination medium (GM). Approximately 20–30 seeds were placed in each box. The cultures were placed at 27 ± 1°C under a 16 h photoperiod for 10 days.
Plant expression vector, tomato transformation and plant regeneration
Agrobacterium tumefaciens strain LBA4404, containing in a recombinant plasmid pCLD04541 (Tao and Zhang 1998) the potato genomic clone 10-2, was used to generate transgenic tomato plants in this study. This clone is a 10 kb fragment of the potato genome carrying the R1 gene (provided by Dr. Christiane Gebhardt MPZI, German). According to the procedure described by Park et al. (2003) with some modifications, A. tumefaciens was grown in YEP liquid medium (Table 1), supplemented with tetracycline, streptomycin and spectinomycin, at 28°C for approximately 3 days in the dark. One day before inoculation, 1 ml of pre-inoculums cells was inoculated in 50 ml of AB medium (Table 1) and the flask was shaken overnight at 28°C at the speed of approximately 200 rpm. The bacterial cells were collected by centrifugation at 1500 g for 5 min and then resuspended in AB medium up to a final OD 600 of 0.8 for infection.
Cotyledons were sectioned into halves. The end of each cotyledon was cut off to allow it to adsorb the bacterial suspension. The explants were dipped in the bacterial suspension for 10 min and blotted on a sterilized paper towel placed on CM medium. The explants were placed with the upper leaf surface in contact with the medium. The plates were closed with parafilm, wrapped with aluminium foil and incubated for 2 days at 25°C. The explants were then grown on RM medium to induce callus formation. Every 2 weeks, the explants were propagated onto RM selection medium. When a stem developed a leaf, the stem was cut off at the maximum possible length and transferred to rooting medium (RTM). The plants were propagated every 4 weeks on MS30 supplied with kanamycin and carbacillin. Composition of the media used is reported in Table 2.
Molecular detection of transgenic plants by polymerase chain reaction
The presence of the construct was verified by PCR amplification. Genomic DNA was isolated according to the procedure of DNeasy-Kit (Qiagen, Hilden, Germany). PCR was carried out by using specific primer for the NPTII and R1 genes (Table 3). The amplification was performed under the following conditions: 94°C for 5 min, 94°C for 1 min, 60°C for 1 min, and 72°C for 1.5 min for 40 cycles and 72°C for 7 min. Finally, the PCR products were separated on a 1% agarose gel, and visualized by ethidium bromide staining.
RNA isolation and retrotranscription PCR analysis
Total RNA was extracted from tomato leaves, using RNeasy-Kit (Qiagen, Hilden, Germany), according to the manufacturer’s instructions. One microgram of total RNA was reverse-transcribed using 0.5 μg of oligo (dT) and 200 units of SuperScript III (Invitrogen, USA). The specific primers 76-2sf2 and 76-2SR (Table 3), were used for gene amplification. One μl of the retrotranscription reaction and 10 pmol of each oligonucleotide primer were used for RT-PCR, in a total volume of 25 μl. The PCR conditions used were as follows: 94°C for 1 min, 60°C for 1 min, and 72°C for 1.5 min for 40 cycles, and 72°C for 7 min and 94°C for 5 min after 40 cycles of 94°C for 1 min, 60°C for 1 min, and 72°C for 1.5 min, followed by 7 min of final extension at 72°C. The amplified products were electrophoresed on a 1.5% agarose gel and visualized by ethidium bromide staining.
Transgene detection by q-RT-PCR
Genomic DNA was used in q-RT-PCR experiments. The q-RT-PCR reactions were performed by iCycler (Bio-Rad, Richmond, CA, U.S.A.) using the SYBR Green approach as a fluorescence reporter and hot-start Taq polymerase iQ SYBR Green (Bio-Rad, Richmond, CA, U.S.A.). Reactions were performed in triplicate using the following conditions: one cycle of 95°C for 10 min and 35 cycles of 95°C for 20 s, 60°C for 30 s. cDNA concentrations were normalized based on primers designed for Elongation Factor 1 (EF-1) (Nicot et al. 2005) genomic DNA (Table 3). The software iQ5 (Bio-Rad, Richmond, CA, U.S.A.) was used to determine the fold between the endogenous and target sequences. Two DNA controls with respectively one and two copies NPTII gene number were used in the analysis. Transgenic DNAs containing one copy and two copy NPTII gene were kindly provided by Dr. M Tucci IGV-CNR Portici (Italy) and copy number was assessed by Southern blot analysis (Data not shown).
