Abstract
While most of the available studies on climate change effects on Lepidosauria focused on changes in species distribution, none has focused on evaluating biogeographic and phylogenetic patterns of these effects. Here, we aimed to test if some lepidosaurian clades are more likely to be vulnerable than others and if their vulnerability corresponds with zoogeographic-related climatic conditions. We measured Pagel’s λ and Blomberg’s K and indicated a significant phylogenetic signal of lepidosaurians’ vulnerability to climate change, which tends to increase towards more recent clades. We performed a parsimony analysis of endemicity to determine the most climatically vulnerable zoogeographical realms, considering local lepidosaurian vulnerability. We recovered that taxa occurring in multiple zoogeographical realms are usually vulnerable across different geographic regions. Thus, we indicated that the lepidosaurian vulnerability is not related to their occurrence area, since most of the clades are shown to be vulnerable despite their biogeographic distribution or local climate conditions. We conducted a meta-analysis and showed that climate change is globally affecting taxa distribution, with no effects of heterogeneity. Finally, we performed a panbiogeographical analysis and found that Neotropical, Afrotropical, Australian, and Nearctic realms contain the highest number of biotic convergence zones. Areas with high spatial concentration of diversity also presented a greater number of vulnerable species, indicating that these areas can be possible targets for conservation at a larger scale and may help to identify especially diverse areas for conservation efforts at a small scale, focusing on buffering the effects of climate change on local populations.
Similar content being viewed by others
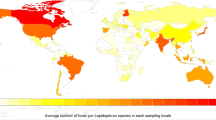
Avoid common mistakes on your manuscript.
1 Introduction
Climate change poses severe threats to the conservation of natural landscapes across the globe and is cited as one of the main drivers of the current global biodiversity crisis (Garcia et al. 2014; Ceballos et al. 2015). The Earth’s average surface temperature has increased 1.1 °C from 1901 to 2018, and considering that current emissions will not be reduced until the end of the century (“business-as-usual” scenario of greenhouse gases emissions), future estimates indicate a potential increase of about 4.8 °C by 2100 (Field et al. 2014; IPCC 2018).
The vulnerability of an organism to environmental change depends on its exposure to change, its sensitivity to this change, and its ability to recover or adapt to it (Williams et al. 2012). Predicting the potential risks to biodiversity caused by climate change has become an extremely active field of research (Bellard et al. 2012), and some studies suggest that the trends of current global climate change could become the most significant global threat to biodiversity over the next few decades (Pereira et al. 2010; Cahill et al. 2012).
Lepidosauria, which includes lizards, snakes, amphisbaenians, and tuatara, is the most diverse group of extant reptiles in terms of number of species and morphological, physiological, and ecological traits (Jetz and Fine 2012). Because Lepidosauria are ectothermic, several of their life history traits are likely to be influenced by environmental temperature variation (Diele-Viegas and Rocha 2018), making them excellent models to evaluate potentzial effects of climate change on terrestrial ectotherms (Chamaillé-Jammes et al. 2006).
Most of the species-level diversity of Lepidosauria is located in the tropics and subtropics (Roll et al. 2017), where some species are already experiencing body temperatures above their physiological optima, indicating a potentially higher vulnerability than species from cold environments (Huey 1982; Huey et al. 2009; Diele-Viegas et al. 2018). However, temperate species are also likely to be vulnerable, assuming that their physiological adaptations for living in cold environments may hinder their ability to cope with hotter environments (Monasterio et al. 2013; Kubisch et al. 2016).
While most of the available studies evaluating climate change effects on Lepidosauria focused on changes in species distribution (Diele-Viegas and Rocha 2018), none has focused on evaluating general biogeographic and phylogenetic effects on Lepidosauria with a macroecological approach. Thus, there is still a lack of information regarding which group or area around the globe is likely to be more severely affected by climate change.
Here, we aimed to evaluate if the effects of climate change on Lepidosauria around the world follow a general phylogenetic or biogeographic pattern. The first hypothesis posits phylogenetic conservatism, and in this scenario, we would expect to see that some groups are more likely to be vulnerable to climate change than others, independent from their biogeography. The second hypothesis posits that their vulnerability corresponds with biogeography-related climatic conditions irrespective of relatedness between species. Thus, we aim to test these hypotheses and to seek to determine (1) the most climatically vulnerable areas around the world with regard to their local Lepidosauria fauna; (2) the most climatically vulnerable lepidosaurian taxa; (3) the general trends of the influences of climate change on lepidosaurian distribution at the species level; and (4) the critical areas for conservation of the lepidosaurian species evaluated by the studies addressed here.
2 Methods
2.1 Searching for global trends
We focused our review on studies that aimed to evaluate the influences of recent changes in climate on Lepidosauria. We conducted a literature search using online publication databases (Periódicos CAPES, PubMed, Google Scholar, SciELO, Scopus, Web of Science, and Wiley Online Library), using the following keywords: [Climate Change OR Global Warming OR Climate Warming] AND [Lizard* OR Snake* OR Sphenodon OR Tuatara OR Lepidosauria OR Squamata]. We also included studies identified by contacting authors and studies listed in the references sections of the previously identified articles that were not captured by the search in the literature databases. We excluded studies that focused on paleoclimatic changes since we are focusing on the effects of current and future changes in climate change.
We extracted the following information from the analyzed articles: the impacts of climate change on the evaluated species (negative, neutral, positive); collecting area to allow identification of zoogeographical realms [i.e., geographical entities delineated by considering an animal’s global distribution and phylogenetic relationships, (Holt et al. 2013; Table S1)]; the coordinates given by the studies (Table S2; Fig. S1); and the area under the curve (AUC) values of the receiver operating characteristic (ROC) analysis published when the research focused on species distributions (Table S3).
To evaluate the phylogenetic patterns of climate change effects on Lepidosauria, we aggregated species into more comprehensive groups using a phylogeny supported by both nuclear and mitochondrial data (Pyron et al. 2013): order (e.g., Sphenodontia), suborder (e.g., Serpentes), infraorder (e.g., Gekkota, Iguania, and Anguimorpha), and superfamily (e.g., Lacertoidea and Scincoidea). To evaluate the biogeographic patterns among the evaluated taxa, we characterized their distribution using the most recent classification of zoogeographic realms (Holt et al. 2013): Afrotropical, Australian, Madagascan, Nearctic, Neotropical, Oceanian, Oriental, Palearctic, Panamanian, Saharo-Arabian, and Sino-Japanese.
