Abstract
Sodium lauryl ether sulphate (SLES) is the main chemical component in several lubricant products used for soil conditioning in the mechanized excavation industry using Earth Pressure Balance-Tunnel Boring Machines. During the tunnelling process, huge amounts of excavated soil are produced and the SLES presence can affect the subsequent re-use of this material as a by-product. Currently, there is still no regulatory indication of reliable and sensitive bioassays for monitoring soil quality during the excavation process. The main objective of this work was to verify if the Vibrio fischeri screening test was suitable as a consistent and precautionary tool for this specific purpose. Firstly, the ecotoxicity (EC20 and EC50) of the SLES standard solution and three commercial products (SLES content from 10 to 50%) were evaluated to select the most environmental friendly product. Subsequently, soil samples from about 2 years of tunnelling in a real construction site, conditioned with the selected product, were evaluated for their environmental compatibility with the prescriptions of an Italian site-specific protocol. The latter established 2 mg/L as a threshold value for SLES concentration in soil water extracts and a no toxic response (≤20%) for the Vibrio fischeri test. The comparison of the bacterium bioluminescence inhibition values (%) with analytical determinations showed an ecotoxicity when SLES was >2 mg/L. The toxicity was directly related to SLES concentration, indicating that the V. fischeri test and the SLES analyses are suitable tools for assessing excavated soil as a by-product, ensuring its safe reuse in accordance with a green production process (circular economy).
Similar content being viewed by others
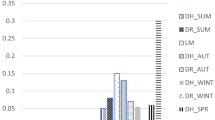
Explore related subjects
Discover the latest articles, news and stories from top researchers in related subjects.Avoid common mistakes on your manuscript.
Introduction
The widespread use of Earth Pressure Balance-Tunnel Boring Machines (EPB-TBMs) in the excavation industry (for highway, railway and metro tunnel construction) is due to the various advantages compared to the traditional drill-and-blast methods. In fact, mechanized tunnelling makes it possible to reduce the damage at surface level, lower the environmental impact on groundwater circulation, increase tunnelling speed and have safer working conditions, while lowering project costs (EFNARC 2005; Huang et al. 2018). EPB-TBM performance involves the use of commercial lubricant products (foaming agents) to condition the soil, reducing hard rock abrasiveness and facilitating excavated material transportation (Vinai et al. 2008; Peila et al. 2016). The type and amount of products depend mainly on both the TBM and excavated material characteristics (Peila et al. 2007; Peila 2014; Talebi et al. 2015). The EPB-TBM mechanized process produces huge amounts of spoil material (soil debris) which can contain commercial soil-conditioning products. Several of these consist of water solutions of the anionic surfactant sodium lauryl ether sulphate (SLES) in percentages varying from 5 to 50%; in some cases, other minor components are also present (Barra Caracciolo et al. 2017). SLES is an anionic surfactant (ANS) that can be composed by several homologues, with the hydrocarbon and the ethoxy tails that can differ in composition and length. ANS are considered by the European Chemical Agency as Unknown or Variable composition, Complex reaction products. SLES can have several synonyms (such as Alcohols, C10-16, ethoxylated, sulfates, sodium salts; Alcohols, C12-14, ethoxylated, sulfates, sodium salts; 2-dodecoxyethyl hydrogen sulfate), and can have different CAS (e.g. CAS#: 68585-34-2; 68891-38-3; 9004-82-4) and EC numbers (e.g. EC#: 500-223-8; 500-234-8; 618-398-5), (Barra Caracciolo et al. 2017; Finizio et al. 2020). High concentrations of SLES in soil debris (ranging from 26 to 350 mg/kg soil; Finizio et al. 2020) may pose a risk for the environment, including the aquatic one if there is a direct contact with water bodies (Mininni et al. 2018; Grenni et al. 2019; Finizio et al. 2020).
In the framework of EU policies on environmental protection such as the 7th Environment Action Programme to 2020 (European Commission 2019) one of the main objectives is a “circular economy where nothing is wasted and where natural resources are managed sustainably”. Consequently, to avoid production of millions of tonnes of waste as spoil material, the reuse of excavated soil as a by-product is desirable and implemented in most EU Countries, in line with the circular economy model (D’Aloia Schwartzentruber et al. 2019; Murr et al. 2019; Padulosi et al. 2019). Currently, at EU level there are no specific regulations for soil (European Commission 2012) or even for this specific matrix, although the use of excavated soil as a by-product is potentially regulated by the EU Directive 98/2008 relating to waste (D’Aloia Schwartzentruber et al. 2019; ITA-AITES 2019). In Italy, spoil material from excavation processes can currently be re-used as a by-product if it meets environmental legislative criteria. In particular, the chemical thresholds for several organic and inorganic contaminants should not be exceeded (Italian Decrees 161/2012, 120/2017 and 152/2006). The chemicals to be investigated are some inorganic elements (As, Cd, Cb, Ni, Pb, Cu, Zn, Hg, total Cr and Cr VI), Hydrocarbons (C > 12), asbestos, BTEX (Benzene, Toluene, Ethylbenzene and Xylene) and PAHs (Polycyclic Aromatic Hydrocarbons). However, there are currently no threshold limits for SLES concentrations in either European or Italian legislation, although SLES residues in re-used excavated materials could pose an environmental risk (Finizio et al. 2020). In the case of lubricant products containing chemicals not listed in the above-mentioned Decrees, a technical document showing that the excavated soils do not represent a risk for the environmental and human health has to be approved by the Italian authorities (Padulosi et al. 2019).
