Abstract
The characteristics of the Atlantic salmon (Salmo salar) smolt run of the Rivière Saint-Jean, Quebec, Canada, in 2009 and 2010 were determined using acoustic telemetry. Tagged smolts were tracked from freshwater release sites for 17 km, then through the estuary and for their entry into the Gulf of St. Lawrence. The smolt migration began in both years at water temperatures of 10 °C, but lasted twice as long in the cooler year. The smolts in 2009 crossed the river to ocean boundary faster than the smolts of 2010, despite being similar in size. Smolt speed over ground increased from the river to the estuary to the marine environment. Smolts migrated both during the day and the night, but most movements began near or just after sunset, with increasingly nocturnal movements in the ocean. Smolts crossed the estuary during ebbing tides and moved faster during the night than the day. The tide cycle also influenced estuarine smolt travelling rates, but only secondarily to the diel cycle.
Similar content being viewed by others
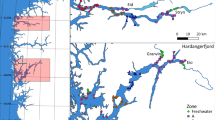
Explore related subjects
Discover the latest articles, news and stories from top researchers in related subjects.Avoid common mistakes on your manuscript.
Introduction
Anthropogenic and natural changes in freshwater, estuarine and marine environments are affecting the ecology and habitat of many diadromous fishes (Greene and Pershing 2007; Haro 2009). Since the 1970s, anadromous Atlantic salmon (Salmo salar L. 1758) populations have significantly decreased due to overfishing and the compounding effects of anthropogenic and environmental impacts such as dams, pollution and climate change (Cairns 2001; Friedland et al. 2003; Lacroix and Knox 2005; Todd et al. 2008). Despite mitigations such as hatchery supplementation, installations of fish ladders, commercial fisheries closures, and catch-and-release programs, wild Atlantic salmon numbers remain much lower than they were 40 years ago (Cairns 2001; WWF 2001).
In the smolt stage, juvenile anadromous Atlantic salmon leave freshwater for a feeding migration at sea. Presently, little is known about the timing of smolt migrations and their migration pathways (e.g., Caron 1983; Dutil and Coutu 1988; Whoriskey et al. 2008). Changes in the environment are known to affect cues used by salmon for migration and possibly their migration routes (Todd et al. 2008). Environmental variables shown to affect the smolt migration at particular sites include water temperature (Veselov et al. 1998; Antonsson and Gudjonsson 2002; Zydlewski et al. 2005), tidal cycles in the estuary (Lacroix et al. 2005) and the diel cycle (Hedger et al. 2008).
In temperate regions, smolts and post-smolts tend to favour nocturnal migrations but daylight movements can occur (Solomon 1978; Moore et al. 1995; Lacroix and McCurdy 1996; Moore et al. 1998; Ibbotson et al. 2006), while in sub-arctic regions (where the smolt run occurs under 24 h sunlight conditions) smolts travel throughout the diel cycle (Davidsen et al. 2005; Orell et al. 2007). A shift from nocturnal emigration at the beginning of the run to an increase in diurnal migration has been reported at some sites (Moore et al. 1995, 1998) possibly due to increases in water temperatures toward the end of the run (Thorpe et al. 1994; Ibbotson et al. 2006).
Most studies investigating the effect of diel rhythm on smolt movements and speeds focused only on a single migration stage, either in freshwater (Gibson and Côté 1982; Moore et al.1995; Moore et al. 1998; Davidsen et al. 2005; Ibbotson et al. 2006; Orell et al. 2007), the estuary (McCleave 1978; Moore et al. 1995, 1998; Hedger et al. 2008; Martin et al. 2009) or coastal marine areas (Lacroix and Knox 2005; Lacroix et al. 2005; Davidsen et al. 2009). Consequently we do not have good information on how smolts alter their behaviours as they shift from one environment to another. Much less work has been done on the estuarine and marine movements of smolts compared to those in fresh water. Several studies found that smolts crossed estuaries predominantly on ebbing tides (Fried et al. 1978; Moore et al. 1995; Lacroix and McCurdy 1996; Moore et al. 1998). However, there have also been reports of smolts moving upstream or holding position during flood tides (Moore et al. 1995; Lacroix and McCurdy 1996; Lacroix et al. 2005; Martin et al. 2009). Moore et al. (1998) found that smolts swam through the estuary preferentially on nocturnal ebbing tides whereas Hedger et al. (2008) found that the strongest outwardly directional movements of smolts in a coastal embayment occurred on nocturnal flooding tide. These results suggest that local conditions particular to specific sites and regions drive adaptive behavioural changes in smolt migration that cannot be easily predicted.
Here, we studied the smolt migration in the Rivière Saint-Jean on Quebec’s North Shore of the Gulf of St. Lawrence. Characteristics of this system that make it a good model for other systems are that it is relatively pristine in that it has not suffered site specific anthropogenic disturbances, and as it is in the northern Gulf of St. Lawrence it has likely been less impacted by climate change than rivers to the south. The objective of this study was to assess how environmental variables were related to the movement characteristics of migrating smolts. Specifically, we investigated: (1) how water temperature related to the onset of smolt migration; (2) the effects of the diel and tidal cycles on the time of day at which smolts travelled, and on the minimum speed over ground of smolts in the river, estuary and ocean ecosystems.