We considered a species vulnerable when 50% or more studies indicated negative effects of climate change on the species (e.g., range contractions, inability to genetically, behaviorally or physiologically cope with the changes in climate). Conversely, non-vulnerable species were those for which more than 50% of the studies indicated neutral or positive effects of climate change. For more comprehensive groups, they were considered to be “vulnerable” when more than 50% of the evaluated species for that group were considered vulnerable and “non-vulnerable” when less than 50% of the evaluated species were considered vulnerable. When 50% of the species were considered to be vulnerable, we considered this group as “vulnerable or not.”
To test our phylogenetic hypothesis that vulnerability is conserved within the phylogeny, we measured the phylogenetic signal of species’ responses to climate change both at the species level and across different phylogenetic scales (Graham et al. 2018). We used the D metric to measure the phylogenetic signal at the species level, which was designed to measure phylogenetic signal on binary traits (Fritz and Purvis 2010). It can vary between 0, when the trait is distributed as expected under a Brownian motion model of evolution, and 1, when the trait is randomly distributed along the phylogeny (Fritz and Purvis 2010).
The phylogenetic scaling analysis was used to indicate if and how the phylogenetic signal changes across different phylogenetic resolutions. The phylogenetic scaling approach assumes that phylogenies represent hierarchically nested groups that end up in tips that normally represent the species. By grouping the species into higher hierarchical lineages or in other words lowering the phylogenetic resolution of the tree, we can perform this phylogenetic analysis on a different scale (see Graham et al. 2018, for a detailed discussion on the subject). Thus, we can employ this approach to check if vulnerability to climate change is conserved at other phylogenetic scales rather than the species level and therefore shed some light on the origins of such vulnerability. To lower the phylogenetic resolution, we grouped species according to their phylogenetic similarity, which was measured as the pairwise branch length distance between tips. We set the number of groups to vary based on a continuous set of decreasing phylogenetic resolution, from 350 (high phylogenetic resolution) to 20 (low phylogenetic resolution) groups of the most phylogenetically similar species. The lower bound was chosen based on a reasonable number of observations for which the phylogenetic signal could still be measured. We defined the maximum number of groups based on a number slightly higher than the number of genera in this study (n = 340). This upper bound establishes a safety margin as current groups could be split into more groups in the future, although we highlight that such a change did not change the general pattern of our findings in this analysis. We ended up with 67 trees across a gradient of phylogenetic resolution, where the tips in these trees represented the defined groups of species (or higher hierarchical lineages). We then calculated the percentage of vulnerable species in each group and measured the phylogenetic signal of the percentage of vulnerable species per group. Since these new vulnerability measures at the tip values were continuous, we used both Pagel’s λ (Pagel 1999) and Blomberg’s K (Blomberg et al. 2003) to measure phylogenetic signal. Pagel’s λ varies from 0, no phylogenetic signal, to 1, when the trait is distributed as expected under Brownian motion. Values close to 0 in Bloomberg’s K also indicate a randomly distributed trait along the phylogeny and 1 a trait distributed as expected under Brownian motion. However, K values can sometimes go above 1 when a trait is more conserved than expected under Brownian motion. All phylogenetic analyses were carried out using the statistical software environment R (R Core Team 2018).
2.2 Parsimony analysis of endemicity (PAE)
The PAE is a biogeographical tool designed to classify areas according to the shared presence of taxa (Nihei 2006). We adapted this methodology to test our biogeographic hypothesis and determine the most climatically vulnerable zoogeographical realms in terms of local lepidosaurian vulnerability. If our hypothesis is correct, PAE results will indicate a pattern in taxa vulnerability determined by the climatic conditions of the realms. For example, tropical realms (e.g., Neotropical, Afrotropical, and Madagascan) should present similar rates of taxa vulnerability, as should the subtropical and temperate realms (e.g., Nearctic and Palaearctic), and the zoogeographical realms with different climatic conditions should have different patterns from each other.
Since most of the evaluated families were subsampled, our assumptions were based on the proportions among the analyzed species per family. The PAE analysis relies on the presence of shared species between the predetermined areas (Morrone 2014), so we first associated the presence/absence of evaluated families among the zoogeographical realms through a binary matrix of presence (1) and absence (0) generated with Mesquite (Maddison and Maddison 2018). Then, we generated a PAE tree by using the implicit enumeration algorithm in the phylogenetic software TNT (Goloboff et al. 2008).
To associate the taxa vulnerability to parsimony of endemicity, we evaluated the vulnerability of the families at the zoogeographical realms through a binary matrix. We inputted the following codes into the matrix: (1) for “vulnerable” families, (0) for “non-vulnerable” families, and (01) for “vulnerable or not” families. Then, we reconstructed these values over the PAE cladogram and merged the families into more inclusive clades with the software Mesquite (Maddison and Maddison 2018). The raw data resulting from this analysis (nexus, matrices, and area cladogram) are available in Supplementary Data 1.
2.3 Meta-analysis
The extent to which a species’ distribution is correlated with climate variables may indicate its sensitivity to future climate change (Sinervo et al. 2010). We conducted a meta-analysis to evaluate if the current literature supports the hypothesis that lepidosaurians will change their geographical distributions in response to climate change. To do so, we used the package metafor (Viechtbauer 2010) in R (R Core Team 2018).
In species distribution modeling (SDM), a standard metric to describe how well the model variables predict species’ distributions is the AUC of the ROC (Jiménez-Valverde 2012). Thus, we obtained these values from 35 articles in which occurrence data of 577 species were considered to fit climate variables using SDM. Since we aimed to evaluate the correlation between species distribution and climate change, we converted the AUC values (a measurement of model’s performance) into Pearson’s r (a measurement of correlation between variables), based on (Salgado, 2018; Table S3). We measured how variable this value was among taxa and zoogeographical realms, separated into vulnerable or not based on observed climate responses (see above methods; e.g., vulnerable Iguania from Neotropical; non-vulnerable Scincoidea from Australian).