Indications on the assessment of the environmental compatibility of soil debris, applying an ecological approach as regards both the foaming agent degradation and ecotoxicological effects, are lacking in the regulatory framework. An ecotoxicological approach could be very useful for evaluating the overall effects of chemical mixtures occurring in soil treated with foaming agents (Meistro et al. 2019; Padulosi et al. 2019; Grenni et al. 2019). In fact, ecotoxicological tests overcome the limitations of chemical analysis by detecting only what is searched for, making it possible to identify both the additive and synergic effects of all environmental contaminants, including unknown ones, in an environmental matrix (Bispo et al. 1999; Ma et al. 2014). In this context, site-specific degradation studies were recently performed in microcosms, using different soils conditioned with two different commercial foaming agents, and demonstrated that SLES is a biodegradable compound (Grenni et al. 2018; Barra Caracciolo et al. 2019; Finizio et al. 2020). In fact, this anionic surfactant halved from initial concentrations of 70–100 mg/kg in 6–9 days, depending on the soil considered (Barra Caracciolo et al. 2019). Moreover, in another study, ecotoxicological tests on the terrestrial plant Lepidium sativum (OECD 2006), using the same spoil material conditioned with the same foaming agents, but with higher (191–350 mg/kg) concentrations, showed that SLES was non-toxic for the target species tested (Grenni et al. 2018). Furthermore, a battery test (using the Vibrio fischeri, Pseudokirchneriella subcapitata, Daphnia magna and Danio rerio embryo) was also performed on the same conditioned soils to estimate if the overall toxicity of soil water extracts decreased over time. These aquatic species were selected in order to hypothesize a worst-case scenario at the final destination site, with SLES from the soil debris being transported via runoff or leaching to a water body. The ecotoxicological tests were performed at different times from the soil conditioning in order to reproduce the temporary storage of the spoil material in the construction area, where SLES degradation can occur naturally, making the spoil material non-toxic. The results showed that the initial SLES ecotoxicity for the aquatic species decreased over time, in line with the reduction in SLES concentration in soil water extracts (Grenni et al. 2018). Finally, another recent experiment (Galli et al. 2019) performed with both L. sativum and Eisenia foetida (OECD 2006; 1984) confirmed that SLES concentrations in spoil materials are generally not toxic for terrestrial organisms, suggesting that aquatic species are more sensitive to SLES residues, as reported for surfactants in other studies (Barra Caracciolo et al. 2019). Among all the organisms tested, the bacterium V. fischeri (UNI EN ISO 2019) was one of the most sensitive to residual concentrations of the anionic surfactant SLES (Grenni et al. 2018). This species was also found to be highly sensitive to other surfactants (Farré et al. 2001b), including the anionic Sodium Dodecyl Sulphate (SDS), (Mariani et al. 2006), also commonly used as a reference toxicant.
The bioluminescent bacteria Vibrio fischeri is currently considered a useful biosensor in environmental risk assessments for chemicals (Parvez et al. 2006; Lopez-Roldan et al. 2012; Abbas et al. 2018). This test has also been successfully used with various environmental matrices containing chemicals, such as metals (Guéguen et al. 2004; Hsieh et al. 2004; Ishaque et al. 2006; Rosen et al. 2008; Tsybulskii and Sazykina 2010; Yang et al. 2016), PCBs and PAHs (Salizzato et al. 1998; Bispo et al. 1999; Serafim et al. 2013). The test made it possible to evaluate the harmful impacts of various complex matrices such as effluents (Reemtsma et al. 1999; Wang et al. 2002; Farré et al. 2008), sewage sludge (Farré et al. 2001a; Farré and Barceló 2003), landfill leachate (Thomas et al. 2009) and even soil, sediments and air (Girotti et al. 2008; Chen et al. 2017; Abbas et al. 2018). The test can be performed on soil water extracts or by directly exposing bacteria to a contaminated matrix and provides an accurate toxicity assessment (Bláha et al. 2010; Jarque et al. 2016).
This paper reports a data set of V. fischeri tests and SLES chemical determinations in soil water extracts from a real case study of a tunnel excavation in Central Italy. The ecotoxicological and chemical analyses were performed in accordance with a site-specific protocol (ISPRA 2016), agreed with the Italian Ministry of the Environment, which defined the criteria for classifying the spoil material as a by-product. For this purpose, the acute toxicity screening test with Vibrio fischeri and the analytical determinations of residual SLES concentrations, using the MBAS method on elutriates from each sample, were selected as the suitable parameters for evaluating the environmental compatibility of the spoil material from a tunnelling construction site. The overall results of more than 2 years of data collection are reported and discussed here.
Materials and methods
Study area and selection of the commercial lubricant product (foaming agent)
The geographical location of the EBP-TBM tunnel excavation was in Central Italy. Three main soil lithologies were found: gravel in a sandy-silty-clay soil (SS), gravel in a sandy-silty soil (MM) and a mixture of both (MIX). For these lithologies, three commercial lubricant products (foaming agents, FA) were identified as suitable for the tunnelling. The amount of each product (Treatment Ratio, TR, L/m3 soil) for conditioning the excavated material was selected with geotechnical tests (slump cone tests) based on each soil type (EFNARC 2005). The slump tests were performed at Polytechnic University of Turin (Italy) where a laboratory foam generation system, reproducing the conditioning activity carried out by an EPB-TBM, was available (Borio and Peila 2011; Peila 2014; Peila et al. 2016).
The three foaming agents, termed FA1, FA2 and FA3, contained a water solution where SLES was present from 10 to 30% (Table 1). In order to select the most environmental friendly FA for use at the excavation site, ecotoxicological evaluations, using the Vibrio fischeri test, were performed. For this purpose, the effective concentrations (EC20 and EC50 values) of a SLES standard solution and of the three foaming agents were determined.
The SLES concentrations expected in the conditioned soil were also calculated for each foaming agent (FA1, FA2 and FA3) by converting the TR values (L/m3) of each FA into mg SLES/kg soil, considering a soil density of 1.54 t/m3 and each specific product density (Table 1). Each product had a range of SLES concentration (%) indicated in the chemical safety data sheet. The same soil samples used for the slump cone tests were also analysed for determining the actual SLES concentrations.
Site-specific protocol for monitoring the environmental compatibility of excavated soil
In compliance with the Italian legislation, if the spoil material produced from each tunnelling construction work is used as a by-product, it has to be demonstrated that it is not waste. Owing to the lack of legal threshold limits for surfactant anions, the Italian Ministry of the Environment required specific ecotoxicological studies for the selection of the most environmental friendly foaming agent. For this purpose, a “site-specific protocol” was designed on the basis of the ecotoxicological evaluations of the foaming agents FA1, FA2 and FA3, the maximum concentrations of SLES expected in the soils conditioned (Table 1) and other site-specific environmental considerations mentioned in the “Report on the final destination and use of the excavated soil”. The Engineering contractor is required to produce this Report by the national legislation (Italian Decree 120/2017). The site-specific protocol was drawn up by the Italian Institute for Environmental Protection and Research together with the Water Research Institute of the National Research Council, the Italian National Institute of Health and the Tuscany Regional Environmental Agency (ISPRA 2016). In the study case reported here, the final destination of the excavated soil was a landfill in an area to be used for an industrial building and unrelated to any water bodies. The protocol also established in detail the operating procedures for sampling the spoil material and performing both the chemical analysis of the anionic surfactant SLES and the V. fischeri test on the soil elutriates. In particular, it established 2 mg/L as a threshold value for SLES concentration in soil water extracts and a no toxic response (≤20%) for the ecotoxicological test with the bacterium Vibrio fischeri. Both parameters needed to be satisfied to classify the spoil material as a by-product.