cDNA q-RT-PCR
cDNA of T1 L5 plants was used to perform q-RT-PCR amplifications with PR primers (Table 3). Reactions were performed in triplicate using the following conditions: one cycle of 95°C for 10 min and 35 cycles of 95°C for 20 s and 60°C for 30 s. cDNA concentrations were normalized based on primers for the Elongation Factor 1 (EF-1) (Nicot et al. 2005). The software iQ5 (Bio-Rad, Richmond, CA, U.S.A.) was used to determine the fold between the endogenous and target sequences.
Pathogen inoculation
P. infestans isolate IPO (race 0) and isolate 88133 (race 1,3,5,7,11), kindly provided by Dr Francine Govers, Wageningen University, The Netherlands, were grown in the dark at 26°C on V8 juice agar medium (200 ml V8 juice, 3 g CaCO3, 800 ml DW, 15 g agar, pH 6.4). For the leaf infection assay, fully expanded leaves of 5- or 6-week-old plants were used for P. infestans inoculation. An oomycete plug (AGAR disc) of a five-day-old culture (0.4 mm in diameter) was placed at the center of the abaxial leaf surface. The inoculated plants were cultured in a growth chamber at 25°C and 100% humidity. Finally, three expanded leaves from three different plants of each T0 plant or T1 population were inoculated. The presence or absence of a hypersensitive response (HR) was scored after 8–10 days post-inoculation. Each experiment was replicated at least twice.
Statistical test
Statistical analysis, performed by GeneStat software q-RT-PCR, both at the genomic and cDNA level, was made with ANOVA and Tukey’s test at 95% of significance. The chi-square test at P ≥ 0.5 was used to analyze the segregation ratio of four independent T1 populations (n = 30).
Results
Tomato transformation
To generate transgenic tomatoes expressing R1, we used a Agrobacterium tumefaciens strain containing the clone 10-2, that has been demonstrated to be necessary and sufficient to transfer the HR response upon infection with P. infestans to the susceptible potato cultivar Desiree (Ballvora et al. 2002). Susceptible tomato Moneymaker and San Marzano cultivars were used in transformation experiments. In all, 190 explants were co-cultivated by Agrobacterium tumefaciens clone LBA4404/10-2. Thirty-two (17%) shoots were regenerated after 8 weeks of culture on RM selection medium containing 50 mg/l kanamycin. Generally, single shoots were produced from each explant. These 32 regenerated plants were finally transferred onto rooting medium (RTM) with carbanicillin. Twelve (6.3%) plants were rooted on the RTM. These shoots were propagated about five times on the rooting medium to suppress A. tumefaciens infection. Growing on rooting medium containing the antibiotic, the shoots were analyzed at molecular level to detect the stable integration of the R1 gene by PCR and RT-PCR.
Molecular analysis of the transgenic plants
Twelve plants were analyzed by using NPTII and R1 specific primers and all of them showed the presence of the heterologue gene (Fig. 1). Further RT-PCR analysis confirmed the transcription of the R1 gene in the tomato plants. PCR analysis conducted with specific R1 primers showed that the gene was introgressed and transcribed in the same way between potato and tomato (Fig. 2). The evidence is confirmed by the results of retrotranscription analysis: the R1 primer pair was designed in a region spanning an intron which produced an amplicon 200 bp smaller than the cDNA amplicon with the gDNA. The results confirm the correct splicing of the gene in tomato (Fig. 2a, b). All the plants were propagated and transferred into soil pots. The plants were grown in a greenhouse and all produced progeny.