Since most of the studies do not provide the sample size used in the AUC analysis, we calculated the average r for these groups and determined the sample size by the number of evaluated species in each group (Diele-Viegas and Rocha 2018). We treated the average r as the fixed effect size (vulnerable/non-vulnerable for each taxon/zoogeographical realm). In order to determine the strength of the effects of climate change on species distribution, we tested for variation in Pearson’s r by describing and testing for significant heterogeneity of the effect size (Q) and further testing for inconsistency among groups by measuring the variation of the effect and the proportion of the true variation between the groups (I2), considering the equation below (Higgins and Thompson 2002). Low values in each metric indicated little variability among groups and consequently low heterogeneity and a strong effect of climate change on species distribution.
2.4 Panbiogeography
We performed a panbiogeographical analysis to delimit the potential areas for conservation of the evaluated lepidosaurians and identify poorly sampled areas by using the software Croizat 2.1.0 (Cavalcanti 2009). This analysis uses occurrence data to draw geographic distribution of clades as tracks. When tracks of different clades overlap, it indicates a region of shared distribution, called generalized track. Then, when these generalized tracks overlap or cross, it indicates nodes, which are regions where numerous clades are predicted to coexist or have coexisted at some point in time and therefore might show a considerably high diversity (see Croizat et al. 1974). Thus, generalized tracks and nodes represent potential areas of distribution of groups of species, in an historical sense (Heads 2004). Therefore, these features can be placed in localities without reported species occurrences.
We considered the distribution of the comprehensive groups rather than individual genera/species and used the results to determine where the generalized tracks (i.e., areas where different clades co-occur) overlap or cross forming the nodes (i.e., potential areas for conservation). We calculated the generalized tracks considering the coordinates of the evaluated species given by the studies. We considered a congruence value of 1.0, which indicates a complete congruence between the species distribution. Then, we defined the biotic convergence zones, or potential areas for species conservation, as the localities where two or more generalized tracks overlapped (Morrone 2004; Echeverría-Londoño and Miranda-Esquivel 2011).
3 Results
We analyzed a total of 200 articles published from 2000 to January 2018 (Table S4). These studies evaluated 1875 species, which represents 18% of lepidosaurian diversity (Uetz et al. 2018). From those, 1114 species were considered vulnerable to changes in climate (59.41%), 688 were considered non-vulnerable (36.69%), and 73 were not evaluated for vulnerability (3.89%). Zootoca vivipara (Lacertilia: Lacertoidea) was the most studied species, with 14 studies indicating negative effects of climate change, eight studies indicating positive effects, and two studies with no definite conclusions about this issue. All studies considered changes on temperature and/or precipitation over time as climate change factors. Other climate change factors, such as sea level rise, were not addressed by this literature.
Most of the lepidosaurian families were subsampled, with Typhlopidae (Serpentes) presenting the smallest ratio (N = 4; 0.98%) of evaluated species related to the current known diversity for the family (Uetz et al. 2018; Table 1). Besides Bipedidae (N = 3), Helodermatidae (N = 2), Lanthanotidae (N = 1), Shinisauridae (N = 1), and Sphenodontidae (N = 1), which had 100% of species evaluated, Phrynosomatidae (N = 101; 63.5%) and Liolaemidae (N = 190; 61.9%) were the most well represented families among the studies (Table 1).
At the species level, vulnerability had weak phylogenetic signal among the evaluated species (D = 0.75). However, our analysis revealed that the strength of the phylogenetic signal for both Pagel’s λ and Bloomberg’s K increased when considering higher-level phylogenetic relationships (e.g., Gekkota, Scincoidea; Fig. 1). There is a clear trend of increasing vulnerability among most recent clades (Fig. 2), when excluding Sphenodontia. Besides being the most basal clade, Sphenodontia is represented by only one (vulnerable) species, and thus, it has the highest vulnerability ratio among the analyzed groups (1:1).
3.1 Global trends of lepidosaurian vulnerability
Most zoogeographical realms were not sampled homogenously (Fig. S1), except the Neotropical, Panamanian, and Australian realms. Nearctic and Palaearctic realms lack studies focused on northern species, where there are up to 200 known species (Uetz et al. 2018), mainly in Canada, Russia, Kazakhstan, and Mongolia. The Afrotropical realm lacks studies focusing on Central Africa species, which reaches up to 485 known species (Uetz et al. 2018), mainly in Angola, Congo, and Zambia. Studies in the Saharo-Arabian realm encompass only 144 from more than 650 known species within the realm, while those on the Oriental realm lack species from Pakistan and the Indi-Pacific islands, which host more than 1370 species (Uetz et al. 2018). Finally, the Sino-Japanese realm lacks studies on species from Central Asia and Japan, which host more than 520 species (Uetz et al. 2018).
Although most of the evaluated species were from Australian, Neotropical, and Nearctic realms, the ratio of vulnerable species was comparatively higher for the Sino-Japanese, Afrotropical, and Nearctic realms (Table 2). All zoogeographical realms had at least 50% of the evaluated species considered to be vulnerable to climate change, except for Oceanian and Madagascan realms (Fig. 3). Most of the taxa occur in multiple zoogeographical realms and are usually vulnerable across different geographic regions (Fig. 3). Thus, our parsimony analysis of endemicity indicated that the lepidosaurian vulnerability is not related to the occurrence area of the comprehensive groups, since most of the clades are shown to be vulnerable despite their biogeographic distribution (Fig. 3). Oceanian was the only realm with no taxa evaluated as vulnerable to climate change, while in the Madagascan realm, only Iguania was considered vulnerable (Fig. 3).
Taxa vulnerability associated with parsimony analysis of endemicity. Stars represent the vulnerable taxa along the zoogeographical realms, pentagons are the non-vulnerable taxa along the realms, and squares are taxa that did not occur in this realm. We considered a taxon vulnerable if more than 50% of the evaluated species for that taxon was considered vulnerable by the evaluated studies
Our meta-analysis demonstrated the strength of the climate change effects on species distribution of the evaluated taxa along the zoogeographical realms (I2 = 0, p < 0.001), with no effect size heterogeneity (Q(df) = 5.645, p = 0.99). Both vulnerable and non-vulnerable Australian scincoideans had substantial effects on the analysis, followed by vulnerable Australian Serpentes and Palearctic lacertoideans (Fig. 4). Three non-vulnerable and seven vulnerable taxa presented confidence intervals reaching the neutral effect on the analysis (Fig. 4), indicating that these groups showed no effect of climate change on their species distribution. However, analyses of most of the groups resulted in robust and precise statistical findings that corroborate that species distribution is changing due to climate change, for both vulnerable and non-vulnerable species.
