Abstract
Application of endosulfan tolerant rhizospheric bacterial strain isolated from pesticide contaminated area, Ghaziabad in combination with V. zizanioides for the remediation of endosulfan is described herein. The dissipation of endosulfan from soil was considerably enhanced in the presence of bacterial strain and Vetiveria zizanioides together when compared to the dissipation in presence of either of them alone. Four strains— EAG-EC-12 (M1), EAG-EC-13(M2), EAG-EC-14(M3) and EAG-EC-15(M4) are used for this purpose. V. zizanioides was grown in garden soil spiked with 1500 µg g–1 of endosulfan and inoculated with 100 ml of microbial culture of above motioned strains. Effect of microbial inoculation on plant growth, endosulfan uptake and endosulfan removal efficiency were analyzed. The microbial inoculation significantly enhances the growth of test plant and endosulfan dissipation from soil (p < 0.05). The addition of bacterial strain M1, M2, M3 and M4 in treated pots showed enhanced root length by 13, 33 35, 20.2 and 4.3 %, above ground plant length by 16.38, 35.56, 24.92 and 9.8 % and biomass by 33.69, 49.63, 39.24 and 17.09 % respectively when compared with endosulfan treated plants. After 135 days of exposure, a decline in endosulfan concentration by 59.12, 64.56, 62.69 and 56.39 % was obtained in the spiked soil inoculated with bacterial strains M1, M2, M3 and M4 respectively whereas, decrease in endosulfan concentration by 72.78, 85.25, 76.91 and 65.44 % in the vegetative spiked soil inoculated with same strains was observed during same exposure period. After 135 days of growth period, enhanced removal of endosulfan from experimental soil by 13.66, 20.69, 14.22 and 9.05 % was found in vegetative experiment inoculated with same strains when compared with non vegetative experiment. Result of the study showed that use of toletant plant and tolerant bacterial strains could be the better strategy for the remediation of endosulfan contaminated soil.
Similar content being viewed by others
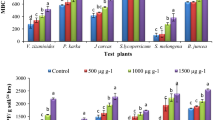
Explore related subjects
Discover the latest articles, news and stories from top researchers in related subjects.Avoid common mistakes on your manuscript.
Introduction
In present study, among various chlorinated pesticides, intensive attention has been given to endosulfan because it is not only highly toxic but also widely used in large quantities in many countries as a broad-spectrum insecticide. Due to widespread use, the isomers and metabolites of endosulfan have penetrated into almost all ecosystems, which threaten the human health and cause imbalance in natural processes. Apart from its capacity to directly damage the nervous system, endosulfan also acts as an endocrine disruptor, even low levels of exposure during pregnancy could result in various forms of endocrine disrupting effects in the offspring, including mental retardation, reproductive organ anomalies, developmental disorders, behavioural disorders later in life etc. (US-EPA 2002; Agency of Toxic Substances and Disease Registry, Toxicological Profile for Endosulfan 2000). At present, India is the largest producer and consumer of endosulfan in the world and due to its incessant use and messy industrial production, endosulfan contaminated soils are widespread in the country (Bhadauria et al. 2012; Devi et al. 2013). Residues of endosulfan have been detected in areas far distant from where it has been used, which indicates its long-range environmental transport. The half-life of endosulfan in soil varies from 60 days (alpha-endosulfan) to 800 days (beta-endosulfan). The persistence increases by acidic conditions, while in water it varies from 35 to 150 days. Endosulfan is highly insoluble in water; hence, mostly it is associated with soil particles. Soil contaminates with endosulfan mainly by the application in agricultural fields. Long after use of the endosulfan, these chemicals remain in the soil and sediments where they can enter the food chain directly. Therefore, the contaminated soil sites serve as a sink of these pesticides. So, there is an urgent need to develop cost effective, eco friendly and sustainable technology for removal or detoxification of these contaminants from degraded soil.
The in situ remediation of persistent organic pollutants (POPs) is difficult as the contaminant is tightly bound in soil matrix. Bioremediation can be applied in situ without the removal and transport of polluted soil and disturbance of the soil matrix. For making this technique meaningful, suitable bacterial strain need to be inoculated that can thrive in the contaminated soil and degrade the target compounds (Panno et al. 2005). Phytoremediation is a cost effective and innovative technology that uses plants to clean up a broad range of organic and inorganic wastes (Warsaw et al. 2012; Ibrahim et al. 2013; Li et al. 2014). Without microbial contribution, phytoremediation alone may not be a viable technology for many hydrophobic organic pollutants (Chaudhry et al. 2005). To overcome the possible restrictions on plant growth, tolerant rhizospheric bacterial strains have been used to detoxify contaminants that occur in close proximity to the plant (Huang et al. 2005; Epelde et al. 2009). Use of tolerant plant along with rhizospheric tolerant bacterial strains enhances the survival of microbes by secreting root exudates which helps the strains in effectively detoxify contaminants in close proximity of the roots.