Assessment of gene copy number by using q-RT-PCR and progeny segregation analysis
The real time PCR approach on gDNA, in combination with segregation analysis, was used to determine the gene copy number in tomato transformed plants. The EF-1 gene was used as a reference sequence for q-RT-PCR analysis. Although the introgressed gene was the R1 gene, in order to detect the transgene copy number, specific primers for the reporter NPTII gene were used. By using the reporter gene as a target, we avoided aspecific amplification due to the presence of R1 homologue genes in the tomato genome. Primary transformants were used in q-RT-PCR experiments. Two control plants, respectively carrying one copy and two copies for the NPTII target gene, were included in the analysis. The gDNA target gene was quantified as 0.5 times less than the endogenous control EF-1 that is present as a double copy in the tomato genome (Fig. 3). Tukey’s test revealed significant differences between the two-copy control and the other assessed samples (Fig. 3). In order to confirm the gene copy number evaluated by q-RT-PCR, four different progenies from the single L5, L16, L18 and L23 primary transformants were investigated by PCR molecular analysis using specific NTPII and R1 primers. The segregation ratio was analyzed by chi-square test; data were in accordance with a Mendelian segregation ratio of 3:1 reported for a single gene (p > 0.05) (Table 4).
q-RT-PCR on the primary transformants T0 compared with the “C” one-copy NPTII control (violet bar) and “C” two-copy NPTII control (red bar). The letters bar showed Tukey’s test calculated from three different replicas. Amplification of T0 plants showed no significant differences with the one-copy control (black bar) but differences with the two-copy control (white bar) and the non-transformed Moneymaker (MM)
Disease evaluation in R1 transgenic plants
To determine whether or not the R1 transgene confers resistance to late blight isolate IPO-0, tests were carried out on seven T0 plants and fifty T1 plants, derived from different T0 individuals (Table 4). Completely expanded leaves of primary transgenic tomato plants were inoculated by using the agar plug method with IPO-0 and 88133 pathogen isolates. Sixty-three independent leaves of tomato, from seven different tomato transformed plants, were tested and the disease symptoms evaluated. Nine days after inoculation, prominent necrotic areas with late blight symptoms appeared on the leaves inoculated with isolate 88133 and in non-transformed plants, whereas a hypersensitive reaction developed in all transgenic lines inoculated with isolate IPO-0 of P. infestans (Fig. 4). An additional disease test was conducted using zoospores of virulent and avirulent P. infestans isolates, confirming the previous results. Disease tests were also performed on the full L5 and L16T1 segregating populations and selected individuals of L8 and L23 populations. All the plants carrying the R1 gene showed the resistant phenotype after 9 days, confirming molecular results (Table 4).
Leaves of three L5 inoculated individuals carrying R1 were collected 0, 3 and 6 h after inoculation (hpi) to conduct expression analysis during infection. The PR-1 gene was chosen as a marker gene to evaluate P. infestans infection in the resistant and susceptible pools of plants. The PR-1 expression level, quantified by q-RT-PCR, showed a high transcription level already 3 h after inoculation in the resistant plant-pathogen interaction that increased after 6 hpi, while in the susceptible plants the same amount of transcription was revealed at two time points (Fig. 5).
Discussion
Wild Solanum species represent an important source of resistance against diseases and pests in tomato. However, introduction of such resistance genes to commercial cultivars of S. lycopersicum by conventional breeding techniques often encounters serious difficulties due to high incompatibility barriers to hybridization (Bhatia et al. 2004). Cisgenic (Schouten et al. 2006) and/or transgenic approaches can be useful to overcome problems of incompatibility between species, extending the pool of genes of available for this crop.
In the present study, tomato plants were generated, carrying the heterologue potato R1 gene. The putative transformants were selected in the presence of kanamycin, and the integration of the transgene into the host genomes was confirmed by molecular analyses. We then developed a sensitive and high-throughput q-RT-PCR technique for rapidly estimating the transgene copy number in our material. Indeed, the number of the transgene copy could greatly influence the expression level and genetic stability of the target gene (Yang et al. 2005).