Association between taxa distribution and climate change by zoogeographical realms. RE model (black diamond) = Random effects. The x-axis represents the absolute statistics of the meta-analysis, varying from − 1 (low significant changes on species distribution) to 3 (high significant changes in species distributions). The vertical dotted line represents the null effect. The size of the squares represents the size of the group effect in the analysis
Finally, our panbiogeographical analysis demonstrated that Afrotropical, Australian, Neotropical, and Nearctic realms encompass the highest number of biotic convergence zones, with most of the nodes comprising five or six taxa (Fig. 5). In contrast, the Oriental, Oceanian, Saharo-Arabian, and Sino-Japanese realms showed the lowest number of biotic convergence zones, with most of their nodes comprising only three taxa.
Potential areas for conservation of the lepidosaurians evaluated in the present study. The size of the circles is related to the number of taxa co-occurring in that area. Zoogeographical realms are identified by colors (adapted from Holt et al.,2013)
4 Discussion
Despite covering all zoogeographical realms, current literature on the impacts of climate change on Lepidosauria represents only 18% of known biodiversity, thus underrepresenting some groups and zoogeographical realms (Table 2). The Madagascan realm, for example, is known for its exceptional diversity of endemic squamates, supporting over 420 species of Gekkota (N = 121), Iguania (N = 101), Serpentes (N = 101), and Scincoidea (N = 99). In fact, the Madagascar realm boasts over 90% endemic species and genera (Raxworthy et al. 2003; Glaw and Vences 2007; Uetz et al. 2018). However, only 29 species from the Madagascan realm were evaluated among the studies, which represents around 7% of its total diversity. Also, the Oceanian realm hosts around 460 species of Anguimorpha (N = 10), Gekkota (N = 100), Iguania (N = 16), Scincoidea (N = 203), and Serpentes (N = 131), but only 13 species were evaluated among the studies, representing around 2.8% of local diversity (Uetz et al. 2018). These are also the only zoogeographical realms composed completely of island territories, demonstrating their sensitivity to other climate change factors than temperature and precipitation, such as sea level rise and erosion (Nurse et al. 2014).
Besides the scarcity of data, it is also important to highlight a possible publishing bias in sampling efforts among the studies. The researchers could have focused their analyses on species with a prior expectation of high vulnerability to climate change, in order to make recommendations for future research. This could have led to a tendency to recover negative rather than positive effects of climate change on the evaluated species. Although this is a real possibility, it does not invalidate the results obtained by the researchers and consequently those recovered in the present study, since the species were evaluated with robust methods widely used in the literature, which recovered results with high confidence levels. Therefore, the interpretation of our results was made with caution, not because of this possible bias, but because our sampling only covers 18% of known lepidosaurian diversity.
4.1 What trends have shown
The data indicate that most of the evaluated species, totaling at least 9% of the world’s Lepidosauria diversity, are likely to be negatively affected by climate change in the next few decades (2050–2080; Uetz et al. 2018). Using the phylogeny proposed by Pyron et al. (2013), we identify a relationship between phylogeny and vulnerability among lepidosaurians, with an increase in vulnerability in more recent clades (i.e., Anguimorpha + Iguania). Gekkota, the sister group to all remaining squamates, seems to be less vulnerable, while Scincoidea, Lacertoidea, and Serpentes show intermediate values and Anguimorpha and Iguania the highest vulnerabilities (Fig. 2). The exception to the pattern is Sphenodontia, which shows 100% vulnerability. Considering that there is only one extant species, this may be caused by the low diversity of this clade. Gekkota is a mainly nocturnal taxon, so its lower vulnerability may indicate that nocturnal species will be more robust to climate variation. Although these species are thermoconformers, usually implying high vulnerability to climate change (Huey et al. 2009), they are not likely to be affected by the predicted rise in temperature in the same way as other taxa.
The observed tendency of increasing phylogenetic signal in lower phylogenetic resolution groups may indicate that more conserved characteristics are more likely to drive vulnerability to climate change than more derived ones. Over deep time scales, local-scale ecological factors (e.g., microhabitat usage) are more important to clade diversification in lower phylogenetic resolutions of squamate reptiles, such as families, than large-scale factors (Bars-Closel et al. 2017). Thus, the indirect effects of climate change at regional and local levels, such as habitat fragmentation, increased predation, and decreased resource availability, could perhaps be more predictive of vulnerability to climate change at lower taxonomic resolutions than evolutionary history. Further studies are needed to test this hypothesis.
Most zoogeographical realms had at least three clades with more than 50% of the evaluated species considered vulnerable to global warming. Sensitivity to changes in environmental temperature is primarily associated with their dependence on external temperatures to regulate their body temperatures (Huey 1982). These animals may overheat when ambient temperatures increase beyond their critical limits, making their ecological and physiological processes unfeasible (Sinervo et al. 2010, 2018). However, this sensitivity may be overestimated, since other biological aspects of lepidosaurians may act as a buffer to the effects of climate change, such as genomic and phenotypic plasticity, behavioral and physiological adaptation, heritability and hormonal profiles variations, many of which have only begun to be studied in more recent years (e.g., Muñoz et al. 2014; Rodríguez et al. 2017; Dupoué et al. 2018).
The three most vulnerable realms (Afrotropical, Nearctic, and Sino-Japanese) had utterly different climatic characteristics, from tropical to subtropical and temperate regions. Besides refuting our hypothesis that species vulnerability corresponds with biogeography-related climatic conditions, this also refutes the classic hypothesis that tropical forest ectotherms should be more impacted by warming than species from higher latitudes, at least for Lepidosauria (Tewksbury et al. 2008; Huey et al. 2012).
The two least vulnerable realms (Madagascan and Oceanian) also had the lowest number of evaluated species. Besides being composed of island territories, both Madagascan and Oceanian realms are known for their great diversity of squamates, of which several species are endemic. Endemic species with narrow distribution ranges, such as those from island environments, are usually considered highly vulnerable to climate change, since local or regional changes may impact their entire distribution (Parmesan 2006; Bonino et al. 2015; Davis et al. 2015), Thus, the vulnerability level recorded for these realms among the evaluated studies may be underestimated. Studies encompassing more species are needed to confirm whether lepidosaurians from the Madagascan and Oceanian realms are indeed less vulnerable to climate change than those from other highly diverse realms, such as the Afrotropical and Neotropical.