Materials and methods
Study site
The research was performed in the spring and summer of 2009 and 2010 in the Rivière Saint-Jean, in Québec on the North Shore of the Gulf of St. Lawrence (Fig. 1a). In the Rivière Saint-Jean, the Head of Tide (HoT) is located at a Bridge, 2.5 km upstream of the river mouth. The tides in this area are mixed semi-diurnal with heights ranging from 0.1 m to 2.6 m.
Location of the study site (a) and locations of hydro acoustic receivers (black circles), the smolt wheel (triangle) and the release site (dark square) in 2009 (b) and 2010 (c) in the Rivière Saint-Jean and in the near shore environment. Detection ranges of 500 m radius are indicated with an open (in ocean) or dark grey (in freshwater) circle around the receivers
Trapping and tagging protocols
A 1.52 m diameter rotary screw fish trap (also termed a “smolt wheel”) was used to trap salmon smolts. The trap was deployed about 12 km upstream from the HoT at the same location in 2009 and 2010 (Fig. 1b and 1c) and was operated without interruption from 4 June to 14 July 2009 and from 25 May to 28 June 2010. The trap was checked every morning and all smolts present were collected, anesthetized (0.2 ml of clove oil in 1000 ml of river water), weighed (+/− 0.1 g accuracy) and had their fork lengths (FL) recorded (+/− 1 mm). Selected smolts measuring 13.1 cm or more in FL and weighing at least 20.0 g were implanted with acoustic transmitters. The transmitters were uniquely coded and programmed to emit signals at a frequency of 69.0 kHz at randomly determined intervals varying from 20 s to 60 s (Vemco/Amirix Inc., Halifax, NS, Canada, V9-6 L model, 20 × 9 mm, 2.9 g in air, 1.9 g in water; minimum battery life of 55 days in 2009 and 74 days in 2010 and a programmed turn-off date 100 days after minimum battery life; power output of 146 dB with the reference level of one micropascal at 1 m). Activation of each tag was confirmed with a VR60 mobile acoustic receiver before the surgery. Transmitters were inserted into the abdominal cavity through a small incision on the ventral side between the pectoral and pelvic fins. During surgery, gills were irrigated to provide salmon with oxygen. Incisions were generally sealed with two interrupted stitches, but three stitches were occasionally required. A non-absorbable black sterile monofilament nylon suture (Ethilon 662 G, size 4–0) with reverse cutting edge (19 mm and 3/8 circle needle) was used for each surgery. Surgeries lasted 2 to 3 min (excluding anesthetic and recovery time).
Following the tagging procedure, smolts were placed in a recovery bucket until they recovered from the anesthesia. They were then transported to a holding cage in the river where they stayed approximately 11 h in 2009 and 4 h in 2010. In 2009 smolts were transported by boat 3 km upriver of the smolt wheel for release at dusk in an unsuccessful attempt to develop a mark-recapture estimate for smolt run size. In 2010 smolts were released from the holding cage, 100 m below the smolt wheel, at approximately 11:30 local time. The mean of tag weight (in air; 2.9 g) to smolt body weight was ≤ 12 % and the maximum transmitter weight was ≤ 14.2 % of the fish body weight. Tag burden was slightly greater than was reported in Brown et al. (1999; 6 to 12 %) and Hedger et al. (2008; 7 to 13 %) despite efforts to tag only the biggest smolts. In salmon smolt studies, tag burdens often range between 7 % and 10 % (Wagner et al. 2011). Lacroix et al. (2004) recommended body to tag weight ratio of 8 % or less because they observed that tags of 8.5 % of salmon smolt body weight negatively affected salmon smolt critical swimming speed 1 to 3 days after surgery compared to a control group.
Receiver mounting and deployments
Hydro acoustic receivers (Vemco/Amirix Inc. Halifax, NS, Canada, VR2 and VR2W models) were moored in the river and in the ocean to record fish passages (Fig. 1b and c). In the river, receivers were deployed on flat substrates in relatively slow flow sections of narrow width (<200 m) to depths of 6 m to maximize signal propagation and receiver detection efficiency (Clements et al. 2005). A diver using SCUBA positioned receivers on a cement block, and the unit was nested within rocks leaving only the tip of the hydrophone protruding. Other river anchors were suspended at depths of about 1 m from a surface float to an anchor. In the near shore coastal area, receivers were attached to a line 4 to 7 m below the surface depending on depth, with 1 to 3 jet floats (depending on current strength) as a surface marker, and a 20 kg trawl anchor. Sinking line was used from the surface floats to a nylon swivel (1200 lbs breaking strength) located below the receiver, which in turn was attached to the anchor with floating line.
Receivers have an expected detection range of 513 m radius under calm conditions or 497 m for 1.5 to 3.1 m.s−1 wind speed (calculated by Vemco, www.vemco.com). This study took place in a dynamic system. Detection efficiency can be affected by physical environmental variables, such as current speed, turbulence, changes in density (i.e., thermoclines) and wind speed over water. These variables will have had differing effects on all receivers, as the study area was not uniform in regard to all physical variables especially current speed and wind speed. Further, each receiver’s efficiency may have varied over time as current speed was variable depending on rain water input throughout the study. In this study we necessarily assume that receiver efficiency is similar over the study area and life of the study, however, there were likely some differences in detection efficiency over the spatial and temporal scale of the study.