Based on our approach, all transformed plants showed an amount of transgenic gDNA comparable with the one copy NPTII control providing evidence for a single gene copy insertion. Estimation of transgene copy number based on Southern analysis is laborious and time-consuming, may involve hazardous radioisotopes, and requires relatively large amounts of plant materials. The q-RT-PCR strategy has already proved useful for quantifying the transgene copy number (Ingham et al. 2001; Batista et al. 2008). However, in previous works a single copy gene was selected as reference gene in a relative q-RT-PCR (German et al. 2003; Prior et al. 2006). Bradeen et al. (2009) showed a high correlation between the transgene quantification obtained using both a single copy gene (Urease) and a double copy gene (EF-1). Here we have reported the combined use of the tomato EF-1 endogenous gene with NTPII quantified genes, as reference sources, for accurate measurement of the number of introgressed genes. To confirm our results, molecular and phenotypic segregation analysis was conducted on two progenies derived from different T0 as reported elsewhere (Bubner and Baldwin 2004).
To test the hypothesis that R1 represents a specifically regulated R-gene, transgenic tomato plants were inoculated with P. infestans isolates 88133 and IPO-0. All the T0 and T1 plants containing the R1 gene were resistant to the late blight isolate IPO-0 and susceptible to isolate 88133. These results provide evidence for specific activation of the R1 gene during pathogen attack also in tomato. In Solanaceae, several R-genes isolated from a species have proved functional in sexually incompatible species. Interestingly, Van der Vossen et al. (2005) reported a functional complementation of resistance gene Rpi-blb2 against P. Infestans isolated from wild potato species in susceptible tomato. The Bs2 gene, which encodes Xanthomonas resistance in pepper, has been transferred into tomato and shown to be functional (Tai et al. 1999). The N gene isolated in tobacco is active also in tomato (Whitham et al. 1996); the tomato Sw-5 resistance gene was transferred into the tobacco susceptible plant proving its ability to confer resistance to tospoviruses (Spassova et al. 2001).
Analysis of the PR proteins expression and of molecules triggered by the HR response in the plant cell programmed death could be useful to better investigate resistance process. In our study the Pr-1 gene was analyzed during the first hours post inoculation in the compatible and incompatible interaction. Our results suggest an activation of the PR-1 gene in R1 incompatible reaction at a very early stage. Indeed, a PR-1 expression increasing of one fold between 3 hpi and 6 hpi in incompatible interaction was displayed. Other data provided evidence of PR-1 activation in the early defence stages. In particular, Ronning et al. (2003), reported PR-1 activation during the first 12 h after inoculation. The Pr-proteins, particularly PR-1 and PR-5, are reported as important components of the defence mechanism of potato against P. infestans (Wang et al. 2006). PR genes induced in this interaction have been described as “pathogen response” genes (Smart et al. 2003). Indeed, van Loon et al. (2006) demonstrated that, in tomato, PR-1 was able to reduce sporangia germination and germ-tube length.
In our work we generated several tomato transgenic R1 lines and estimated gene copy number by the real time PCR method using an endogenous tomato sequence which is not in a single gene copy. Furthermore, we provided evidence that the PR-1 gene is expressed specifically during pathogen challenge also in tomato, as a defence response upon infection with P. infestans isolate 88133 is induced. Finally, we recognized enhancement of PR-1 gene expression during the P. infestans resistance response. Future investigations will seek to elucidate the pattern of defence genes activated during R1 compatible and incompatible reactions.
References
Bai, Y. L., Huang, C. C., van der Hulst, R., Meijer-Dekens, F., Bonnema, G., & Lindhout, P. (2003). QTLs for tomato powdery mildew resistance (Oidium lycopersici) in Lycopersicon parviflorum G1.1601 co-localize with two qualitative powdery mildew resistance genes. Molecular Plant-Microbe Interactions, 16, 169–176.
Ballvora, A., Ercolano, M. R., Weiss, J., Meksem, K., Bormann, C. A., Oberhagemann, P., et al. (2002). The R1 gene for potato resistance to late blight (Phytophthora infestans) belongs to the leucine zipper/NBS/LRR class of plant resistance genes. The Plant Journal, 30, 361–371.
Ballvora, A., Jocker, A., Viehover, P., Ishihara, H., Paal, J., Meksem, K., et al. (2007). Comparative sequence analysis of Solanum and Arabidopsis in a hot spot for pathogen resistance on potato chromosome V reveals a patchwork of conserved and rapidly evolving genome segments. BMC Genomics, 8, 112.