Sampling and preparation of excavated soil for analysis
During the tunnelling, spoil material was directly transported by a conveyor belt to the temporary deposit areas inside the construction site where it was stocked for some days before its final use. The provisional storage of excavated soil was generally 7 days and in some cases more, depending on the results of the environmental compatibility analyses foreseen in the site-specific protocol mentioned above.
After the storage period, a composite sample was collected from the deposit area, in accordance with the operating procedures set out by specific guidelines from the Tuscany Regional Environment Agency. The sample was dried at the environmental temperature, sieved (2 mm) and then used for both the chemical analyses (foreseen in Italian Decree 152/2006, Annex 1, Section V) and for evaluating the environmental compatibility, as specified in the “Site-specific protocol” (ISPRA 2016). In this regard, the analyses were performed on the soil water extracts (elutriates), which were prepared in a solid/liquid ratio of 1:10 with distilled water (taking the moisture already in the sample into account). The elutriates were obtained following standardized procedures for waste characterization (UNI EN 2004) and in accordance with other authors (Hubálek et al. 2007; Marguí et al. 2016). In brief, each soil sample (3 replicates, 100 g each) was put into a bottle (1 L) with distilled water. The bottles were shaken to simulate the leaching process (in the dark for 24 h, 20 °C). After the solid particles fell to the bottom (15 min), the supernatant phase was than centrifuged (15 min at 9000 rpm). The elutriates were subsequently processed for SLES determination or filtered (0.45 µm, cellulose acetate Whatman) for ecotoxicological analysis in accordance with UNI EN 2005. The elutriates obtained were stored in polyethylene vials at 4 °C until ecotoxicological and chemical analysis. The environmental compatibility analyses were performed on soil water extracts of about 150 soil samples collected during more than 2 years of tunnel excavation.
Chemicals and SLES determination
Chemical Chloroform and methanol of HPLC grade, sulphuric acid (98%) and methylene blue were obtained from VWR (Radnor, PA, USA). Sodium hydrogen carbonate and anhydrous sodium carbonate were purchased from Carlo Erba Reagents (Milano, Italy). The water was purified (18 MΩ/cm quality) using a Milli-Q system (Millipore, Bedford, MA, USA). The SLES standard (CAS#: 68585-34-2, technical grade purity of 71.92%) was purchased from BOC Sciences (US, Canada) and used as the MBAS (Methylene Blue Active Substances) reference compound. The stock solution of SLES was prepared at 1000 mg/L in methanol and stored at −20 °C. Two calibration curves for SLES were obtained by analysing working standard solutions in lower (0.05–0.5 mg/L) and higher (0.5–4.0 mg/L) concentration ranges.
The SLES extraction from conditioned soil was performed by Pressurized Liquid Extraction (PLE), as described in detail in Barra Caracciolo et al. (2019). The PLE extracts and the soil water extracts (elutriates) were analysed for SLES content with a quick and easy method that could be used by the national and regional agencies in charge of environmental monitoring. In particular, the optimized MBAS spectrophotometric method, reported in detail elsewhere (Grenni et al. 2018), was used. SLES was extracted three times with solvent chloroform to obtain a blue salt. The intensity of the blue colour of the SLES-MBAS complex was then measured using a UV/Vis spectrophotometer (Perkin Elmer 25) at a wavelength of 650 nm. The SLES concentration was finally calculated using the previously determined calibration curve. The limit of detection (LOD) (Thompson et al. 2002) was 0.013 mg/L. SLES was determined in both the FA solutions prepared for evaluating EC20 and EC50 values and in soil water extracts produced from the spoil material over 2 years of the tunnelling.
Ecotoxicological tests
The Vibrio fischeri acute toxicity test was performed using a Microtox® analyser (Model 500, Modern Water, UK) in accordance with both the manufacturer’s instructions and the standard method (UNI EN ISO 2019). This test is based on the inhibition of the luminescence naturally emitted by the bacterium Vibrio fischeri after its exposure to a sample. The difference in light output between the sample and the blank (saline solution, 20 g/L NaCl) is due to the sample effect on the organisms, measured at 30 min of exposure. The endpoint (luminescence inhibition %) is calculated using the Microtox calculation software (Microtox Omni® software V 4.2).
The lyophilized, freeze-dried Vibrio fischeri (strain NRRL B-11177) and the reconstitution solution (RS) for the bacteria were purchased from Ecotox LDS s.r.l. (Milan, Italy). To achieve a ready-to-use bacterial suspension, the lyophilized bacteria (batch number 17H4227) were rehydrated with the RS. Before starting the test, an osmotic adjustment was performed, using a saline solution (22% w/v NaCl in Milli-Q water) to make the sample salinity optimal (2%) for V. fischeri.
The toxicity of the SLES standard solution and foaming agents (FA1, FA2 and FA3) was expressed as the effective concentration (EC) causing 20% (EC20) and 50% (EC50) of luminescence inhibition. The EC20 and EC50 values were determined with various dilutions (using distilled water) of a stock solution (50 mg SLES standard/L water) of SLES; 7 solutions (0.6, 1.3, 2.6, 5.1, 10.2, 20.5, 40.9 mg/L) were obtained and tested. In a similar way, a stock solution (127.6, 130.5, 127.6 mg FA/L water for FA1, FA2 and FA3, respectively) for each foaming agent was diluted to obtain 7 solutions (1.6, 3.3, 6.5, 13.1, 26.1, 52.3, 104.5 mg/L) to be tested. The concentrations of SLES in the solutions were analysed with the MBAS method above described. The test was repeated three times; the EC20 and EC50 (average values) were calculated using the Microtox software.