Temperature measurements
Daily surface water temperature was recorded (°C) to the nearest decimal at the smolt trapping site in 2009 and 2010 during smolt wheel operation. Additionally, temperature loggers (Vemco/Amirix Inc. Minilogs) recorded water temperatures every 30 min at Guard’s Camp (2009 and 2010), at the Bridge (2010) and in the ocean at receivers “E” and “I” (2010) on the third arc array (Fig. 1c) from 8 June to 28 July 2009 and from 31 May to 26 July 2010.
Statistical analyses
The speeds of smolts were estimated using the time elapsed (last detection on a first receiver to last detection on the next receiver) to cover the distance in kilometers following the river path between two receivers, with distances determined using ArcGIS / Arc Map 10. Speeds were converted to cm*s−1 and mean values were calculated for each year. Smolt speeds over ground are referred as smolt speeds hereafter.
Smolt speeds in the river (freshwater travelling speed) were calculated between the Lac Castor and Landslide sites (Fig. 1b and c), if smolts were not detected at the Lac Castor or landslide receivers, the closest receivers upstream were used for speed calculation (this happened three times in 2009 and none in 2010).
Smolt speeds across the estuary were calculated individually between departure from the HoT (the Bridge receiver or at the Landslide receiver if no detections occurred at the Bridge receiver, which happened five times in 2009 and three times in 2010) and the last detection in the ocean on any first arc receiver. Smolts that were not detected on the first arc but subsequently logged onto the second were excluded from the calculation of estuary travel speeds because of the risk of confounding estuary with ocean speeds.
Ocean speeds were calculated in 2010 between the receiver of first detection (on the first or second receiver array) and the receiver of its last detection on the third receiver array (Fig. 1c). The length of the course track of detections between the first and last location was determined to calculate the distance individuals covered in the ocean. Ocean speeds were not calculated for 2009 due to overlapping detection ranges among receivers that confounded calculations of speed. Welch’s t-test (two-tailed; Welch 1947) was used to compare speed among the river, estuary and the ocean, within and between years.
The time of day at which an individual smolt was travelling was determined as the first detection of a smolt at each receiver (hh : mm, Local time [Greenwich Mean Time—4 h]). Only data from the two receivers from each of the freshwater, estuarine water and ocean water zones for which the most individual smolts were detected were kept for analysis to avoid repeated measures bias in the analysis. The package “circular” in R programming (Lund and Agostinelli 2007; R Development Core Team 2010) was used to plot the travel times at the selected receivers onto a clock diagram and calculate mean time of travel. Rayleigh’s test for circular distributions (Zar 1996; Rao and SenGupta 2001) was used to test if smolt travel times were uniformly distributed and also provided an index of concentration (r) of smolt travel times ranging from 0 % to 100 %.
Among the smolts whose speeds were calculated in the river, the estuary and the ocean, a subset of speeds were used that included only the smolts that travelled entirely during the night and entirely during the day within each ecosystem. This means that ten smolts were excluded from river speed calculations and six smolts from estuary speed calculations in 2009. In 2010, one smolt was excluded river speed calculations, eight from the estuary and eight from the marine ecosystems. Times falling within 20:00 and 4:00 local time were considered nocturnal and times falling between 4:01 and 19:59 were diurnal. These nocturnal and diurnal periods were chosen to reflect the sunset and sunrise times during the study. Mean diurnal and nocturnal minimum travelling speeds were compared among the river, estuary and ocean for each year using Welch’s t-test (two-tailed; Welch 1947) and chi-square tests. There could be slow moving fish that would be more likely to be excluded from the night period as that period is half as short as the day period.
Detections between the Landslide and Bridge receivers were examined to document residency at the HoT in relation to the direction of the tide. Final detection times recorded by the Bridge receiver were plotted against the tide cycle and diel periods to determine if detections occurred primarily at night or during the day, and to identify potential diel and tidal effect on smolt movements.
The effect of the tide cycle on smolt movements was examined by giving an angular value (xi°) to the last time (di) of detection at the head of tide receiver (Bridge). To do this, the duration of each ebb tide (Ei) and flood tide (Fi) was standardised to equal 180°. Tide tables for Mingan, QC were used (Canadian Hydrographic Service, Fisheries and Oceans Canada; www.tides.gc.ca) to obtain high tide times (thi) and low tide times (tei) and to calculate durations between specific low and high tides. If smolts departed during ebb tide, time after high tide (ti) was calculated (ti = di−thi) and if smolts departed during flood tide, time after low tide (ti) was calculated as such: (ti = di−tei). Angular values (xi°) were then attributed for each ti to fit that value on a circular plot following equation (1) for ebb tide departures or equation (2) for flood tide departures as per the formulas below:
Rayleigh test was performed to detect possible non-uniformity of departure times with tide cycles.
The last detection recorded for each of the smolts leaving the HoT for the estuary was used in the analysis of the influence of diel and tide cycles upon movements. Departure times for each of the smolts recorded at the Bridge receiver or the Landslide receiver if no detections occurred at the Bridge were plotted against the tide cycle for each year, and tide height (m) (available at www.tides.gc.ca and using the mean lower low water level as the reference point of 0 m) was compared between smolts that entered the estuary at night or during the day using Welch’s t-test. Smolt estuary speeds were contrasted with the tide stage (ebbing or flooding) by calculating the estuary speeds for the smolts that entered the estuary on an ebb tide with those that entered on a flood tide using Welch’s t-test. Ebb tides included high slack water periods since smolts leaving the Bridge then would cross the estuary at the onset of ebbing tides; similarly, low slack water periods were included in flood tides.