Batista, D., Fonseca, S., Serrazina, S., Figueiredo, A., & Pais, M. S. (2008). Efficient and stable transformation of hop (Humulus lupulus L.) var. Eroica by particle bombardment. Plant Cell Reports, 27, 1185–1196.
Bhatia, P., Ashwath, N., Senaratna, T., & Midmore, D. (2004). Tissue culture studies of tomato (Lycopersicon esculentum). Plant Cell, Tissue and Organ Culture, 78, 1–21.
Bradeen, J. M., Iorizzo, M., Mollov, D. S., Raasch, J., Kramer, L., Millett, B. P., et al. (2009). Higher copy numbers of the potato RB transgene correspond to enhanced transcript and late blight resistance levels. Molecular Plant-Microbe Interactions, 22, 437–446.
Bubner, B., & Baldwin, I. T. (2004). Use of real-time PCR for determining copy number and zygosity in transgenic plants. Plant Cell Reports, 23, 263–271.
Chunwongse, J., Chunwongse, C., Black, L., & Hanson, P. (2002). Molecular mapping of the Ph-3 gene for late blight resistance in tomato. Journal of Horticultural Science & Biotechnology, 77, 281–286.
Ercolano, M. R., Ballvora, A., Paal, J., Steinbiss, H. H., Salamini, F., & Gebhardt, C. (2004). Functional complementation analysis in potato via biolistic transformation with BAC large DNA fragments. Molecular Breeding, 13, 15–22.
Foolad, M. R., Merk, H. L., & Ashrafi, H. (2008). Genetics, genomics and breeding of late blight and early blight resistance in tomato. Critical Reviews in Plant Sciences, 27, 75–107.
Fry, W. E., & Goodwin, S. B. (1997). Re-emergence of potato and tomato late blight in the United States. Plant Disease, 81, 1349–1357.
Gebhardt, C., & Valkonen, J. P. T. (2001). Organization of genes controlling disease resistance in the potato genome. Annual Review of Phytopathology, 39, 79–102.
German, M. A., Kandel-Kfir, M., Swarzberg, D., Matsevitz, T., & Granot, D. (2003). A rapid method for the analysis of zygosity in transgenic plants. Plant Science, 164, 183–187.
Hein, I., Gilroy, E. M., Armstrong, M. R., & Birch, P. R. J. (2009). The zig-zag-zig in oomycete-plant interactions. Molecular Plant Pathology, 10, 547–562.
Hoegen, E., Stromberg, A., Pihlgren, U., & Kombrink, E. (2002). Primary structure and tissue-specific expression of the pathogenesis-related protein PR-1b in potato. Molecular Plant Pathology, 3, 329–345.
Ingham, D. J., Beer, S., Money, S., & Hansen, G. (2001). Quantitative real-time PCR assay for determining transgene copy number in transformed plants. Biotechniques, 31, 132–140.
Jones, J. D., & Dangl, J. L. (2006). The plant immune system. Nature, 444, 323–329.
Moreau, P., Thoquet, P., Olivier, J., Laterrot, H., & Grimsley, N. (1998). Genetic mapping of Ph-2, a single locus controlling partial resistance to Phytophthora infestans in tomato. Molecular Plant-Microbe Interactions, 11, 259–269.
Murashige, T., & Skoog, F. (1962). A revised medium for rapid growth and bioassays with tobacco cultures. Physiology Plantarum, 15(3), 473–497.
Nicot, N., Hausman, J. F., Hoffmann, L., & Evers, D. (2005). Housekeeping gene selection for real-time RT-PCR normalization in potato during biotic and abiotic stress. Journal of Experimental Botany, 56, 2907–2914.
Park, S. H., Morris, J. L., Park, J. E., Hirschi, K. D., & Smith, R. H. (2003). Efficient and genotype-independent Agrobacterium—mediated tomato transformation. Journal of Plant Physiology, 160, 1253–1257.
Prior, F. A., Tackaberry, E. S., Aubin, R. A., & Casley, W. L. (2006). Accurate determination of zygosity in transgenic rice by real-time PCR does not require standard curves or efficiency correction. Transgenic Research, 15, 261–265.