The grass varieties were reported to be the most suitable plant species for rhizosphere bioremediation of organic contaminants due to their ability to harbour large number of bacteria on their highly branched root system (Kidd et al. 2008; Datta et al. 2013). In our previous study, we found that V. zizanioides can accumulate considerable amount of endosulfan in their tissue (Singh and Singh 2014) and these selected strains can tolerate high concentration of endosulfan. The aim of the present study was to examine the dissipation of endosulfan by V. zizanioides along with microbial isolates M1, M2, M3 and M4.
Materials and methods
Isolation and characterization of bacterial strains
Bacterial isolation was carried out by following the serial dilution method using minimal salt medium (composition: 7 g dipotassium phosphate, 2 g monopotassium phosphate, 0.5 g sodium citrate, 1 g ammonium sulfate, 0.1 g magnesium sulfate in 1 L medium pH 7.0 ± 0.2) and then incubated in an orbital shaker, at 37 °C and 150 rpm. Plates were inoculated at 37 °C for 24 h. After 24 h of inoculation, the isolated pure colonies developed on the agar plates. Separate colonies were picked up for the purification of bacteria by streaking method on MSM agar plates supplemented with 1500 µg mL−1 endosulfan. Subsequently, four endosulfan degrading bacterial strains coaded as EAG-EC-12, EAG-EC-13, EAG-EC-14 and EAG-EC-15 were also used. For identification of endosulfan degrading bacterial strains on the basis of 16s ribosomal RNA technology, they were grown in nutrient broth separately for overnight and pellets were formed by centrifugation at 15,000 gX 15 min for RNA extraction. These strains were identified on the basis of their homology (>99 %) of RNA sequence with NCBI database of bacteria following 16s RNA technology. The NCBI accession no. for these strains: EAG-EC-12 (KU886044), EAG-EC-13 (KU886045), EAG-EC-14 (KU886046) and EAG-EC-15 (KU886047). For convenience the EAG-EC-12,EAG-EC-13, EAG-EC-14 and EAG-EC-15 strains in this paper are coded as M1, M2, M3 and M4 respectively.
Inoculum preparation for degradation study
The inocula of the bacterial strains for all experiments were prepared by incubating these bacterial strains separately in nutrient broth for 4 days at 28 °C on shaker at 150 rpm. Cell pellet was collected by centrifugation of cell culture at 4 °C and 10,000 rpm for 10 min. Cells were washed 3 times with 25 mL of sterile 12.5 mM phosphate buffer (pH 7.2) and quantified by dilution plate count technique. For pot culture experiment, 106 cells were added per gm of soil.
Experimental design
Pot experiment was performed in green house for 135 days. A stock solution of endosulfan was prepared with hexane in order to obtain a concentration of endosulfan at 1500 µg g−1. Two sets of experiment namely vegetative and non-vegetative were carried out simultaneously. The experimental setup illustrated in Fig. 1.
Non vegetative experiment
Eight inches earthen pots were filled with endosulfan spiked soil and inoculated with 100 mL each of M1, M2, M3 and M4 strains. Besides, control treatments were also set up to calculate the loss of endosulfan through environmental factors and through native microbes. Three sets of control include: (i) Non spiked soil without inoculums (S); (ii) spiked soil without inoculums (S+E); and (iii) Spiked soil sterilized with HgCl2 to kill native microbes (S+E+HgCl2).
Vegetative experiment
Rhizomes of V. zizanioides were first grown in vermiculite and after 2 weeks, the V. zizanioides saplings were transplanted in each pot. Four treatments were given, that included the endosulfan spiked soil with V. zizanioides and above mentioned bacterial strains (M1, M2, M3 and M4). Similar to non vegetative experiment, additional control experiments were also set up. Three sets of control include: (i) Non spiked vegetated soil without inoculums (S + P); (ii) Spiked vegetated soil without inoculums (S+P+E); and (iii) Spiked vegetated soil sterilized with HgCl2 (S+P+E+HgCl2).
Nine replicates of each treatment were taken. Three samplings were done at 45, 90 and 135 days of exposure. Three replicates of each treatment were harvested during each sampling. Morphological parameters of experimental plant were measured during each harvesting. Remaining amount of endosulfan concentrations in experimental soil were detected by the method of Anastassiades et al. (2003).
Instrumentation and operating conditions
Endosulfan isomers and its metabolite concentrations were analyzed on a Perkin Elmer (Norwalk, CT, USA) gas chromatograph Model Clarus 500 series equipped with a 63Ni electron capture detector (ECD) system. A split/split less injector was used in a split less mode, applying injection volume of 1 µL. For separation a 35 % diphenyl and 65 % dimethyl polysiloxane capillary column (30 mX 0.32 mm ID X 0.5 µm) Elite 5 (Perkin Elmer, USA) was employed. The injection temperature was 250 °C and detector temperature was 300 °C. Nitrogen (N2) of purity greater than 99.999 % was used as carrier gas at programmed flow of 1.0 mL/min. Oven temperature was 170 °C (2 min), an increase of 10 °C/min to 250 °C.