Results
Transmitter and smolt weight relationship
Transmitter weight relative to smolt weight was similar in the 2 years (mean = 11.3 ± SD 1.59 % in 2009; n = 44; and mean = 11.5 ± SD 1.85 % in 2010; n = 49).
Relationship of water temperature and fluctuation to the onset of the smolt run
In 2009 and 2010, the smolt run started at water temperatures of 10 °C. The smolt run peaked in mid-June in both years despite starting approximately 2 weeks later in 2009 compared to 2010 (Fig. 2). In 2009 the run was concentrated in 14 days (14 to 27 June). In 2010 we recorded sustained high captures over a period twice as long (26 days, 3 to 28 June). Daily mean water temperatures in the river were 17.1 °C during 2009’s smolt run compared to 13.9 °C in 2010 (t = 3.33, df = 22, P < 0.005). In 2010, tagged smolts were detected at the Bridge receiver from 6 to 23 June. Mean daily water temperature there at this time was 13.4 °C. In the ocean in 2010, post-smolts were detected on our coastal arrays between 6 and 30 June, during which period mean water temperatures ranged from 4.8 °C (at receiver “I”) to 5.0 °C (at receiver “E”).
Percentages of undetected and detected smolts to the HoT and ocean
Of the 44 tagged smolts in 2009, 68.2 % were detected reaching the HoT and 56.8 % were detected in the ocean. Of the 49 tagged smolts in 2010, 85.7 % were detected reaching the HoT and 41 smolts (83.7 %) were detected in the ocean. Of the 44 tagged smolts in 2009 and the 49 tagged smolts in 2010, 15.9 % and 12.2 %, respectively, were not detected after release.
Smolt travelling rates in the river, estuary and ocean
Acoustically tagged smolts took significantly less time to reach the ocean in 2009 (mean = 1.9 ± SD 1.2 days; n = 25) compared to 2010 (mean = 3.0 ± SD 1.2 days; n = 41) despite the fact that to reach the ocean smolts released in 2009 had to swim an additional 3 km from their release site compared to smolts in 2010 (Welch’s t-test two-tailed: t = 3.5; df = 49; P < 0.001).
Smolts were detected by a river receiver sooner after release in 2009 (mean of 23 h ± SD 19 h) compared to 2010 (mean of 45 h ± SD 28 h), which indicated significantly faster mean travel rates from release to first detection of 17.2 (± SD 15.5) cm*s−1 in 2009 compared 5.9 (± SD 5.0) cm*s−1 in 2010 (Welch’s two-tailed t-test: t = 4.2, df = 43, P < 0.0005). Also, smolt speeds from release to first detection were significantly slower than the river speeds calculated for section located further downstream of the release site (> 12 km) (Welch’s two-tailed t-tests: t =−3.7, df = 34, P < 0.0005 for 2009; t =−7.7, df = 42, P < 0.0001 for 2010).
In 2009, smolts moved at similar rates down the river and through the estuary, however, in 2010 river speeds were significantly slower than both estuary and ocean speeds. Smolts were faster crossing the estuary in 2010 compared to 2009 (Tables 1 and 2).
Periodicity of smolt detections in the river, estuary and ocean
Similar timing of movements was observed for smolts in the 2 years of the study (Fig. 3), although in 2009 some of the tendencies were not statistically significant at α = 0.05 (Rayleigh’s test for uniformity). The means of first detections of smolts at all sites and years were near sunset, or at night (Fig. 3). However, at all sites, some individuals moved at other times of day but most travelled during the night (Fig. 3). In freshwater and the ocean few first detections occurred in the first quarter of the day (between sunrise and noon local time). By contrast, few fish moved across the estuary between sunrise and noon compared to the afternoons and nights especially in 2010 when only three smolts were detected at Landslide and two at the Bridge (Fig. 3).
Smolt first detection times (black circles) in a 2009 at Portage (n = 17, mean = 00:18, r = 0.47, p = 0.02), Lac Castor (n = 22, mean = 19:23, r = 0.30, p = 0.15), Landslide (n = 30, mean = 21:26, r = 0.27, p = 0.10), Bridge (n = 26, mean = 20:48, r = 0.24, p = 0.02), Arc 3 (n = 14, mean = 18:55, r = 0.46, p = 0.03) and Arc 9 (n = 12, mean = 20:45, r = 0.40, p = 0.1) receivers; and in b 2010 at Guard’s Camp (n = 31, mean = 20:06, r = 0.35, p = 0.02), Lac Castor (n = 42, mean = 20:42, r = 0.40, p = 0.02), Landslide (n = 41, mean = 20:34, r = 0.41, p = 0.001), Bridge (n = 38, mean = 21:10, r = 0.49, p = 0.001), Arc 2 (n = 22, mean = 22:40, r = 0.64, p = 0.0001) and Arc 7 (n = 15, mean = 23:00, r = 0.57, p = 0.001) receivers. Circular mean vectors are indicated by an arrow and periods of darkness are shown with a black bar
Effect of dial cycle on smolt speeds in the river, estuary and ocean
Smolts swam faster across the estuary at night than during the day both in 2009 and in 2010 (Tables 3 and 4). There was no difference between diurnal and nocturnal smolt speeds in the river in 2009 and in the ocean in 2010, but smolts were faster at night than during the day in the river in 2010. Also, diurnal riverine smolt speeds were greater in 2009 compared to 2010 but there was no difference in yearly diurnal smolt speeds in the estuary but nocturnal riverine and estuarine speeds did not differ between 2009 and 2010.