The topology of the area cladogram (Fig. 3) shows similarities between the taxonomic composition of evaluated zoogeographical realms and potentially indicates the biogeographic history of the lepidosaurians based on their recent distribution (Morrone, 2014). Different realms sharing families and less inclusive lineages indicate that these regions were related in the past and subsequently became isolated (vicariance) or that dispersal events might have separated the previous species distribution into two independent geographic regions. Thus, one clade could be exposed to different climatic conditions due to biogeographic events implying that a single species could be more vulnerable in one area and less so in another, especially when dealing with dispersal events that can necessarily happen within a generation time (Queiroz 2005). However, our area cladogram showed a close relationship between historically distant zoogeographical realms (e.g., Oceanian and the Americas [Neotropical, Panamanian, and Nearctic]). This could reflect a bias regarding species vulnerability, which indicate a need for a more robust dataset to reconstruct the historical distribution patterns among lepidosaurians and relate them to climate change. Although the origin of Lepidosauria is estimated to have occurred during the Triassic, the recent lineages analyzed here emerged much later during the Cenozoic. Therefore, the topology shown in Fig. 3 cannot be explained by paleobiogeographical patterns of landmass movement (Hipsley et al. 2009), suggesting that dispersal events might have played a significant role in shaping the current distribution of Lepidosauria.
As mentioned above, lepidosaurians will be negatively affected by climate change, and species from all zoogeographical realms might be negatively affected in the near future. This may indicate that adaptability to local climate is not affected by the historical distribution of lepidosaurian families. It is possible that lepidosaurians in the past were able to adapt to natural climate change occurring over long timespans but are likely to be endangered now due to rapid temperature variation.
Our meta-analysis indicates that climate change is affecting the geographical distribution of taxa on a global scale, with no effects of heterogeneity of the effect size. This corroborates the results from Sinervo et al. (2010), which derived global extinction projections for a large dataset comprising 34 families around the world. Currently, most of the studies on expansions or contractions of species distributions have focused on a restricted number of species (e.g., Kubisch et al. 2016; Park et al. 2017; Pontes-da-Silva et al. 2018) or on a restricted area (e.g., Aragón et al. 2010; Cabrelli et al. 2014; El-Gabbas et al. 2016). These studies also reinforce the results of the comprehensive approach developed by Sinervo et al. (2010). Recent studies are employing more complex models, which include environmental, biological, and ecological parameters to increase the degree of refinement and reliability of the predictions, as predicted by Huey et al., 2010; e.g., Dupoué et al. 2017; Flesch et al. 2017; Carlo et al. 2018). Despite these advances, there is still no consensus regarding the best model to make an accurate prediction of future changes on species distribution due to climate variation (Warren et al. 2014; Araújo et al. 2019). Thus, evaluations on species distribution modeling should be interpreted with caution.
Australian scincoideans demonstrated the most significant change in their geographical distribution due to global warming compared to the other taxa. This may be due to the high number of species (169 vulnerable, 146 non-vulnerable) evaluated by a single study (Cabrelli and Hughes 2015), in which the authors found low divergence among the methods used to calculate the ROC. This also explains the size of the effect of both vulnerable and non-vulnerable Australian scincoideans in the analysis.
We observed that the effects of vulnerable and non-vulnerable taxa on the meta-analysis differed markedly among the zoogeographical realms. This may be due to the substantial differences between the number of evaluated species for each taxa and realm by the different studies (e.g., Cabrelli and Hughes 2015; Srinivasulu and Srinivaslu, 2016). Also, the level of significance of the vulnerable taxa was generally lower than those from non-vulnerable taxa, which may indicate that the degree of vulnerability of the species must be better evaluated and interpreted with caution.
Our results show that areas with higher potential for conservation due to the high spatial concentration of diversity, such as Afrotropical and Nearctic regions, also presented a greater number of vulnerable species. Although we identify several areas around the globe that represent biotic convergence zones, our spatial resolution is not precise since we searched for a general pattern around the globe. Planning policies and management strategies for species conservation in these areas are needed to preserve the habitat of vulnerable species and will require refinement of the analysis at a local scale (Martínez-Freiría et al. 2013). However, the decision-making process focused on buffering the effects of climate change on natural populations also requires that factors other than species vulnerability be considered (Guisan et al. 2013). For example, Popescu et al. (2013) used a modeling approach to identify a conservation priority area for Romanian herpetofauna based on the species distribution dynamics in a climate change scenario. Martínez-Freiría et al. (2013) identified altitude and latitude as geographical factors related to species richness in a climate change scenario and concluded that high altitudes and/or latitudes are most species-rich in Moroccan endemic reptiles. However, both studies focused on a local scale.
Although several studies have focused on the assessment of reptile extinction risk around the globe (e.g., Sinervo et al. 2010; Meiri et al. 2013; Böhm et al. 2016), macroecological analyses on this subject are still rare due to the paucity of available data (Tingley et al. 2016). Thus, this study represents the first effort to determine the priority areas for large-scale conservation of lepidosaurians identified as vulnerable (or not) to climate change in the literature. This was accomplished by a meta-analysis of all available studies on this topic up to January 2018. Future studies are needed to validate our macroecological results and thus improve the planning policies and management strategies for species conservation around the globe.
5 Conclusions
We conclude that there is a relationship between phylogeny and vulnerability among higher hierarchical lineages of Lepidosauria, with an increase in vulnerability in more recent clades. This trend is probably due to conserved characteristics in deep phylogenetic history. Also, species from environments with different climatic characteristics are equally vulnerable, indicating that a species’ vulnerability does not correspond with biogeographic-related climatic conditions.
Lacking studies focusing on species from several areas, most of the zoogeographical realms were not sampled homogenously. Some of them can be considered subsampled in relation to the known local diversity. Areas with higher potential for conservation, due to the high spatial concentration of diversity, also presented a greater number of vulnerable species. This indicates that these areas are possible targets for conservation at a larger scale and may help to identify especially diverse areas for conservation efforts at a small scale aimed to buffer the effects of climate change on local populations.
5.1 Trends for future studies
-
Evaluations of species distribution modeling should be made more carefully, and the degree of species vulnerability should be better described and interpreted with caution.