The screening tests on spoil material were performed on 6 replicates of soil water extract (elutriates) using a 1:10 ratio soil/water following the procedure described in UNI EN 2005. At the same time, the saline solution (blank, 20 g/L NaCl) and the reference toxicant (3,5-Dichlorophenol aqueous solution, 3.5 mg/L) were used in all tests as negative and positive controls, respectively. The pH value was recorded and eventually neutralized, as required by the standard procedures for the V. fischeri test. The coefficient of variation ratio (CVR% = standard deviation/mean × 100) for test reproducibility was also calculated. If necessary, a correction of the colour or turbidity of the elutriates was applied in accordance with the standardized method and with procedural adaptations by other authors (Lappalainen et al. 2001; Volpi Ghirardini et al. 2009; Jarque et al. 2016). The colour correction was useful for avoiding interference factors that could cause false toxic responses.
Results
Toxicity of SLES standard solution and foaming agents
Figure 1 shows the dose-response relationship for SLES standard solution. The bioluminescence inhibition of the bacterium V. fischeri expressed as EC20 and EC50 corresponded to average SLES concentrations of 1.3 ± 0.1 mg/L and 4.00 ± 0.1 mg/L, respectively (Table 2). The coefficient of variation ratio was always ≤20%, see Table 2 (Environment Canada 2007).
The dose-response relationships for the three commercial lubricant products (foaming agents, FA) considered in this study are reported in Fig. 2a–c for FA1, FA2 and FA3, respectively.
The EC20 and EC50 average values estimated for the foaming agent FA1 (5.8 and 17.3 mg/L, respectively) were almost two times higher than those of FA2 and FA3 (2.2 and 6.9 mg/L, respectively), demonstrating that FA1 was less toxic than the other two FAs (Table 2). Moreover, the lowest SLES concentrations expected in soils were also those of FA1 (Table 2). The latter result was confirmed by the actual SLES concentrations; in fact, the average values measured for the three lithologies (SS, MM and MIX) in conditioned soil samples were: FA1: 177.5 mg/kg < FA2: 232.3 mg/kg < FA3: 250.5 mg/kg.
For these reasons, FA1 was selected as the commercial product to be used for the tunnelling and included in the site-specific Protocol for conditioning the soil at the actual excavation site.
Environmental compatibility of excavated soil in accordance with the site-specific protocol
As mentioned above, the tunnel excavation was performed using the FA1 commercial product as the conditioning agent at the treatment ratios indicated in Table 1. The site-specific protocol established that the spoil material produced weekly during the tunnelling should be put in temporary deposit containers constructed at the excavation site. Before its final destination, the environmental compatibility analyses foreseen in the site-specific protocol (ecotoxicological test and SLES concentration in elutriates) were performed 7 days after the temporary storage. The V. fischeri toxic responses (% bioluminescence inhibition at 30 min) and the SLES residual concentrations (mg/L) measured in soil elutriate samples over time, are reported as monthly averages in Fig. 3. The CVR values for V. fischeri tests were always ≤20% (Environment Canada 2007). SLES concentration in the spoil material and its effect percentage did not exceed the threshold limit of 2 mg/L and bioluminescence inhibition of 20% in most cases. In a few cases the samples required more time (ranging from 15 to 18 days) to be in line with the Protocol thresholds. Interestingly, when plotting the chemical (SLES residual concentrations) versus the ecotoxicological data, a significant (r = 0.8; p < 0.01) correlation was found. This means that the bacterium bioluminescence inhibition was directly correlated to SLES residues detected in the elutriate, as shown in Fig. 4. However, there were a few samples where, although the concentration of SLES was less than 2 mg/L, a bioluminescence inhibition higher than 20% was found.
Discussion
In the coming years, due to the numerous tunnelling projects planned in Europe and worldwide, several hundreds of millions of tons of spoil material containing SLES will be produced. A recent paper (Finizio et al. 2020) reports the environmental risk assessment of SLES in 12 foaming agents used for tunneling in site-specific conditions. Owing to the presence of residual SLES concentrations in the material excavated and to the fact that it can be in contact with aquatic bodies at some final destination sites, potential threats to the natural environment have to be taken into account. This work reports the application of the Vibrio fischeri test to the SLES compound on its own, to three commercial products containing SLES as the main component in a variable percentage and finally to soil treated with a foaming agent (FA1) from a real tunnelling project. V. fischeri is a gram-negative luminescent bacterium and its exposure to toxic substances causes the inhibition of enzymatic activity, corresponding to a decrease in bioluminescence. The light intensity reflects the bacterium’s metabolic status and is related to the toxicity of a xenobiotic (Meighen 1991, 1993; Ma et al. 2014). The theoretical effective concentrations evaluated using SLES on its own showed V. fischeri to be very sensitive to the anionic surfactant and confirm that it is potentially harmful for aquatic organisms (Ying 2006; Mariani et al. 2006; Karray et al. 2016). Other comparisons performed with pharmaceuticals and personal care products containing surfactants reported that V. fischeri exhibited greater sensitivity than assays involving other aquatic test organisms (Ortiz de García et al. 2014). A review reported the effective concentrations of SLES for various aquatic organisms and found EC50 values higher (less toxicity) for crustaceans, fish and other invertebrates and a comparable value for a microalga (Barra Caracciolo et al. 2017). The ecotoxicological analysis of the foaming agents showed how their intrinsic toxicity (EC20 and EC50) is ascribable to their percentage content of SLES (Table 1). The SLES percentage in FA1 (10–20%) was lower than in the other FAs (FA2 < 30% and FA3 10–30%). In accordance with these data, the effective concentrations (EC20: 5.8 mg/L and EC50: 17 mg/L) for V. fischeri in the case of FA1 were higher than the other two commercial products, owing to a lower SLES concentration (Table 2). The SLES expected in the spoil material depended on the TR used for the soil conditioning and the percentage of the anionic surfactant in each foaming agent (see TR values and SLES % for each FA in Table 1). Consequently, in the FA1 conditioned soil lower SLES concentrations would be expected, as detected in this study.
Surfactants are known to be toxic for microorganisms at a critical micelle concentration (CMC) and SLES is reported to form micelles at a concentration higher than 300 mg/L (Aoudia et al. 2009). Surfactant micelles can solubilize the membrane lipid of bacterial cells and lead to cell lysis (Glover et al. 1999; Li and Chen 2009). On the other hand, the bacterium Vibrio fischeri was particularly sensitive to SLES, showing a toxic response at concentrations that were very low and much below the CMC.