Statistical analysis
The experiments were carried out in triplicates and the results are presented in average ± SD. The data were subjected to one-way ANOVA (analysis of varience) and statistical significance was estimated. Duncans test was used to compare treatment with control.
Results and discussion
Effect of bacterial strains on growth of V. zizanioides
The growth of V. zizanioides is graphically presented in Fig. 2. The supplementation of microbial strains significantly (p < 0.05) enhanced the growth in terms of root length, above ground plant length and biomass of V. zizanioides in endosulfan spiked soil.
There was a significant (p < 0.05) reduction in the growth of test plants grown in endosulfan spiked soil without additional microbial input. After 135 days, with 1500 µg g−1 endosulfan, growth in terms of root length, above ground plant length and biomass exhibited 18.13, 16.80 and 32.75 % decline respectively, compared to control (without microbial input and endosulfan). The addition of bacterial strains M1, M2, M3 and M4 showed positive effects on root length which increased by 13, 33.35, 20.2 and 4.3 % respectively when compared with endosulfan treated plants. The longest root length of V. zizanioides was observed in the soil amended with strain M2 and the smallest was with strain M4. Root length of V. zizanioides amended with strain M3 was statistically similar to control (without microbial input and endosulfan). The addition of bacterial strains M1 and M4 showed decline in root length by 6.27 and 16.89 %, however, increment of root length by 18.6 and 2.53 % was observed in strain M2 and M3 amended soil when compared with control (without microbial input and endosulfan).
Similarly, the amendment of same strains (M1, M2, M3 and M4) also enhanced the above ground plant length by 16.38, 35.56, 24.92 and 9.8 % when compared with endosulfan treated plants. Similar to root length, the longest above ground plant length was also found in the strain M2 amended plant and the smallest was found in soil supplemented with strain M4. After 135 days, above ground length of test plant grown in strain M1 amended soil was statistically similar (p < 0.05) to control (without microbial input and endosulfan). Increment in above ground length of test plant grown in strain M2 and M3 amended soil by 22.46 and 9.74 % were found however, decline by 7.76 % in above ground length of test plant grown in strain M4 amended soil was observed when compared with control (without microbial input and endosulfan).
A significant (p < 0.05) enhancement in the biomass of test plants were observed with microbial input when compared with endosulfan treated plants. Increase in biomass by 33.69, 49.63, 39.24 and 17.09 % respectively was observed with the addition of bacterial strains (M1, M2, M3 and M4) when compared with endosulfan treated plants. Biomass of test plant amended with strain M1 and M3 was statistically similar to control (without microbial input and endosulfan). An increment in biomass of test plant grown in strain M2 amended soil by 25.1 % was found and decline in biomass by 18.9 % was observed in test plant grown in strain M4 amended soil when compared with control (without microbial input and endosulfan). Based on the results shown in Fig. 2, the V. zizanioides inoculated with strains M1, M2, M3 and M4 exhibited increased root, above ground length and biomass compared with spiked uninoculated V. zizanioides. It was evidenced that V. zizanioides and inoculated strains (M1, M2, M3 and M4) had an efficient ability to increase the stress tolerance to endosulfan. There are several ways in which rhizospheric bacteria can directly facilitate the growth of plant. They may fix atmospheric nitrogen and supply it to plants; help in synthesizing phytohormones such as auxins, cytokinins and gibberellins which can enhance plant growth; and synthesize the enzyme 1-aminocyclopropane-1-carboxylate (ACC) deaminase which lowers plant ethylene levels and helps in survival of plant against any stress (Glick et al. 2007; Glick and Stearns 2011).
Removal of endosulfan from soil
The residual quantity of endosulfan in soil was determined to analyze the effect of V. zizanioides and bacterial inoculation on endosulfan degradation in soil. Data obtained from the study provides a comparison between the spiked, non vegetative, inoculated and vegetative, spiked and inoculated treatments (Fig. 3). A gradual decline in the concentration of endosulfan with time was observed in almost all the treatments at endosulfan concentration of 1500 µg g−1 In the treatments with added bacterial strains viz. S+E+M1….M4 and S+P+E+M1……M4, the effect was quit significant and a sharp decline (p < 0 0.05) in endosulfan concentration was observed at the end of incubation period as compared to respective control (S+E and S+P+E).