Tidal influence on smolts entering the estuary
A residency period was observed at the HoT in the 1.6 km of river channel between the Landslide and Bridge receivers. Smolts stayed within range of these two receivers in 2009 and 2010 on average for 8 h 43 and 5 h 54 min, respectively. Residency time were longer within the Bridge receiver range compared to landslide’s range in 2010 (Welch’s two-tailed t-test: t = 2.1, df = 60, P < 0.05) but not in 2009. Some smolts were frequently detected on both receivers indicating rapid movement up and downstream between the ranges of the Bridge and Landslide receivers with the majority coinciding with ebbing tides. Most smolts entered the estuary at the onset of ebbing tide periods, on average 38 and 66 min after high tide in 2009 and 2010, respectively (Fig. 4).
Last detection times at the head of tide (Bridge receiver) for a 2009 tagged smolts (n = 23) and for b 2010 tagged smolts (n = 39) with each time adjusted to the tide cycle. The mean vector is indicated by the arrow: 17.9° (38 min after high tide) for 2009 and 31.6° (66 min after high tide) for 2010
Smolts did not favour a specific diel cycle (day or night) to enter the estuary in 2009 (χ2 = 2.1, P < 0.2) or in 2010 (χ2 = 0.9, P < 0.4), with approximately equal numbers of smolts being last detected at the Bridge at night than during the day (Fig. 5). Also, within years, smolts that departed the HoT at night travelled across the estuary on greater mean tide heights (m) compared to smolts departing the HoT during the day in 2009 (Welch’s two-tailed t-test: t = 4.5, df = 12, P < 0.001) but not in 2010 (t = 0.89, df = 35, P = 0.4). Between years, mean tidal height (m) for smolts diurnally entering the estuary was smaller in 2009 compared to 2010 (Welch’s two-tailed t-test: t = 2.2, df = 29, P = 0.03), however, there was no inter-year difference in tidal height for nocturnal estuary entrances (t = 1.9, df = 12, P = 0.09).
Discussion
Timing of the smolt run
The start of the smolt run in Rivière Saint-Jean in 2009 and 2010 coincided with water temperatures of 10 °C or greater. Rapid increases in temperature resulted in a shorter smolt run. Water temperature of approximately 10 °C has been reported to be a trigger for smolt migration (e.g., Gibson and Côté 1982; Moore et al. 1990; Jutila et al. 2005; Orell et al. 2007). Above 10 °C, Zydlewski et al. (2005) suggested that the number of degree days that a smolt experiences accelerates the migration of fish, with rapidly rising temperatures resulting in populations showing a more synchronized and shorter duration of smolt migration. Optimal timing of sea entry is believed to bring important survival benefits to smolts matching migration timing to positive environmental conditions including the start of spring ocean productivity cycles (McCormick et al. 1998).
Time of travel and travelling speed of smolts
It took twice as long for smolts to be first detected at a receiver after their release in 2010 compared to 2009. Delays of 16 h to 2 days in smolt migration following handling and/or tagging have been observed in other studies because the procedure and/or the associated stress likely hinder osmoregulatory abilities (Iversen et al. 1998). Yearly variation in delay may also have been caused by different release sites and times. Hansen and Jonsson (1985) reported that smolts released in the evening descended faster than smolts released during the day. Therefore, the diurnal time of release implemented in 2010 may have contributed to the greater post release migration delay observed that year compared to 2009 when smolts were released at sunset.
In the Rivière Saint-Jean freshwater ecosystem smolts appear to travel mostly around sunset and avoid travelling between sunrise and noon. In sub-arctic rivers, for which the smolt run occurs under 24 h daylight, smolts have been observed travelling at all times of the day (Davidsen et al. 2005; Orell et al. 2007). Perhaps smolts in temperate areas travel at sunset or in the night to avoid visual predators, which may not be an option for smolt in the sub-arctic.
More smolts were detected during the morning in the estuary compared to the riverine and marine stages possibly due to tidal influences since smolts selected ebb tides to swim through the estuary. Smolts residency durations in the HoT zone were similar. Gudjonsson et al. (2005) hypothesized that smolts entering the estuarine environment slowed their migration because they needed to adapt physiologically to saline waters. Also, differing developmental ability to tolerate salt water may explain the noticeable variation in smolt residency periods at the HoT (McCormick et al. 1998; McCormick 2009).
Post-smolts mostly travelled at night once in the marine environment. In the ocean, feeding opportunities are greater but so may be the abundance of predators (Dieperink et al. 2002) and as a consequence it may be risky for post-smolts to be actively moving in daylight. The choice between diurnal or nocturnal movements at sea is probably linked to predator avoidance strategies (Fraser et al. 1993; Jepsen et al. 2006), but also trade-offs between predator avoidance and feeding efficiency (Metcalfe et al. 1999; Ibbotson et al. 2006; Jepsen et al. 2006).