The overall data sets (150 samples over 2 year of analyses) of the FA1-conditioned soil samples from the tunnelling construction site definitely confirmed the relationship between SLES residues and its toxicity (Fig. 4). The amount and degradation of the anionic surfactant in the spoil material during its storage in temporary deposit areas is a key factor for its potential toxicity in the corresponding soil water extract, as found in a previous work (Grenni et al. 2018). In all cases where the bioluminescence inhibition was less than 20%, SLES was present at residual concentrations ≤2 mg/L in the elutriates. The fact that some samples were not in compliance with the threshold of 2 mg/L after 7 days from the conditioning depended on a delay in the SLES degradation, presumably due to abiotic factors (e.g. temperature, water soil content). Moreover, in another few cases, although SLES concentrations were very low (much less than 2 mg/L), a significant effect (>20%) on the V. fischeri was found. This could be ascribable by other chemicals in the soil (not included in the Italian decrees on spoil materials from tunnelling) which can negatively affect the bacterium response, showing how this test is more reliable than the chemical analysis alone of a single component such as SLES. In fact, the ecotoxicological response refers to effects of overall chemicals of a sample. Other studies used V. fischeri as an effective screening test for soil samples (Parvez et al. 2006; Abbas et al. 2018). However, this work is the first one with a huge number of soil debris samples, produced from a long time real excavation work, which has demonstrated a significant correlation between SLES content and V. fischeri response.
The overall results showed that V. fischeri, beyond its sensitivity to low SLES concentrations, was a useful, reliable and reproducible tool for meeting tunnelling operational needs (e.g. short time for obtaining the results) and making it possible to recycle spoil material as a safe by-product in line with a circular economy strategy.
The test organism has a high sensitivity to various contaminants (Parvez et al. 2006; Yang et al. 2016; Wieczerzak et al. 2016; Abbas et al. 2018) and the test procedure makes it possible to obtain reliable and reproducible data thanks to its standardized method, involving an automatic equipment. The test is cost-effective and can be performed quickly, since bacterial metabolism responds promptly to the presence of a toxic compound (Coz et al. 2007). All these characteristics make the test a suitable tool for evaluating the environmental compatibility of foaming agent conditioned-soil. For this reason, we suggest the bioluminescence inhibition test be included as a precautionary tool in a regulatory framework for evaluating the environmental compatibility of spoil material.
Conclusions
The successful use, in support of analytical determinations, of V. fischeri as an ecotoxicological tool for assessing the environmental compatibility of spoil materials containing FAs was demonstrated thanks to the significant correlation between the anionic surfactant SLES and ecotoxicity. Moreover, the effectiveness of the site-specific protocol used for assessing the environmental compatibility of a huge amount of soil debris from the real excavated site was validated.
The bioluminescence inhibition test made it possible to obtain information in a real case study about the different interactions among the mixture (soil debris and foaming agent) and the specific matrix (different soil lithologies conditioned with different FA amounts), and the possible ecotoxicological effects. If in the soil excavated there was an unknown element, the test showed a higher toxicity than expected, as occurred in some samples. The test characteristics in terms of SLES sensitivity, reliable response and fast and easy application made it possible to quickly assess if the spoil material could be used as a by-product.
References
Abbas M, Adil M, Ehtisham-Ul-Haque S, Munir B, Yameen M, Ghaffar A, Shar GA, Asif Tahir M, Iqbal M (2018) Vibrio fischeri bioluminescence inhibition assay for ecotoxicity assessment: a review. Sci Total Environ 626:1295–1309. https://doi.org/10.1016/j.scitotenv.2018.01.066
Aoudia M, Al-Maamari T, Al-Salmi F (2009) Intramolecular and intermolecular ion-dipole interactions in sodium lauryl ether sulfates (SLES) self-aggregation and mixed micellization with Triton X-100. Colloids Surf A Physicochem Eng Asp 335:55–61. https://doi.org/10.1016/j.colsurfa.2008.10.026
Barra Caracciolo A, Ademollo N, Cardoni M, Di Giulio A, Grenni P, Pescatore T, Rauseo J, Patrolecco L (2019) Assessment of biodegradation of the anionic surfactant sodium lauryl ether sulphate used in two foaming agents for mechanized tunnelling excavation. J Hazard Mater 365:538–545. https://doi.org/10.1016/j.jhazmat.2018.11.002
Barra Caracciolo A, Cardoni M, Pescatore T, Patrolecco L (2017) Characteristics and environmental fate of the anionic surfactant sodium lauryl ether sulphate (SLES) used as the main component in foaming agents for mechanized tunnelling. Environ Pollut 226:94–103. https://doi.org/10.1016/j.envpol.2017.04.008
Bispo A, Jourdain M, Jauzein M (1999) Toxicity and genotoxicity of industrial soils polluted by polycyclic aromatic hydrocarbons (PAHs). Org Geochem 30:947–952. https://doi.org/10.1016/S0146-6380(99)00078-9
Bláha L, Hilscherová K, Čáp T, Klánová J, Machát J, Zeman J, Holoubek I (2010) Kinetic bacterial bioluminescence assay for contact sediment toxicity testing: Relationships with the matrix composition and contamination. Environ Toxicol Chem 29:507–514. https://doi.org/10.1002/etc.81