Non vegetative experiment
The unspiked control (S) had 0.06 µg g−1of endosulfan was observed. This slight amount may be due to the deposition of volatilized endosulfan from other sources. In the uninoculated, spiked soil (S+E), decline in endosulfan residue in soil was observed by 5.24, 11.73 and 18.8 % respectively at 45, 90 and 135 days of growth period. This decrease in endosulfan concentration may be due to both degradation by native microflora of soil, physico-chemical (volatilization and photodegradation) and environmental factors like temperature, pH and moisture content (Singh et al. 2004). In the spiked soil sterilized by HgCl2, decrease in endosulfan residue was found by 3, 7.1 and 14.66 % respectively on 45, 90 and 135 days of exposure. This decrease in endosulfan concentration may be due to physico-chemical and environmental factors only because indigenous microorganisms were killed due to HgCl2 treatment. From the result, it was observed that after 135 days of incubation period 13.66 % endosulfan was removed by environmental factors and 4.14 % endosulfan was degraded by native microbes present in soil.
A decrease in endosulfan concentration in the spiked soil having bacterial inputs with increasing incubation period was observed. In the spiked soil inoculated with bacterial inoculums (M1, M2, M3 and M4), the highest degree of removal of endosulfan was obtained with strain M2 and the lowest with strain M4 at all incubation periods. After 135 days of exposure, a decline in endosulfan concentration by 59.12, 64.56, 62.69 and 56.39 % was obtained in the spiked soil inoculated with bacterial strains M1, M2, M3 and M4 respectively. The endosulfan degradation potential of screened bacterial strains was found in the order M2 > M3 > M1 > M4.
Degradation of pesticides refers to the breakdown of pesticides within environment. The degradation may refer through photodegradation, chemical degradation and biodegradation. Biodegradation of endosulfan in soil was studied by (Odukkathil and Vasudevan 2013) in four bacterial isolates, capable of growing on endosulfan as sole carbon source. They reported decline in endosulfan concentration of up to 69 % in soil containing 200 µg g−1 endosulfan after 40 days of incubation in presence of each individual bacterial isolate. In another study, authors have found that the bacterium Pseudomonas sp. was highly efficient in degrading endosulfan (Panno et al. 2005). However, in the present study, the maximum degradation observed was 71.92 % (spiked soil inoculated with strain M2). This difference in the results may be due to several reasons including the efficiency of the isolates, the type of soil used for the study and the concentration of endosulfan used. Endosulfsan is an organic pesticide having carbon and sulfur in its molecular composition. Presence of endosulfan concentration in soil provides additional carbon source to microbes, which cause proliferation of microorganism using carbon and sulfur of endosulfan as nutrient source and biodegrade endosulfan to either its non toxic forms (endosulfan diol and endosulfan lactone) or end products CO2 and H2O.
Vegetative experiment
In the vegetative spiked and uninoculated soil (S+P+E), removal of endosulfan was 12.07, 20.95 and 29.35 % after 45, 90 and 135 days of experiment respectively. Compared to non vegetative spiked uninoculated soil (S+E) the removal of endosulfan by S+P+E was significantly (95 % confidence level) increased by 6.83, 9.22 and 10.55 % after 45, 90 and 135 days respectively. Removal of endosulfan from soil by phytomass of Ocimum basilicum was reported by Ramirez-Sandoval et al. (2011). They reported a decrease in endosulfan concentration up to 37 % in soil containing 1000 mg kg−1 endosulfan after 90 days of plant growth. In another study, removal of endosulfan from soil by Brassica juncea was reported up to 14.5 % (Mukherjee and Kumar 2012).
In the case of spiked, vegetative but sterilized soil (S+P+E+HgCl2) removal of endosulfan was 5.36, 12.69 and 23.16 % after 45, 90 and 135 days of plant growth. In this case significant percentage of endosulfan 6.36, 14.62 and 25.39 % removal was recorded when compared to same on non vegetative experiment. This increase in removal of endosulfan in sterilized, vegetative, spiked soil is due to root exudates secreted by V. zizanioides which enhanced the degradation of endosulfan (Sicilliano et al. 2003; Nelson 2004). The main reason for the improved degradation of endosulfan in present study is presumably the increase in the number and metabolic activity of microbes in the rhizosphere through exudates secretion by V. zizanioides roots. Plant root exudates contain sugars, organic acids, and amino acids as main components (Vancura and Hovadik 1965). The exudation of nutrients by V. zizanioides roots creates a nutrient-rich environment in which bacterial activity is stimulated. Bacteria able to use these chemicals as carbon and/or energy sources often have enzymes that can (co-) metabolise pollutants with similar structures. Thus, the degradation of several chlorinated pesticides has been reported to be higher in a vegetated soil than a non-vegetated soil (Kingsley et al. 1994; Kuiper et al. 2001; Abhilash et al. 2011).