-
The lack of research on Madagascan and Oceanian realms, both island environments with a high degree of endemicity, is likely to result in underestimates of their vulnerability to climate change. Thus, future studies should focus on assessing species’ vulnerability to climate change in these environments, aiming to decrease this sampling bias in comparison to other zoogeographical realms.
-
The investigation of potential areas for lepidosaurian conservation on a broad zoogeographical scenario, considering the current global status of species vulnerability and phylogenetic diversity on different areas, should improve the knowledge about biodiversity and conservation of this group.
-
Further studies are needed to test the hypothesis that the indirect effects of climate change at local scales are more effective in dictating the pathway of evolutionary process than the direct effect of temperature variation among less inclusive taxonomic levels of Lepidosauria.
-
The influence of climate change on the majority of the lepidosaurian species remains unknown. Thus, further studies are needed on those areas with a lack of data, such as Canada, Russia, Congo, and the Middle East, to homogenize sampling and reinforce the patterns of climate change effects on Lepidosauria among the zoogeographical realms.
References
Aragón P, Rodríguez MA, Olalla-Tárraga MA, Lobo JM (2010) Predicted impact of climate change on threatened terrestrial vertebrates in Central Spain highlights differences between endotherms and ectotherms. Anim Conserv 13:363–373. https://doi.org/10.1111/j.1469-1795.2009.00343.x
Araújo MB, Anderson RP, Márcia Barbosa A et al (2019) Standards for distribution models in biodiversity assessments. Sci Adv 5:eaat4858. https://doi.org/10.1126/sciadv.aat4858
Bars-Closel M, Kohlsdorf T, Moen DS, Wiens JJ (2017) Diversification rates are more strongly related to microhabitat than climate in squamate reptiles (lizards and snakes). Evolution 71:2243–2261. https://doi.org/10.1111/evo.13305
Bellard C, Bertelsmeier C, Leadley P et al (2012) Impacts of climate change on the future of biodiversity. Ecol Lett 15:365–377. https://doi.org/10.1111/j.1461-0248.2011.01736.x
Blomberg SP, Garland T, Ives AR (2003) Testing for phylogenetic signal in comparative data: behavioral traits are more labile. Evolution 57:717–745. https://doi.org/10.1111/j.0014-3820.2003.tb00285.x
Böhm M, Cook D, Ma H et al (2016) Hot and bothered: using trait-based approaches to assess climate change vulnerability in reptiles. Biol Conserv 204:32–41. https://doi.org/10.1016/j.biocon.2016.06.002
Bonino MF, Moreno Azócar DL, Schulte JA et al (2015) Thermal sensitivity of cold climate lizards and the importance of distributional ranges. Zoology 118:281–290. https://doi.org/10.1016/j.zool.2015.03.001
Cabrelli AL, Hughes L (2015) Assessing the vulnerability of Australian skinks to climate change. Clim Chang 130:223–233. https://doi.org/10.1007/s10584-015-1358-6
Cabrelli A, Stow A, Hughes L (2014) A framework for assessing the vulnerability of species to climate change: a case study of the Australian elapid snakes. Biodivers Conserv. https://doi.org/10.1007/s10531-014-0760-0
Cahill AE, Aiello-lammens ME, Fisher-Reid MC et al (2012) How does climate change cause extinction? Proc R Soc B Biol Sci 280:20121890–20121890. https://doi.org/10.1098/rspb.2012.1890
Carlo MA, Riddell EA, Levy O, Sears MW (2018) Recurrent sublethal warming reduces embryonic survival, inhibits juvenile growth, and alters species distribution projections under climate change. Ecol Lett 21:104–116. https://doi.org/10.1111/ele.12877
Cavalcanti MJ (2009) Croizat: a software package for quantitative analysis in panbiogeography. Biogeografía 4:4–6
Ceballos G, Garcia A, Pringle RM et al (2015) Accelerated modern human – induced species losses : entering the sixth mass extinction. Sci Adv 1:1–6. https://doi.org/10.1126/sciadv.1400253
Chamaillé-Jammes S, Massot M, Aragón P, Clobert J (2006) Global warming and positive fitness response in mountain populations of common lizards Lacerta vivipara. Glob Chang Biol 12:392–402. https://doi.org/10.1111/j.1365-2486.2005.01088.x
Core Team R (2018) R: a language and environment for statistical computing. R Foundation for Statistical Computing, Vienna, Austria
Croizat L, Nelson G, Rosen D (1974) Centers of origin and related concepts. Syst Zool 23:265–287. https://doi.org/10.2307/2412139
Davis MA, Douglas MR, Webb CT et al (2015) Nowhere to go but up: impacts of climate change on demographics of a short-range endemic (Crotalus willardi obscurus) in the sky-islands of southwestern North America. PLoS One 10. https://doi.org/10.1371/journal.pone.0131067
Diele-Viegas LM, Rocha CFD (2018) Unraveling the influences of climate change in Lepidosauria (Reptilia). J Therm Biol 78:401–414. https://doi.org/10.1016/j.jtherbio.2018.11.005
Diele-Viegas LM, Vitt LJ, Sinervo B et al (2018) Thermal physiology of Amazonian lizards (Reptilia: Squamata). PLoS One 13. https://doi.org/10.1371/journal.pone.0192834
Dupoué A, Rutschmann A, Le Galliard JF et al (2017) Shorter telomeres precede population extinction in wild lizards. Sci Rep 7:1–8. https://doi.org/10.1038/s41598-017-17323-z
Dupoué A, Rutschmann A, Le Galliard JF et al (2018) Reduction in baseline corticosterone secretion correlates with climate warming and drying across wild lizard populations. J Anim Ecol 87:1331–1341. https://doi.org/10.1111/1365-2656.12843
Echeverría-Londoño S, Miranda-Esquivel DR (2011) Martitracks: a geometrical approach for identifying geographical patterns of distribution. PLoS One 6. https://doi.org/10.1371/journal.pone.0018460
El-Gabbas A, Baha El Din S, Zalat S, Gilbert F (2016) Conserving Egypt’s reptiles under climate change. J Arid Environ 127:211–221. https://doi.org/10.1016/j.jaridenv.2015.12.007