Borio L, Peila D (2011) Laboratory test for EPB tunnelling assessment: results of test campaign on two different granular soils. Gospod Surowcami Miner 27:85–100. https://min-pan.krakow.pl/wp-content/uploads/sites/4/2017/12/borio-peila.pdf. Accessed 10 Mar 2019
Chen SS, Sun Y, Tsang DCW, Graham NJD, Ok YS, Feng Y, Li XD (2017) Potential impact of flowback water from hydraulic fracturing on agricultural soil quality: Metal/metalloid bioaccessibility, Microtox bioassay, and enzyme activities. Sci Total Environ 579:1419–1426. https://doi.org/10.1016/j.scitotenv.2016.11.141
Coz A, González-Piñuela C, Andrés A, Viguri JR (2007) Physico-chemical and environmental characterisation of sediments from Cantabrian estuaries (Northern Spain). Aquat Ecosyst Health Manag 10(1):41–46. https://doi.org/10.1080/14634980701212118
D’Aloia Schwartzentruber L, Robert F (2019) Management and use of materials excavated during underground works. In: Peila D, Viggiani G, Celestino T (eds) Tunnels and underground cities. Engineering and innovation meet archaeology, architecture and art. Proceedings of the WTC 2019 ITA-AITES World Tunnel Congress (WTC 2019). CRC Press, Taylor and Francis group, London, UK, pp 284–293
EFNARC (2005) Specification and guidelines for the use of specialist products for mechanised tunnelling (TBM) in soft ground and hard rock. EFNARC, The European Federation of Specialist Construction Chemicals and Concrete Systems. http://www.efnarc.org/pdf/TBMGuidelinesApril05.pdf. Accessed 10 Mar 2019
Environment Canada (2007) Guidance Document on Statistical methods for environmental toxicity tests. Method Development and Applications Section, Environmental Technology Centre, Environment Canada. Environmental Protection series. EPS 1/RM/46. 283 pp. http://publications.gc.ca/site/eng/278313/publication.html. Accessed 10 Jan 2020
European Commission (2019) Environment Action Programme to 2020. European Commission. http://ec.europa.eu/environment/action-programme. Accessed 18 June 2019
European Commission (2012) COM(2012) 46 final, Report From the Commission to the European Parliament, the Council, The European Economic and Social Committee and the Committee of the Regions the implementation of the Soil Thematic Strategy and ongoing activities. European Commission. https://eur-lex.europa.eu/legal-content/EN/TXT/?uri=CELEX%3A52012DC0046. Accessed 1 Mar 2020
Finizio A, Patrolecco L, Grenni P, Galli E, Muzzini VG, Rauseo J, Rizzi C, Barra Caracciolo A (2020) Environmental risk assessment of the anionic surfactant sodium lauryl ether sulphate in site-specific conditions arising from mechanized tunnelling. J Hazard Mater 383:121116. https://doi.org/10.1016/j.jhazmat.2019.121116
Galli E, Muzzini VG, Finizio A, Fumagalli P, Grenni P, Barra Caracciolo A, Rauseo J, Patrolecco L (2019) Ecotoxicity of foaming agent conditioned soils tested on two terrestrial organisms. Environ Eng Manag J 18:1703–1710
Girotti S, Ferri EN, Fumo MG, Maiolini E (2008) Monitoring of environmental pollutants by bioluminescent bacteria. Anal Chim Acta 608:2–29. https://doi.org/10.1016/j.aca.2007.12.008
Glover RE, Smith RR, Jones MV, Jackson SK, Rowlands CC (1999) An EPR investigation of surfactant action on bacterial membranes. FEMS Microbiol. Lett. 177(1):57–62. https://doi.org/10.1016/S0378-1097(99)00289-X
Grenni P, Barra Caracciolo A, Patrolecco L, Ademollo N, Rauseo J, Saccà ML, Mingazzini M, Palumbo MT, Galli E, Muzzini V, Polcaro CM, Donati E, Lacchetti I, Di Giulio A, Gucci P, Beccaloni E, Mininni G (2018) A bioassay battery for the ecotoxicity assessment of soils conditioned with two different commercial foaming products. Ecotoxicol Environ Saf 148:1067–1077. https://doi.org/10.1016/j.ecoenv.2017.11.071
Grenni P, Barra Caracciolo A, Patrolecco L (2019) Site-specific protocols for evaluating environmental compatibility of spoil materials produced by EPB-TBMs. In: Peila D, Viggiani G, Celestino T (eds) Tunnels and underground cities. Engineering and innovation meet archaeology, architecture and art. CRC Press, Taylor and Francis group, London, UK, pp 360–366
Guéguen C, Gilbin R, Pardos M, Dominik J (2004) Water toxicity and metal contamination assessment of a polluted river: the Upper Vistula River (Poland). Appl Geochemistry 19:153–162. https://doi.org/10.1016/S0883-2927(03)00110-0
Hsieh C-Y, Tsai M-H, Ryan DK, Pancorbo OC (2004) Toxicity of the 13 priority pollutant metals to Vibrio fisheri in the Microtox® chronic toxicity test. Sci Total Environ 320:37–50. https://doi.org/10.1016/S0048-9697(03)00451-0
Huang X, Liu Q, Shi K, Pan Y, Liu J (2018) Application and prospect of hard rock TBM for deep roadway construction in coal mines. Tunn Undergr Sp Technol 73:105–126. https://doi.org/10.1016/j.tust.2017.12.010
Hubálek T, Vosáhlová S, Matějů V, Kovácová N, Novotný C (2007) Ecotoxicity monitoring of hydrocarbon-contaminated soil during bioremediation: a case study. Arch Environ Contam Toxicol 52:1–7. https://doi.org/10.1007/s00244-006-0030-6
Ishaque A, Johnson L, Gerald T, Boucaud D, Okoh J, Tchounwou PB (2006) Assessment of individual and combined toxicities of four non-essential metals (As, Cd, Hg and Pb) in the microtox assay. Int J Environ Res Public Health 3:118–120. https://doi.org/10.3390/ijerph2006030014
ISPRA (2016) Protocol for the assessment of the environmental compatibility of excavated earth and rocks to be protected during construction for the construction of the S. Lucia Tunnel of the A1 Barberino del Mugello - Calenzano motorway. Rome Italian Institute for Environmental Protection and Research (Protocollo per la valutazione della compatibilità ambientale delle terre e rocce da scavo da adottare in corso d’opera per la realizzazione della Galleria S. Lucia dell’Autostrada A1 Barberino del Mugello–Calenzano. Rome. Istituto superiore per la protezione e la ricerca ambientale. In Italian)