Similar to non vegetative, spiked soil, in vegetative spiked soil inoculated with bacterial strains M1, M2, M3 and M4, the highest degree of removal of endosulfan was observed with strain M2 and the lowest with M4 at periods. A decrease in endosulfan concentration in vegetative spiked soil having bacterial inputs with increasing growth period was observed. The retardation of endosulfan was more in vegetative soil than in non-vegetative. After 135 days of exposure, a decline in endosulfan concentration increased to 72.78, 85.25, 76.91 and 65.44 % in the vegetative spiked soil inoculated with bacterial strains M1, M2, M3 and M4 respectively. The endosulfan degradation potential of bacterial strains and V. zizanioides in combination followed the similar trend as non vegetative spiked soil inoculated with bacterial input i.e. M2 > M3 > M1 > M4. In vegetated pots, an increase in endosulfan removal by 13.66, 20.69, 14.22 and 9.05 % was reported as compared to non vegetated, spiked pots containing microbial inoculums.
Normally plants mediate their effect indirectly via rhizodeposits, which attract active and diverse group of microorganisms thereby facilitating the biodegradation process (Kuiper et al. 2004). The rhizosphere effect gets more prominent only after development of well-defined root zone and colonization of the roots by different group of microorganisms, a process which is slow and time taking (Sicilliano et al. 2003; Kuiper et al. 2004).
Few workers have reported the biodegradation of pesticides in soil in presence of externally added degraders. Singh et al. (2004) studied the biodegradation of chlorpyrifos (an organophosphate pesticide) in soil by an Enterobactor sp. the bacterium was capable of degrading up to 48 % chlorpyrifos within 3 days with the formation of 3, 5, 6-trichloro-2-pyridinol and diethyl thiophosphate as metabolites. They also reported that a high inoculum density facilitates biodegradation. Results of the present study agree well with those of Rani and Juwarkar (2012). In their study they reported, 14 % enhanced phorate degradation by utilizing B. juncea and externally aided bacterial strains in comparison with the bacterial consortium alone. Becerra-Castro et al. (2013) have also reported enhanced HCH dissipation by the use of Cytisus striatus in combination with strains Rhodococcus erythropolis and Sphingomonas species.
Microbial degradation has been reported to be a promising way to alleviate anthropogenic compounds from the environment (Rani and Juwarkar 2012). The supplementation of microbial isolates results in the enhanced growth of test plants, which in turn results in an enhanced removal of organic contaminants from soil (Ontanon et al. 2014; Shahsavari et al. 2015). The growing plants release plant root exudates, mucigel and root lysates (enzymes, amino acids, carbohydrates, low molecular mass carboxylic acids, flavonones and phenoloics) (Kidd et al. 2008) which support the growth, multiplication and degradation potential of associated microbes (Korade and Fulekar 2009). Efficient remediation of organic pollutants contaminated sites by combined use of tolerant plants and microbes has been reported by Korade and Fulekar (2009); Ahamed et al. (2012) and Becerra-Castro et al. (2013).
In the present study, V. zizanioides played a supportive role in the degradation of endosulfan rich soil hence it is competent for rhizosphere bioremediation of endosulfan in soil, which may be credited to its advantageous features of having fibrous root system providing large specific surface area to interact with microorganism and capacity to release high amount of exudates in the rhizosphere (Datta et al. 2013). Results from vegetative experiments are of higher importance because these show the effectiveness of symbiosis between microbes and plant over the effectiveness of the microorganism alone for removal of endosulfan.
Formation of metabolites of endosulfan
Metabolism of endosulfan results in the formation of transformation products of endosulfan. Endosulfan is first metabolized to endosulfan sulfate, or endosulfan diol, followed by further degradation from endosulfan diol to endosulfan ether or endosulfan lactone (Xie et al. 2011). In the present study, endosulfan sulfate, endosulfan diol and endosulfan lactone was formed (Figs. 4, 5, 6), which shows the degradation of endosulfan to its non toxic form. It was reported that some bacterial species could completely utilize the hydrolytic pathway (Hussain et al. 2007).
In the present study, formation of endosulfan metabolites (endosulfan sulfate, endosulfan diol and endosulfan lactone) evidenced that both oxidative and hydrolytic pathways rhizospheric microbes to degrade the toxicant. Endosulfan sulfate is the major and toxic metabolite of endosulfan (Kataoka and Takagi 2013; Silambarasan and Abraham 2014), it is formed by the oxidation of endosulfan in presence of endosulfan sulfate monooxygenase I enzyme (Hussain et al. 2007). In this study, it was observed that endosulfan sulfate was found in all the samples except in sterilized and untreated soil.