Field C, Barros V, Mach K, Mastrandrea M (2014) Climate change 2014: impacts, adaptation, and vulnerability. … II contribution to the IPCC …
Flesch AD, Rosen PC, Holm P (2017) Long-term changes in abundances of Sonoran Desert lizards reveal complex responses to climatic variation. Glob Chang Biol 23:5492–5508. https://doi.org/10.1111/gcb.13813
Fritz SA, Purvis A (2010) Selectivity in mammalian extinction risk and threat types: a new measure of phylogenetic signal strength in binary traits. Conserv Biol 24:1042–1051. https://doi.org/10.1111/j.1523-1739.2010.01455.x
Garcia RA, Cabeza M, Rahbek C, Araújo MB (2014) Multiple dimensions of climate change and their implications for biodiversity. Science 344. https://doi.org/10.1126/science.1247579
Glaw M, Vences F (2007) A field guide to the amphibians and reptiles of Madagascar. Third Edition Köln, Vences & Glaw Verlag, 496 pp
Goloboff PA, Farris JS, Nixon KC (2008) TNT, a free program for phylogenetic analysis. Cladistics 24:774–789. https://doi.org/10.1111/j.1096-0031.2008.00217.x
Graham CH, Storch D, Machac A (2018) Phylogenetic scale in ecology and evolution. Glob Ecol Biogeogr 27:175–187. https://doi.org/10.1111/geb.12686
Guisan A, Tingley R, Baumgartner JB et al (2013) Predicting species distributions for conservation decisions. Ecol Lett 16:1424–1435. https://doi.org/10.1111/ele.12189
Heads M (2004) What is a node? J Biogeogr 31:1883–1891. https://doi.org/10.1111/j.1365-2699.2004.01201.x
Higgins JPT, Thompson SG (2002) Quantifying heterogeneity in a meta-analysis. Stat Med 21:1539–1558. https://doi.org/10.1002/sim.1186
Hipsley CA, Himmelmann L, Metzler D, Müller J (2009) Integration of Bayesian molecular clock methods and fossil-based soft bounds reveals early Cenozoic origin of African lacertid lizards. BMC Evol Biol 9:151. https://doi.org/10.1186/1471-2148-9-151
Holt BG, Lessard JP, Borregaard MK et al (2013) An update of Wallace’s zoogeographic regions of the world. Science 339:74–78. https://doi.org/10.1126/science.1228282
Huey RB (1982) Temperature, physiology, and the ecology of reptiles. Biology of the Reptilia Vol 12 physiology C physiological. Ecology 12:25–91. https://doi.org/10.1016/j.dsr.2014.07.003
Huey RB, Deutsch CA, Tewksbury JJ et al (2009) Why tropical forest lizards are vulnerable to climate warming. Proc R Soc B Biol Sci 276:1939–1948. https://doi.org/10.1098/rspb.2008.1957
Huey RB, Losos JB, Moritz C (2010) Ecology. Are lizards toast? Science 328:832–833. https://doi.org/10.1126/science.1190374
Huey RB, Kearney MR, Krockenberger A et al (2012) Predicting organismal vulnerability to climate warming: roles of behaviour, physiology and adaptation. Philos Trans R Soc Lond B Biol Sci 367:1665–1679. https://doi.org/10.1098/rstb.2012.0005
IPCC (2018) Global warming of 1.5°C. summary for policymakers
Jetz W, Fine PVA (2012) Global gradients in vertebrate diversity predicted by historical area-productivity dynamics and contemporary environment. PLoS Biol 10. https://doi.org/10.1371/journal.pbio.1001292
Jiménez-Valverde A (2012) Insights into the area under the receiver operating characteristic curve (AUC) as a discrimination measure in species distribution modelling. Glob Ecol Biogeogr 21(4):498–507. https://doi.org/10.1111/j.1466-8238.2011.00683.x
Kubisch EL, Fernández JB, Ibargüengoytía NR (2016) Vulnerability to climate warming of Liolaemus pictus (Squamata, Liolaemidae), a lizard from the cold temperate climate in Patagonia, Argentina. J Comp Physiol B 186:243–253. https://doi.org/10.1007/s00360-015-0952-2
Maddison WP, Maddison DR (2018) Mesquite: a modular system for evolutionary analysis. http://mesquiteproject.org
Martínez-Freiría F, Argaz H, Fahd S, Brito JC (2013) Climate change is predicted to negatively influence Moroccan endemic reptile richness. Implications for conservation in protected areas. Naturwissenschaften 100:877–889. https://doi.org/10.1007/s00114-013-1088-4
Meiri S, Bauer AM, Chirio L et al (2013) Are lizards feeling the heat? A tale of ecology and evolution under two temperatures: lizard body and environmental temperatures. Glob Ecol Biogeogr 22:834–845. https://doi.org/10.1111/geb.12053
Monasterio C, Shoo LP, Salvador A et al (2013) High temperature constrains reproductive success in a temperate lizard: implications for distribution range limits and the impacts of climate change. J Zool 291:136–145. https://doi.org/10.1111/jzo.12057
Morrone JJ (2004) Homología Biogeográfica: Las Coordenadas Espaciales de la Vida. Cuadernos del Instituto de Biologia Mexico: Instituto de Biología, Universidad Nacional Autonoma de Mexico 37
Morrone JJ (2014) Parsimony Analysis of Endemicity (PAE) revisited. J Biogeogr 41:842–854. https://doi.org/10.1111/jbi.12251
Muñoz MM, Stimola MA, Algar AC et al (2014) Evolutionary stasis and lability in thermal physiology in a group of tropical lizards. Proc R Soc B 281:20132433. https://doi.org/10.1098/rspb.2013.2433
Nihei SS (2006) Misconceptions about parsimony analysis of endemicity. J Biogeogr 33:2099–2106. https://doi.org/10.1111/j.1365-2699.2006.01619.x
Nurse LA, RF ML, Agard J et al (2014) Climate Change 2014: Impacts, Adaptation, and Vulnerability. Part B: Regional Aspects. Contribution of Working Group II to the Fifth Assessment Report of the Intergovernmental Panel on Climate Change. In: Barros VR, Field CB, Dokken DJ, Mastrandrea MD, Mach KJ, Bilir TE, Chatterjee M, Ebi KL, Estrada YO, Genova RC, Girma B, Kissel ES, Levy AN, MacCracken S, Mastrandrea PR, White LL (eds) Small islands. Cambridge University Press, Cambridge, United Kingdom and New York, NY, USA, pp 1613–1654
Pagel M (1999) Inferring the historical patterns of biological evolution. Nature 401:877–884. https://doi.org/10.1038/44766