Tag burden may have affected initial smolt swimming rates; however, it was not possible to measure any effect. Tags used were the smallest available that would provide sufficient tag detection range and battery life to fulfill the objectives of the study that included oceanic migration and possible detection at acoustic receiver lines off Anticosti Island and the Straight of Belle Isle.
Smolt velocities recorded in the Rivière Saint-Jean were relatively fast compared to other sites in the Gulf of Saint Lawrence. In the Gaspé Bay, Hedger et al. (2008) measured slower smolt velocities (27.5 cm*s−1). The discrepancy between these two sites may be due to combined effects of estuary length (2.5 km in our study versus 10 km in Gaspé Bay), the current velocity and water circulation patterns.
Smolts travelled faster at night regardless of tidal height. Smolts may travel across the estuary at night and minimize their movements during the day to avoid visual predators (birds and other fish species). Or, because smolts are visual predators, they are more efficient when feeding during the day (Fraser et al. 1993; Metcalfe et al. 1999). Smolts may spend more time feeding in the estuary during the day taking advantage of greater amounts of prey (Correll 1978) available in that ecosystem compared to the riverine environment, which would results in slower diurnal speeds across the estuary.
Ocean speeds (2010 only) over the 4 km after sea entry were high compared to average speed of 47 cm*s−1 recorded for juveniles in the first 4 km into the Alta Fjord of Northern Norway (Davidsen et al. 2009) or average speed of 34.7 cm*s−1 observed in another Fjord of middle Norway (Thorstad et al. 2004). Tidal currents strength in the coastal environment of the Rivière Saint-Jean with residual currents varying south to south west (El-Sabh 1976; Han 2004) may have contributed to the relative fast speeds but estuarine and marine juvenile speeds did not differ. This may have been a consequence of smolts crossing the estuary at the onset of the ebbing tide, under which condition the tide may regulate travelling speeds across the estuarine and marine stages.
Conclusions
Compared to many more southern rivers, returns of adult Atlantic salmon to rivers in this region are considered relatively healthy, albeit present rates are at lower levels than have occurred in the recent past (COSEWIC 2010). This study may provide a baseline of conditions for migration and migration behaviour that can be compared with future research as the area becomes more affected by climate change. As temperatures warm as is predicted in climate change research (Thibodeau et al. 2010) this may affect juvenile growth (Swansburg et al. 2002). Also the timing of smolt emigration (McCormick et al. 1999) may shift. The timing and patterns of movements of smolts and post-smolts observed were similar to patterns observed in other rivers including where populations are far less healthy such as in the inner Bay of Fundy (WWF 2001). This suggests that the mortality factors and their drivers that are responsible for low return rates of Atlantic salmon are occurring during the extended ocean migration phase, and not during the early portion of the smolt and post-smolt migration. Future research needs to focus on these later portions of the migration if we are to understand what the mortality causes are, and their significance for the conservation of the Atlantic salmon.
References
Antonsson T, Gudjonsson S (2002) Variability in timing and characteristics of Atlantic salmon smolt in Icelandic rivers. Trans Am Fish Soc 131:643–655
Brown RS, Cooke SJ, Anderson WG, Mckinley RS (1999) Evidence to challenge the “2 % rule” for biotelemetry. N Am J Fish Manag 19:867–871
Cairns DK (ed) (2001) An evaluation of possible causes of the decline in pre-fishery abundance of North American Atlantic salmon. Canadian Technical Report of Fisheries and Aquatic Sciences No. 2358. http://www.seagrant.umaine.edu/files/pdf-global/08Cairns2001.pdf. Accessed 23 September 2009
Caron F (1983) Migration vers l’Atlantique des post-saumoneaux (Salmo salar) du Golfe du Saint-Laurent. Nat Can 110:223–227
Clements S, Jepsen D, Karnowski M (2005) Optimization of an acoustic telemetry array for detecting transmitter-implanted fish. N Am J Fish Manag 25:429–436
Correll DL (1978) Estuarine productivity. BioSci 28:646–650
COSEWIC (2010) COSEWIC assessment and status report on the Atlantic Salmon Salmo salar (Nunavik population, Labrador population, Northeast Newfoundland population, South Newfoundland population, Southwest Newfoundland population, Northwest Newfoundland population, Quebec Eastern North Shore population, Quebec Western North Shore population, Anticosti Island population, Inner St. Lawrence population, Lake Ontario population, Gaspé-Southern Gulf of St. Lawrence population, Eastern Cape Breton population, Nova Scotia Southern Upland population, Inner Bay of Fundy population, Outer Bay of Fundy population) in Canada. Committee on the Status of Endangered Wildlife in Canada. Ottawa, pp. xivii + 136
Davidsen J, Svenning M-A, Orell P, Yoccoz N, Dempson JB, Niemela E, Klemetsen A, Lamberg A, Erkinaro J (2005) Spatial and temporal migration of wild Atlantic salmon smolts determined from a video camera array in the sub-arctic River Tana. Fish Res 74:210–222
Davidsen JG, Rikardsen AH, Halttunen E, Thorstad EB, Økland F, Letcher BH, Skarðhamar J, Næsje TF (2009) Migratory behaviour and survival rates of wild northern Atlantic salmon Salmo salar post-smolts: effects of environmental factors. J Fish Biol 75:1700–1718
R Development Core Team (2010) R: A language and environment for statistical computing. R Foundation for Statistical Computing Vienna, Austria. ISBN 3-900051-07-0, URL http://www.R-project.org.