After 135 days of growth, endosulfan sulfate ranged 4.12–13.11 µg g−1 in the non vegetative soil samples from all the treatments while it ranged 5.22–19.45 µg g−1 in the vegetative soil samples. Maximum endosulfan sulfate (19.45 µg g−1) was found in the vegetative spiked soil inoculated with strain M2 whereas minimum (4.12 µg g−1) with spiked, non vegetative soil. Endosulfan sulfate being more toxic than parent compound (Kumar et al. 2008), needs to be degraded to facilitate the production of less toxic or non toxic metabolites (endosulfan diol and endosulfan lactone). The result of the study, suggested that the S–O bond of endosulfan is cleaved, favouring the production of non sulfur containing molecules like endosulfan diol, that can be easily degraded into endosulfan ether or endosulfan lactone. Endosulfan diol is a non toxic metabolite of endosulfan formed through the hydrolytic pathway of biodegradation (Hussain et al. 2007) and it was found in the range of 2.83–16.19 µg g−1 in the spiked, non vegetative and inoculated soil. However, it ranged 14.77–43.14 µg g−1 in the vegetative, spiked and inoculated soil (S+P+E+M1….M4).
Similar to endosulfan sulfate, maximum endosulfan diol concentration was found in the spiked, vegetative soil inoculated with strain M2 while it was found minimum in the spiked, non vegetative soil inoculated with strain M4. Residual endosulfan diol in soil ranged from 2.83 to 16.19 µg g−1 in the spiked non vegetative soil inoculated with strains M1, M2, M3 and M4 after same growth period. However it ranged from 14.77 to 43.14 µg g−1 in the spiked vegetative and inoculated soil (M1, M2, M3 and M4).
After 135 days of growth period, endosulfan lactone was found in the range of 2.15–5.06 µg g−1 was with the non vegetative, spiked inoculated soil while in the vegetative, spiked and inoculated soil, it was found in the range of 7.46–14.5 µg g−1. Similar to endosulfan sulfate, maximum endosulfan lactone residue (14.5 µg g−1) was found in the vegetative soil inoculated with strain M2 whereas minimum (2.15 µg g−1) was found in the non vegetative soil inoculated with strain M4. These observations were almost same like the earlier reports (Katayama and Matsumura 1993), wherein the microbial metabolism of endosulfan isomers was found to be accompanied with the formation of endosulfan sulfate alongwith other metabolites. Martens (1976) reported formation of endosulfan sulfate, diol, hydroxy ether and lactone as the degradation products of endosulfan. Kullman and Matsumura (1996) studied the metabolic pathway of Phanerocheate chrysosporium for the degradation of endosulfan. They reported that this organism utilized both oxidative and hydrolytic pathway for the dagradation of endosulfan and identified endosulfan sulfate, endosulfan diol, and endosulfan hydroxyether in the culture. Katayama and Matsumura (1993) showed that the static cultures of Trichoderma harzianum were capable of producing endosulfan diol as the principal metabolite. They suggested that a hydrolytic enzyme sulfatase is responsible for the indirect formation of endosulfan diol by the hydrolysis of endosulfan sulfate.From the present study, it was clear that rhizospheric bacterial population in soil played very important role in the degradation of endosulfan to its metabolites. It was well known that in soil, endosulfan could first be converted to either endosulfan sulfate or endosulfan diol, and these two derivatives are then degraded seperately (Kumar et al. 2008). V. zizanioides has supportive role in the biotransformation of endosulfan into its metabolites due to highly developed root system with large surface area and deep root penetration. Exudates secreted by V. zizanioides roots helped in the proliferation of microbes in the rhizosphere and these microbes have biotransform endosulfan into its non toxic forms. Although the mechanism of biotransformation of endosulfan was not exhibited in this research.
Conclusion
Recently, endosulfan has been proposed to be included in the Stockholm list of (POPs) for global elimination. Therefore, invasive remediation measures are of utmost importance for the cleanup of already contaminated sites. The present study suggests that phytoremediation using rhizospheric microbes is a promising approach for the in situ remediation of endosulfan contaminated soils. The rhizospheric microbes were supporting the growth of test plants and dissipation of endosulfan in soil. Although the mode of association of rhizospheric microbes and V. zizanioides was not elucidated in this research, there may be several possibilities for an enhanced remediation including growth promoting potential of rhizospheric microbes, secretion of exudates and catabolic enzymes by V. zizanioides. Formation of non toxic metabolites in present study also supports that; utilization of these microbes with V. zizanioides will be very valuable in the bioremediation of endosulfan contaminated soils/sites. Selection of plant–microbial associate where the competitiveness of the microbial partner is improved through a “nutritional bias” caused by exudates available to this partner may open new horizons for rhizodegradation of organically polluted soils. An improved understanding of the rhizosphere will help to convert the results of simplified bench scale and pot experiments to field applications; however detailed studies on plant microbe interaction needed to validate effectiveness of rhizoremediation.