Park J, Kim IH, Fong JJ et al (2017) Northward dispersal of sea kraits (Laticauda semifasciata) beyond their typical range. PLoS One 12:1–9. https://doi.org/10.1371/journal.pone.0179871
Parmesan C (2006) Ecological and evolutionary responses to recent climate change. Annu Rev Ecol Evol Syst 37:637–669. https://doi.org/10.1146/annurev.ecolsys.37.091305.110100
Pereira HM, Leadley PW, Proença V et al (2010) Scenarios for global biodiversity in the 21st century. Science 330:1496–1501. https://doi.org/10.1126/science.1196624
Pontes-da-Silva E, Magnusson WE, Sinervo B et al (2018) Extinction risks forced by climatic change and intraspecific variation in the thermal physiology of a tropical lizard. J Therm Biol:73. https://doi.org/10.1016/j.jtherbio.2018.01.013
Popescu VD, Rozylowicz L, Cogãlniceanu D et al (2013) Moving into protected areas? Setting conservation priorities for Romanian reptiles and amphibians at risk from climate change. PLoS One 8. https://doi.org/10.1371/journal.pone.0079330
Pyron RA, Burbrink FT, Wiens JJ (2013) A phylogeny and revised classification of Squamata, including 4161 species of lizards and snakes. BMC Evol Biol 13:93. https://doi.org/10.1186/1471-2148-13-93
Queiroz A (2005) The resurrection of oceanic dispersal in historical biogeography. Trends Ecol Evol 20:68–73. https://doi.org/10.1016/j.tree.2004.11.006
Raxworthy CJ, Martinez-Meyer E, Horning N et al (2003) Predicting distributions of known and unknown reptile species in Madagascar. Nature 426:837–841. https://doi.org/10.1038/nature02205
Rodríguez A, Rusciano T, Hamilton R et al (2017) Genomic and phenotypic signatures of climate adaptation in an Anolis lizard. Ecol Evol 7:6390–6403. https://doi.org/10.1002/ece3.2985
Roll U, Feldman A, Novosolov M et al (2017) The global distribution of tetrapods reveals a need for targeted reptile conservation. Nat Ecol Evol 1:1677–1682. https://doi.org/10.1038/s41559-017-0332-2
Salgado JF (2018) Transforming the area under the normal curve (AUC) into Cohen’s d, Pearson’s rpb, odds-ratio, and natural log odds-ratio: two conversion tables. European Journal of Psychology Applied to Legal Context 10:35–47. https://doi.org/10.5093/ejpalc2018a5
Sinervo B, Méndez-de-la-Cruz F, Miles DB et al (2010) Erosion of lizard diversity by climate change and altered thermal niches. Science 328:894–899. https://doi.org/10.1126/science.1184695
Sinervo B, Miles DB, Yayong WU et al (2018) Climate change, thermal niches, extinction risk and maternal-effect rescue of toad-headed lizards, Phrynocephalus, in thermal extremes of the Arabian Peninsula to the Qinghai—Tibetan Plateau. Integrative Zoology 13:450–470. https://doi.org/10.1111/1749-4877.12315
Srinivasulu A, Srinivaslu C (2016) All that glitters is not gold: a projected distribution of the endemic Indian golden gecko Calodactyloides aureus (Reptilia: Squamata: Gekkonidae) indicates a major range shrinkage due to the future climate change. Journal of Threatened Taxa 8:8883–8892
Tewksbury JJ, Huey RB, Deutsch C a (2008) Ecology. Putting the heat on tropical animals. Science 320:1296–1297. https://doi.org/10.1126/science.1159328
Tingley R, Meiri S, Chapple DG (2016) Addressing knowledge gaps in reptile conservation. Biol Conserv 204:1–5. https://doi.org/10.1016/j.biocon.2016.07.021
Uetz P, Freed P, Hošek J, (eds.) (2018) The Reptile Database
Viechtbauer W (2010) Conducting meta-analyses in R with the metafor package. J Stat Softw 36:1–48
Warren DL, Wright AN, Seifert SN, Shaffer HB (2014) Incorporating model complexity and spatial sampling bias into ecological niche models of climate change risks faced by 90 California vertebrate species of concern. Divers Distrib 20:334–343. https://doi.org/10.1111/ddi.12160
Williams JB, Shobrak M, Wilms TM et al (2012) Climate change and animals in Saudi Arabia. Saudi J Biol Sci 19:121–130. https://doi.org/10.1016/j.sjbs.2011.12.004
Acknowledgments
We are grateful to I. Raposo for his thoughtful comments on the manuscript; to T. Motta-Tavares for her analytical support; and to W. Edwards, Dr. C. Early, and Dr. D. B. Miles for proofreading the manuscript. We also thank Universidade do Estado do Rio de Janeiro (UERJ) and Ohio University for logistic support.
Funding
LMDV and RTF received fellowship from Coordenação de Aperfeiçoamento de Pessoal de Nível Superior (CAPES, process No. 1684534 and No. 1683216). LMDV received fellowship from Conselho Nacional de Desenvolvimento Científico e Tecnológico (CNPq, process No. 380601/2019-2) and benefitted from grants of CAPES through “Programa de Doutorado Sanduíche no Exterior” (process No. 88881.188452/2018-01). RTF received fellowship from Fundação Carlos Chagas Filho de Amparo à Pesquisa do Estado do Rio de Janeiro (FAPERJ; process No. E-26/ 202.426/2018) and a departmental fellowship from the Department of Earth and Environmental Sciences of the University of Michigan. CFDR benefitted from grants of CNPq (process No. 302974/2015-6 and 424473/2016-0) and FAPERJ through “Cientistas do Nosso Estado” Program (process No. E-26/202.920.2015) and FAPERJ/Biota Program (process No. E-26/010.001639/2014).
Author information
Authors and Affiliations
Corresponding author
Additional information
Publisher’s note
Springer Nature remains neutral with regard to jurisdictional claims in published maps and institutional affiliations.
Rights and permissions
About this article
Cite this article
Diele-Viegas, L.M., Figueroa, R.T., Vilela, B. et al. Are reptiles toast? A worldwide evaluation of Lepidosauria vulnerability to climate change. Climatic Change 159, 581–599 (2020). https://doi.org/10.1007/s10584-020-02687-5
Received:
Accepted:
Published:
Issue Date:
DOI: https://doi.org/10.1007/s10584-020-02687-5