Dieperink C, Bak BD, Pedersen LF, Pedersen MI, Pedersen S (2002) Predation on Atlantic salmon and sea trout during their first days as postsmolts. J Fish Biol 61:848–852
Dutil J-D, Coutu JM (1988) Early marine life of Atlantic Salmon, Salmo Salar, postsmolts in the Northern Gulf of St. Lawrence. Fish Bull 86:197–212
El-Sabh MI (1976) Surface circulation pattern in the Gulf of St. Lawrence. J Fish Res Board Can 33:124–138
Fraser NHC, Metcalfe NB, Thorpe JE (1993) Temperature-dependent switch between diurnal and nocturnal foraging in salmon. Proc R Soc Lond B 252:135–139
Fried SM, McCleave JD, LaBar GW (1978) Seaward migration of hatchery reared Atlantic salmon, Salmo salar, smolts in the Penobscot River estuary, Maine: riverine movements. J Fish Res Board Can 35:76–87
Friedland KD, Reddin DG, McMenemy JR, Drinkwater KF (2003) Multidecadal trends in North American Atlantic salmon (Salmo salar) stocks and climate trends relevant to juvenile survival. Can J Fish Aquat Sci 60:563–583
Gibson RJ, Côté Y (1982) Production de saumoneaux et recaptures de saumons adultes étiquettés à la rivière Matamec, côte-nord, Golfe du Saint-Laurent, Québec. Nat Can (Rev Ecol Syst) 109:13–25
Greene CH, Pershing AJ (2007) Climate drives sea change. Science 315:1084–1085
Gudjonsson S, Jonsson IR, Antonsson T (2005) Migration of Atlantic salmon, Salmo salar, smolt through the estuary area of River Ellidaar in Iceland. Environ Biol Fish 74:291–296
Han G (2004) Sea level and surface current variability in the Gulf of St Lawrence from satellite altimetry. Int J Remote Sens 25:5069–5088
Hansen LP, Jonsson B (1985) Downstream migration of hatchery-reared smolts of Atlantic salmon (Salmo salar) in the river Imsa, Norway. Aquaculture 45:237–248
Haro A (2009) Population and habitat Restoration—Preamble. In: Haro AJ, Smith KL, Rulifson RA, Moffitt CM, Klauda RJ, Dadswell MJ, Cunjak RA, Cooper JE, Beal KL, Avery TS (eds) Challenges for diadromous fishes in a dynamic global environment. Am Fish Soc Sym 69, Bethesda, Maryland, pp 495–496
Hedger RD, Martin F, Dodson JJ, Hatin D, Caron F, Whoriskey FG (2008) The optimized interpolation of fish positions and speeds in an array of fixed acoustic receivers. ICES J Mar Sci 65:1248–1259
Ibbotson AT, Beaumont WRC, Pinder A, Welton S, Ladle M (2006) Diel migration patterns of Atlantic salmon smolts with particular reference to the absence of crepuscular migration. Ecol Freshw Fish 15:544–551
Iversen M, Finstad B, Nilssen KJ (1998) Recovery from loading and transport stress in Atlantic salmon (Salmo salar L.) smolts. Aquaculture 168:387–394
Jepsen N, Holthe E, Økland F (2006) Observations of predation on salmon and trout smolts in a river mouth. Fish Manag Ecol 13:341–343
Jutila E, Jokikokko E, Julkunen M (2005) The smolt run and postsmolt survival of Atlantic salmon, Salmo salar L., in relation to early summer water temperatures in the northern Baltic Sea. Ecol Freshw Fish 14:69–78
Lacroix GL, Knox D (2005) Distribution of Atlantic salmon (Salmo salar) postsmolts of different origins in the Bay of Fundy and Gulf of Maine and evaluation of factors affecting migration, growth, and survival. Can J Fish Aquat Sci 62:1363–1376
Lacroix GL, McCurdy P (1996) Migratory behaviour of post-smolt Atlantic salmon during initial stages of seaward migration. J Fish Biol 49:1086–1101
Lacroix GL, Knox D, McCurdy P (2004) Effects of implanted dummy acoustic transmitters on juvenile Atlantic salmon. Trans Am Fish Soc 133:211–220
Lacroix GL, Knox D, Stokesbury MJW (2005) Survival and behaviour of post-smolt Atlantic salmon in coastal habitat with extreme tides. J Fish Biol 66:485–498
Lund U, Agostinelli C (2007) Circular: Circular Statistics. R package version 0.3–8
Martin F, Hedger RD, Dodson JJ, Fernandes L, Hatin D, Caron F, Whoriskey FG (2009) Behavioural transition during the estuarine migration of wild Atlantic salmon (Salmo salar L.) smolt. Ecol Freshw Fish 18:406–417
McCleave JD (1978) Rhythmic aspects of estuarine migration of hatchery-reared Atlantic salmon (Salmo salar) smolts. J Fish Biol 12:559–570
McCormick SD (2009) Evolution of the hormonal control of animal performance: insights from the seaward migration of salmon. Integr Comp Biol 49:408–422
McCormick SD, Hansen LP, Quinn TP, Saunders RL (1998) Movement, migration, and smolting of Atlantic salmon (Salmo salar). Can J Fish Aquat Sci 55(Suppl 1):77–92
McCormick SD, Cunjak RA, Dempson B, O’Dea MF, Carey JB (1999) Temperature-related loss of smolt characteristics in Atlantic salmon (Salmo salar) in the wild. Can J Fish Aquat Sci 56:1649–1667