ITA-AITES (2019) WG14+WG15—Mechanized tunnelling+underground and environment—handling treatment and disposal of tunnel spoil materials. Società Italiana Gallerie. https://about.ita-aites.org/files/WG14-15-ITA-REPORT-MudHandling.pdf. Accessed 10 Mar 2020
Jarque S, Masner P, Klánová J, Prokeš R1, Bláha L (2016) Bioluminescent Vibrio fischeri assays in the assessment of seasonal and spatial patterns in toxicity of contaminated river sediments. Front Microbiol 7:1738. https://doi.org/10.3389/fmicb.2016.01738
Karray F, Mezghani M, Mhiri N, Djelassi B, Sayadi S (2016) Scale-down studies of membrane bioreactor degrading anionic surfactants wastewater: isolation of new anionic-surfactant degrading bacteria. Int Biodeterior Biodegradation 114:14–23. https://doi.org/10.1016/j.ibiod.2016.05.020
la Farré M, Pérez S, Kantiani L, Barceló D (2008) Fate and toxicity of emerging pollutants, their metabolites and transformation products in the aquatic environment. TrAC Trends Anal Chem 27:991–1007. https://doi.org/10.1016/j.trac.2008.09.010
la Farré M, Barceló D (2003) Toxicity testing of wastewater and sewage sludge by biosensors, bioassays and chemical analysis. TrAC Trends Anal Chem 22:299–310. https://doi.org/10.1016/S0165-9936(03)00504-1
la Farré M, Ferrer I, Ginebreda A, Figueras M, Olivella L, Tirapu L, Vilanova M, Barceló D (2001a) Determination of drugs in surface water and wastewater samples by liquid chromatography–mass spectrometry: methods and preliminary results including toxicity studies with Vibrio fischeri. J Chromatogr A 938:187–197. https://doi.org/10.1016/S0021-9673(01)01154-2
la Farré M, Garcı́a M-J, Tirapu L, Ginebreda A, Barceló D (2001b) Wastewater toxicity screening of non-ionic surfactants by Toxalert® and Microtox® bioluminescence inhibition assays. Anal Chim Acta 427:181–189. https://doi.org/10.1016/S0003-2670(00)01022-9
Lappalainen J, Juvonen R, Nurmi J, Karp M (2001) Automated color correction method for Vibrio fischeri toxicity test. Comparison of standard and kinetic assays. Chemosphere 45:635–641. https://doi.org/10.1016/S0045-6535(00)00579-8
Li JL, Chen BH (2009) Surfactant-mediated biodegradation of polycyclic aromatic hydrocarbons Materials 2(1):76–94. https://doi.org/10.3390/ma2010076
Lopez-Roldan R, Kazlauskaite L, Ribo J, Riva MC, González S, Cortina JL (2012) Evaluation of an automated luminescent bacteria assay for in situ aquatic toxicity determination. Sci Total Environ 440:307–313. https://doi.org/10.1016/j.scitotenv.2012.05.043
Ma XY, Wang XC, Ngo HH, Guo W, Wu MN, Wang N (2014) Bioassay based luminescent bacteria: interferences, improvements, and applications. Sci Total Environ 468–469:1–11. https://doi.org/10.1016/j.scitotenv.2013.08.028
Marguí E, Iglesias M, Camps F, Sala L, Hidalgo M (2016) Long-term use of biosolids as organic fertilizers in agricultural soils: potentially toxic elements occurrence and mobility. Environ Sci Pollut Res 23:4454–4464. https://doi.org/10.1007/s11356-015-5618-9
Mariani L, De Pascale D, Faraponova O, Tornambè A, Sarni A, Giuliani S, Ruggiero G, Onorati F, Magaletti E (2006) The use of a test battery in marine ecotoxicology: the acute toxicity of sodium dodecyl sulfate. Environ Toxicol 21:373–379. https://doi.org/10.1002/tox.20204
Meighen EA (1991) Molecular biology of bacterial bioluminescence. Microbiol Rev 5:123–142
Meighen EA (1993) Bacterial bioluminescence: organization, regulation, and application of the lux genes. FASEB J 7:1016–1022. https://doi.org/10.1096/fasebj.7.11.8370470
Meistro N, Parisi G, Zippo C, Captini L (2019) Management of tunneling machines excavation material. In: Peila D, Viggiani G, Celestino T (eds) Tunnels and underground cities. Engineering and innovation meet archaeology, architecture and art. Proceedings of the WTC 2019 ITA-AITES World Tunnel Congress (WTC 2019). CRC Press, Taylor and Francis group, London, UK, pp 455–463
Mininni G, Sciotti A, Martelli F (2018) Characterization and management of excavated soil and rock. In: SETAC Europe (ed) SETAC Europe 28th Annual Meeting, Responsible and Innovative Research for Environmental Quality. Society of Environmental Toxicology and Chemistry Europe (SETAC Europe) Abstract book. p 96 https://rome.setac.org/wp-content/uploads/2018/04/abstract-book_scientific-part_FINAL-cover-3.pdf. Accessed 2 Mar 2020
Murr R, Cordes T, Hofmann M, Bergmeister K (2019) Autarkic aggregate supply with recycled tunnel spoil at the Brenner Base Tunnel. In: Peila D, Viggiani G, Celestino T (eds) Tunnels and underground cities. Engineering and innovation meet archaeology, architecture and art. Proceedings of the WTC 2019 ITA-AITES World Tunnel Congress (WTC 2019). CRC Press, Taylor and Francis group, London, UK, pp 495–504
OECD (2006) Test No. 208: terrestrial plant test: seedling emergence and seedling growth test. OECD Guidelines for the Testing of Chemicals, Section 2. OECD Publishing, Paris
OECD (1984) Test No. 207: earthworm, acute toxicity tests. OECD Guidelines for the Testing of Chemicals, Section 2. OECD Publishing, Paris
Ortiz de García SA, Pinto Pinto G, García-Encina PA, Irusta-Mata R (2014) Ecotoxicity and environmental risk assessment of pharmaceuticals and personal care products in aquatic environments and wastewater treatment plants. Ecotoxicology 23:1517–1533. https://doi.org/10.1007/s10646-014-1293-8
Padulosi S, Martelli F, Sciotti A, et al. (2019) Environmental risk assessment of conditioned soil: some Italian case studies. In: Peila D, Viggiani G, Celestino T (eds) Tunnels and underground cities. Engineering and innovation meet archaeology, architecture and art. Proceedings of the WTC 2019 ITA-AITES World Tunnel Congress (WTC 2019). CRC Press, Taylor and Francis group, London, UK, pp 505–514