Ronning, C. M., Stegalkina, S. S., Ascenzi, R. A., Bougr, O., Hart, A. L., Utterbach, T. R., et al. (2003). Comparative analyses of potato expressed sequence tag libraries. Plant Physiology, 131, 419–429.
Rubin, E., & Cohen, Y. (2004). Oospores associated with tomato seed may lead to seedborne transmission of Phytophthora infestans. Phytoparasitica, 32, 237–245.
Rubin, E., Baider, A., & Cohen, Y. (2001). Phytophthora infestans produces oospores in fruits and seeds of tomato. Phytopathology, 91, 1074–1080.
Schouten, H. J., Krens, F. A., & Jacobsen, E. (2006). Do cisgenic plants warrant less stringent oversight? Nature Biotechnology, 24, 753–753.
Smart, C. D., Myers, K. L., Restrepo, S., Martin, G. B., & Fry, W. E. (2003). Partial resistance of tomato to Phytophthora infestans is not dependent upon ethylene, jasmonic acid, or salicylic acid signaling pathways. Molecular Plant-Microbe Interactions, 16, 141–148.
Spassova, M. I., Prins, T. W., Folkertsma, R. T., Klein-Lankhorst, R. M., Hille, J., Goldbach, R. W., et al. (2001). The tomato gene Sw5 is a member of the coiled coil, nucleotide binding, leucine-rich repeat class of plant resistance genes and confers resistance to TSWV in tobacco. Molecular Breeding, 7, 151–161.
Tai, T. H., Dahlbeck, D., Clark, E. T., Gajiwala, P., Pasion, R., Whalen, M. C., et al. (1999). Expression of the Bs2 pepper gene confers resistance to bacterial spot disease in tomato. Proceedings of the National Academy of Science, 96, 14153–14158.
Tao, Q. Z., & Zhang, H. B. (1998). Cloning and stable maintenance of DNA fragments over 300 kb in Escherichia coli with conventional plasmid-based vectors. Nucleic Acids Research, 26, 4901–4909.
Timmerman-Vaughan, G. M., Pither-Joyce, M. D., Cooper, P. A., Russell, A. C., Goulden, D. S., Butler, R., et al. (2001). Partial resistance of transgenic peas to alfalfa mosaic virus under greenhouse and field conditions. Crop Science, 41, 846–853.
van der Vossen, E. A. G., Gros, J., Sikkema, A., Muskens, M., Wouters, D., Wolters, P., et al. (2005). The Rpi-blb2 gene from Solanum bulbocastanum is an Mi-1 gene homolog conferring broad-spectrum late blight resistance in potato. The Plant Journal, 44, 208–222.
van Loon, L. C., Rep, M., & Pieterse, C. M. J. (2006). Significance of inducible defense-related proteins in infected plants. Annual Review of Phytopathology, 44, 135–162.
Wang, X. B., ElHadrami, A., Adam, L. R., & Daayf, F. (2006). Local and distal gene expression of pr-1 and pr-5 in potato leaves inoculated with isolates from the old (US-1) and the new (US-8) genotypes of Phytophthora infestans (Mont.) de Bary. Environmental and Experimental Botany, 57, 70–79.
Whitham, S., McCormick, S., & Baker, B. (1996). The N gene of tobacco confers resistance to tobacco mosaic virus in transgenic tomato. Proceedings of the National Academy of Science, 93, 8776–8781.
Yang, L. T., Ding, J. Y., Zhang, C. M., Jia, J. W., Weng, H. B., Liu, W. X., et al. (2005). Estimating the copy number of transgenes in transformed rice by real-time quantitative PCR. Plant Cell Reports, 23, 759–763.
Acknowledgements
The authors wish to thank Michele de Martino for his technical assistance and Mark Walters for editing the manuscript.
Author information
Authors and Affiliations
Corresponding author
Rights and permissions
About this article
Cite this article
Faino, L., Carli, P., Testa, A. et al. Potato R1 resistance gene confers resistance against Phytophthora infestans in transgenic tomato plants. Eur J Plant Pathol 128, 233–241 (2010). https://doi.org/10.1007/s10658-010-9649-2
Accepted:
Published:
Issue Date:
DOI: https://doi.org/10.1007/s10658-010-9649-2