Metcalfe N, Fraser N, Burns M (1999) Food availability and the nocturnal vs. diurnal foraging trade-off in juvenile salmon. J Anim Ecol 68:371–381
Moore A, Russell IC, Potter ECE (1990) Preliminary results from the use of a new technique for tracking the estuarine movements of Atlantic salmon, Salmo salar L., smolts. Aquac Fish Manag 21:369–371
Moore A, Potter ECE, Milner NJ, Bamber S (1995) The migratory behaviour of wild Atlantic salmon (Salmo salar) smolts in the estuary of the River Conwy, North Wales. Can J Fish Aquat Sci 52:1923–1935
Moore A, Ives S, Mead TA, Talks L (1998) The migratory behaviour of wild Atlantic salmon (Salmo salar L.) smolts in the River Test and Southampton Water, southern England. Hydrobiology 372:295–304
Orell P, Erkinaro J, Svenning MA, Davidsen JG, Niemelä E (2007) Synchrony in the downstream migration of smolts and upstream migration of adult Atlantic salmon in the subarctic River Utsjoki. J Fish Biol 71:1735–1750
Rao JS, SenGupta A (2001) Topics in circular statistics, sections 3.3.2 and 3.4.1. World Scientific, Singapore
Solomon DJ (1978) Some observations on salmon smolt migration in a chalkstream. J Fish Biol 12:571–574
Swansburg EO, Chaput G, Moore D, Caissie D, El-Jabi N (2002) Size variability of juvenile Atlantic salmon: links to environmental conditions. J of Fish Biol 61(3):661–683
Thibodeau B, de Vernal A, Hillaire-Marcel C, Mucci A (2010) Twentieth century warming in deep waters of the Gulf of St. Lawrence: a unique feature of the last millennium. Geophys Res Lett 37:L17604. doi:10.1029/2010GL044771
Thorpe JE, Metcalfe NB, Fraser NHC (1994) Temperature dependence of the switch between nocturnal and diurnal smolt migration in Atlantic salmon. In: MacKinlay DD (ed) High performance fish. Fish Physiology Association, Vancouver, pp 83–86
Thorstad EB, Økland F, Finstad B, Sivertsgård R, Bjørn PA, McKinley RS (2004) Migration speeds and orientation of Atlantic salmon and sea trout post-smolts in a Norwegian fjord system. Environ Biol Fish 71:305–311
Todd CD, Hughes SL, Marshall CT, MacLean JC, Lonergan ME, Biuw EMN (2008) Detrimental effects of recent ocean surface warming on growth condition of Atlantic salmon. Glob Chang Biol 14:958–970
Veselov AJ, Sysoyeva MI, Potutkin AG (1998) The pattern of Atlantic salmon smolt migration in the Varzuga River (White Sea Basin). Nord J Freshw Res 74:65–78
Wagner GN, Cooke SJ, Brown RS, Deter KA (2011) Surgical implantation techniques for electronic tags in fish. Rev Fish Biol Fisher 21:71–78
Welch BL (1947) The generalization of “Student’s” problem when several different population variances are involved. Biometrika 34:28–35
Whoriskey F, Chaput G, Cameron P, Moore D, Hambrook M (2008) Sonic tracking of North American Atlantic salmon smolts to sea: correlates of stage specific survivals and lessons on the migration pathway. ICES WGNAS Working Paper 36.
WWF (World Wildlife Fund) (2001) The status of wild Atlantic salmon: a river by river assessment. http://awsassets.panda.org/downloads/salmon2.pdf Accessed 27 January 2011
Zar JH (1996) Biostatistical analysis, 3rd edn. Prentice-Hall, Englewood Cliffs
Zydlewski GB, Haro A, McCormick SD (2005) Evidence for cumulative temperature as an initiating and terminating factor in downstream migratory behaviour of Atlantic salmon (Salmo salar) smolts. Can J Fish Aquat Sci 62:68–78
Acknowledgments
This research was funded by the Atlantic Salmon Federation and the Club Hill Camp Inc. We thank gratefully Mari and Doug Harpur and James J. Hill III for their support and for providing us with the facilities. This research would not have been possible without the Hill Camp’s fishing guides who helped with field work and especially Gilles Maloney for his assistance with smolt processing. We also want to acknowledge Louis Vaillancourt who volunteered generously his time for equipment deployment and retrieval at sea. MJWS was supported by the Canada Research Chairs program.
Author information
Authors and Affiliations
Corresponding author
Rights and permissions
About this article
Cite this article
Lefèvre, M.A., Stokesbury, M.J.W., Whoriskey, F.G. et al. Migration of Atlantic salmon smolts and post-smolts in the Rivière Saint-Jean, QC north shore from riverine to marine ecosystems. Environ Biol Fish 96, 1017–1028 (2013). https://doi.org/10.1007/s10641-012-0100-8
Received:
Accepted:
Published:
Issue Date:
DOI: https://doi.org/10.1007/s10641-012-0100-8