Parvez S, Venkataraman C, Mukherji S (2006) A review on advantages of implementing luminescence inhibition test (Vibrio fischeri) for acute toxicity prediction of chemicals. Environ Int 32:265–268. https://doi.org/10.1016/j.envint.2005.08.022
Peila D (2014) Soil conditioning for EPB shield tunnelling. KSCE J Civ Eng 18:831–836. https://doi.org/10.1007/s12205-014-0023-3
Peila D, Oggeri C, Vinai R (2007) Screw conveyor device for laboratory tests on conditioned soil for EPB tunneling operations. J Geotech Geoenvironmental Eng 133:622–1625. https://doi.org/10.1061/(ASCE)1090-0241
Peila D, Picchio A, Martinelli D, Negro ED (2016) Laboratory tests on soil conditioning of clayey soil. Acta Geotech 11:1061–1074. https://doi.org/10.1007/s11440-015-0406-8
Reemtsma T, Putschew A, Jekel M (1999) Industrial wastewater analysis: a toxicity-directed approach. Waste Manag 19:181–188. https://doi.org/10.1016/S0956-053X(99)00011-2
Rosen G, Osorio-Robayo A, Rivera-Duarte I, Lapota D (2008) Comparison of bioluminescent Dinoflagellate (QwikLite) and Bacterial (Microtox) rapid bioassays for the detection of metal and ammonia toxicity. Arch Environ Contam Toxicol 54:606–611. https://doi.org/10.1007/s00244-007-9068-3
Salizzato M, Pavoni B, Volpi Ghirardini A, Ghetti PF (1998) Sediment toxicity measured using Vibrio fischeri as related to the concentrations of organic (PCBs, PAHs) and inorganic (metals, sulphur) pollutants. Chemosphere 36:2949–2968. https://doi.org/10.1016/S0045-6535(98)00001-0
Serafim A, Company R, Lopes B et al. (2013) Evaluation of sediment toxicity in different Portuguese estuaries: ecological impact of metals and polycyclic aromatic hydrocarbons. Estuar Coast Shelf Sci 130:30–41. https://doi.org/10.1016/j.ecss.2013.04.018
Talebi K, Memarian H, Rostami J, Alavi Gharahbagh E (2015) Modeling of soil movement in the screw conveyor of the earth pressure balance machines (EPBM) using computational fluid dynamics. Tunn Undergr Sp Technol 47:136–142. https://doi.org/10.1016/j.tust.2014.12.008
Thomas DJL, Tyrrel SF, Smith R, Farrow S (2009) Bioassays for the evaluation of landfill leachate toxicity. J Toxicol Environ Heal Part B 12:83–105. https://doi.org/10.1080/10937400802545292
Thompson M, Stephen ELR, Wood R (2002) Harmonised guidelines for the in-house validation of methods of analysis (IUPAC technical report). Pure Appl Chem 74:835–855
Tsybulskii IE, Sazykina MA (2010) New biosensors for assessment of environmental toxicity based on marine luminescent bacteria. Appl Biochem Microbiol 46:505–510. https://doi.org/10.1134/S0003683810050078
UNI EN ISO (2019) Water quality—determination of the inhibitory effect of water samples on the light emission of Vibrio fischeri (Luminescent bacteria test)—part 3: method using freeze-dried bacteria. UNI EN ISO 11348-3:2019
UNI EN (2004) Characterisation of waste—leaching—compliance test for leaching of granular waste materials and sludge UNI EN 12457-2:2004
UNI EN (2005) Characterization of waste—preparation of waste samples for ecotoxicity tests. UNI EN 14735:2005
Vinai R, Oggeri C, Peila D (2008) Soil conditioning of sand for EPB applications: a laboratory research. Tunn Undergr Sp Technol 23:308–317. https://doi.org/10.1016/j.tust.2007.04.010
Volpi Ghirardini A, Girardini M, Marchetto D, Pantani C (2009) Microtox® solid phase test: effect of diluent used in toxicity test. Ecotoxicol Environ Saf 72:851–861. https://doi.org/10.1016/j.ecoenv.2008.01.011
Wang C, Yediler A, Lienert D, Wang Z, Kettrup A (2002) Toxicity evaluation of reactive dyestuffs, auxiliaries and selected effluents in textile finishing industry to luminescent bacteria Vibrio fischeri. Chemosphere 46:339–344. https://doi.org/10.1016/S0045-6535(01)00086-8
Wieczerzak M, Namieśnik J, Kudłak B (2016) Bioassays as one of the Green Chemistry tools for assessing environmental quality: a review. Environ Int 94:341–361. https://doi.org/10.1016/j.envint.2016.05.017
Yang X, Ji Y, Wang F et al. (2016) Comparison of organics and heavy metals acute toxicities to Vibrio fischeri. J Serbian Chem Soc 81:697–705. https://doi.org/10.2298/JSC151124011Y
Ying G-G (2006) Fate, behavior and effects of surfactants and their degradation products in the environment. Environ Int 32:417–431. https://doi.org/10.1016/j.envint.2005.07.004
Acknowledgements
The authors would like to thank the Department of Environment, Land and Infrastructure Engineering (DIATI) at Polytechnic University of Turin (Daniele Peila, Carmine Todaro and Daniele Martinelli) for providing the TR values of the foaming agents. We also thank Gian Luigi Garbini, Martina Di Lenola, Laura Dejana, Francesco Di Nezio, Tommaso Mella and Tanita Pescatore (IRSA-CNR), Francesca Spataro and Nicoletta Ademollo (ISP-CNR) for assisting with the analyses.
Funding
This work was funded by the Italian Company Autostrade Spa—Project N. 100682.
Author information
Authors and Affiliations
Corresponding author
Ethics declarations
Conflict of interest
The authors declare that they have no conflict of interest.
Ethical approval
This article does not contain any research using humans or (vertebrate) animals performed by any of the authors and it is in compliance with ethical standards.
Additional information
Publisher’s note Springer Nature remains neutral with regard to jurisdictional claims in published maps and institutional affiliations.
Rights and permissions
About this article
Cite this article
Mariani, L., Grenni, P., Barra Caracciolo, A. et al. Toxic response of the bacterium Vibrio fischeri to sodium lauryl ether sulphate residues in excavated soils. Ecotoxicology 29, 815–824 (2020). https://doi.org/10.1007/s10646-020-02202-7
Accepted:
Published:
Issue Date:
DOI: https://doi.org/10.1007/s10646-020-02202-7