References
Abhilash PC, Srivastava S, Srivastava P, Singh B, Jafri A, Singh N (2011) Influence of rhizospheric microbial inoculation and tolerant plant species on the rhizoremediation of lindane. Environ Exp Bot 74:127–130
Agency of Toxic Substances and Disease Registry, Toxicological Profile for Endosulfan, 2000
Ahamed GJ, Wang MM, Zhou YH, Xia XJ, Mao WH, Shi K (2012) The growth, photosynthesis and antioxidant defense responses of five vegetable crops to phenanthrene stress. Ecotoxicol Environ Saf 80:132–139
Anastassiades M, Lehotay S, Štajnbaher D, Schenck F (2003) Fast and easy multiresidue method employing acetonitrile extraction/partitioning and dispersive solidphase extraction for the determination of pesticide residues in produce. J. AOAC Int 86(22):412–431
Becerra-Castro C, Kidd PS, Rodríguez-Garrido B, Monterroso C, Santos-Ucha P, Prieto-Fernández A (2013) Phytoremediation of hexachlorocyclohexane (HCH)-contaminated soils using Cytisus striatus and bacterial inoculants in soils with distinct organic matter content. Environ Pollut 178:202–210
Bhadauria BS, Mathur VB, Kaul R (2012) Monitoring of organochlorine pesticides in and around Keoladeo National Park, Bharatpur, Rajasthan, India. Environ Monit Assess 184:5295–5300
Chaudhry Q, Blom-Zandstra M, Gupta S, Joner EJ (2005) Utilizing the synergy between plants and rhizosphere microorganisms to enhance breakdown of organic pollutants in the environment. Environ Sci Pollut Res 12:34–48
Datta R, Das P, Smith S, Pravin Punamiya P, Ramanathan DM, Reddy R, Sarkar D (2013) Phytoremediation potential of vetiver grass [Chrysopogon zizanioides (L.)] for tetracycline. Int J Phytoremediation 15(4):343–351
Devi N, Chakraborty P, Shihua Q, Zhang G (2013) Selected organochlorine pesticides (OCPs) in surface soils from three major states from the north eastern part of India. Environ Monit Assess 185(8):6667–6676
Epelde L, Mijangos I, Becerril JM, Garbisu C (2009) Soil microbial community as bioindicator of the recovery of soil functioning derived from metal phytoextraction with sorghum. Soil Biol Biochem 41(9):1788–1794
Glick BR, Stearns JC (2011) Making phytoremediation work better: maximizing a plant’s growth potential in the midst of adversity. Int J Phytorem 13(1):4–16
Glick BR, Cheng Z, Czarny J, Duan J (2007) Promotion of plant growth by ACC deaminase-producing soil bacteria. Eur J Plant Pathol 119:329–339
Huang XD, EI-Alawi Y, Penrose DM, Glick BR, Greenberg BM (2005) A multi-process phytoremediation system for removal of polyaromatic hydrocarbons from contaminated soils. Environ Pollut 130:465–476
Hussain S, Arshad M, Saleem M, Khalid A (2007) Biodegradation of alpha and beta endosulfan by soil bacteria. Biodegradation 18:731–740
Ibrahim SI, Lateef MFA, Khalifa HMS, Monem AEA (2013) Phytoremediation of atrazine-contaminated soil using Zea mays (maize). Annals of Agriculture Science 58(1):69–75
Kataoka R, Takagi K (2013) Biodegradability and biodegradation pathways of endosulfan and endosulfan sulfate. Appl Microbiol Biotechnol 97:3285–3292
Katayama A, Matsumura F (1993) Degradation of organochlorine pesticides particularly endosulfan by Trichoderm Harzianum. Environ Toxicol Chem 12:1059–1065
Kidd PS, Prieto-Fernandez A, Monterroso C (2008) Rhizospheric microbial community and hexachlorocyclohexane degradative potential in contrasting plant species. Plant Soil 32:233–247
Kingsley MT, Fredrickson JK, Metting FB, Seidler RJ (1994) Environmental restoration using plant-microbe bioaugmentation. In: Hinchee RE, Leeson A, Semprini L, Ong SK (eds) Bioremediation of chlorinated and polyaromatic hydrocarbon compounds. Lewis Publishers, Boca Raton, FL, pp 287–292
Korade DL, Fulekar MH (2009) Rhizosphere remediation of chlorpyrifos in mycorrhizospheric soil using ryegrass. J Hazard Mater 172(2–3):1344–1350
Kuiper I, Bloemberg GV, Lugtenberg BJJ (2001) Selection of a plant-bacterium pair as a novel tool for rhizostimulation of polycyclic aromatic hydrocarbon-degrading bacteria. Mol Plant Microbe Interact 14:1197–1205
Kuiper I, Legendijk EL, Bloemberg GV, Lugtenberg BJJ (2004) Rhizoremediation: a beneficial plant-microbe interaction. Mol Plant-Microbe Interact 17:6–15
Kullman SW, Matsumura F (1996) Metabolic pathways utilized by Phanerochaete chrysosporium for degradation of the cyclodiene pesticide endosulfan. Appl Environ Microbiol 62(2):593–600
Kumar M, Lakshmi VC, Khanna S (2008) Biodegradation and bioremediation of endosulfan contaminated soil. Bioresour Technol 99:3116–3122
Li Z, Xiao H, Cheng S, Zhang L, Xie X, Wu Z (2014) A comparison on the phytoremediation ability of triazophos by different macrophytes. J Environ Sci 26(2):315–322
Martens M (1976) Degradation of [8,9–14C]endosulfan by soil microorganisms. Appl Environ Microbiol 6:853–858
Mukherjee I, Kumar A (2012) Phytoextraction of endosulfan a remediation technique. Bull Environ Contam Toxicol 88:250–254
Nelson JA, Morgan JA, LeCain DA, Mosier AR, Milchunas DG, Parton BA (2004) Elevated CO2 increases soil moisture and enhances plant water relations in a long term field study in semi-arid shortgrass steppe of Colorado. Plant Soil 259:169–179
Odukkathil G, Vasudevan N (2013) Enhanced biodegradation of endosulfan and its major metabolite endosulfate by a biosurfactant producing bacterium. J Environ Sci Health Part B 48:462–469
Ontanon OM, Gonzalez PS, Ambrosio LF, Paisio CE, Agostini E (2014) Rhizoremediation of phenol and chromium by the synergistic combination of a native bacterial strain and Brassica napushairy roots. Int Biodeterior Biodegradation 88:192–198
Panno MTD, Morelli IS, Engelen B, Berthe-corti L (2005) Effect of petrochemical sludge concentrations on microbial communities during soil bioremediation. FEMS Microbiol Ecol 53:305–316
Ramirez-Sandoval M, Melchor-Partidaa GN, Muniz-Hernandez S, Giron-Perez MI, Rojas-Garcia AE, Medina-Diaz IM, Robledo-Marenco ML, Velazquez Fernandez JB (2011) Phytoremediatery effect and growth of two species of Ocimum in endosulfan polluted soil. J Hazard Mater 192:388–392
Rani R, Juwarkar A (2012) Biodegradation of phorate in soil and rhizosphere of Brassica juncea (L.) (Indian Mustard) by a microbial consortium. Int Biodeterior Biodegradation 71:36–42
Shahsavari E, Adetutu EM, Taha M, Ball AS (2015) Rhizoremediation of phenanthrene and pyrene contaminated soil using wheat. J Environ Manage 155:171–176
Sicilliano SD, Germida JJ, Banks MK, Greer CW (2003) Changes in microbial community composition and function during polyaromatic hydrocarbom phytoremediation field trial. Appl Environ Microbiol 69:483–489
Silambarasan S, Abraham J (2014) Halophilic bacterium JSA4 in biomineralization of endosulfan and its metabolites isolated from Gossypium herbaceum rhizosphere soil. J Taiwan Inst Chem E 45(4):1748–1756
Singh N, Megharaj M, Kookana R, Naidu R, Sethunathan N (2004) Atrazine and simazine degradation in Pennnisetum rhizospher. Chemosphere 56:257–263
Singh V, Singh N (2014) Uptake and accumulation of endosulfan isomers and its metabolite endosulfan sulfate in naturally growing plants of contaminated area. Ecotoxicol Environ Saf 104:189–193
US-EPA (2002) Reregistration Eligibility Decision for Endosulfan. EPA 738-R-02-013. Pollution, Pesticides and Toxic Substances (7508C), United States Environmental Protection Agency. http://www.epa.gov/oppsrrd1/REDs/endosulfan_red.pdf
Vancura V, Hovadik A (1965) Root exudates of plants II. Composition of root exudates of some vegetables. Plant Soil 22:21–32
Warsaw AL, Fernandez RT, Kort DR, Cregg BM, Rowe B, Vandervoort C (2012) Remediation of metalaxyl, trifluralin, and nitrate from nursery runoff using container-grown woody ornamentals and phytoremediation areas. Ecol Eng 47:254–263
Xie HN, Gao FW, Tan W, Wang SG (2011) A short term study on the interaction of bacteria, fungi and endosulfan in soil microcosm. Sci Total Environ 412–413:375–379
Acknowledgments
Authors are grateful to the Director CSIR- National Botanical Research Institute (NBRI) for providing necessary facilities. Authors also wish to thank the Department of Science and Technology (DST) for financial support as DST-WOS-A project.
Author information
Authors and Affiliations
Corresponding authors
Ethics declarations
Conflict of interest
The authors declare that they have no conflict of interest.
Ethical statement
We followed ethical standards throughout the manuscript.
Rights and permissions
About this article
Cite this article
Singh, V., Singh, P. & Singh, N. Synergistic influence of Vetiveria zizanioides and selected rhizospheric microbial strains on remediation of endosulfan contaminated soil. Ecotoxicology 25, 1327–1337 (2016). https://doi.org/10.1007/s10646-016-1685-z
Accepted:
Published:
Issue Date:
DOI: https://doi.org/10.1007/s10646-016-1685-z