Abstract
Recently our group used oligodendrocyte progenitor cells (OPCs) as appropriate model cells to pinpoint the mechanism of the progress of neurodegenerative disorders. In the present study, we focused on the therapeutic role of osteopontin (OPN), a secreted glycosylated phosphoprotein, involved in a number of physiological events including bone formation and remodeling, immune responses, and tumor progression. Protective role of OPN, as a negative regulator of tumorigenesis, has already been clarified. Human embryonic stem cell-derived OPCs were pretreated with OPN before induction of apoptosis by H2O2. Data indicated that OPN prohibited cell death and enhanced OPC viability. This effect is achieved through reduction of apoptosis and induction of anti-apoptosis markers. In addition OPN induces expression of several integrin subunits, responsible for OPN interaction. Notably, our findings showed that expression of αV β1/β3/β5 and β8 integrins increased in response to OPN, while treatment with H2O2 down-regulated αV β1/β5 and β8 integrins expression significantly. In conclusion, OPN may act via αV integrin signaling and trigger suppression of P53-dependent apoptotic cascades. Therefore OPN therapy may be considered as a feasible process to prevent progress of neurodegenerative diseases in human.
Similar content being viewed by others
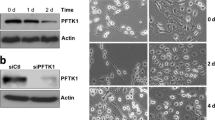
Avoid common mistakes on your manuscript.
Introduction
In nervous system, axons are insulated by myelin sheaths for efficient transduction of electrical impulses (Miron et al. 2011). Therefore, any destruction in myelin sheaths generates severe conditions termed myelopathies. Inflammatory responses are major pathogenic causes which play significant roles in the progress of myelin degeneration diseases (Glass et al. 2010). Multiple sclerosis (MS) is a chronic autoimmune disease which is associated with inflammation, demyelination, and axon collapse, in which repetitive inflammatory attacks to the myelin sheath give rises to neuron degeneration, and gradually results in sclerotic plaques in central nervous system (CNS) (Dhib-Jalbut 2007). Inversely, remyelination is a natural recovery process by which demyelinated axons are reinvested with new myelin sheaths. Remyelination is a complicated process in CNS, mediated by OPCs which migrate into the lesion sites and proliferate, then subsequently differentiate into mature oligodendrocytes (OLs). Therefore, a significant interaction between OPCs and axonal tracts of neurons is crucial for the completion of successful remyelination (Watzlawik et al. 2010; Stangel and Hartung 2002; Buchet and Baron-Van Evercooren 2009).
Evidence has indicated the involvement of reactive oxygen species (ROS) such as hydrogen peroxide (H2O2) in pathogenesis of MS lesions. H2O2 is a by-product of inflammatory responses of macrophage, triggers myelin degradation, hence causes apoptosis of OPCs and OLs leading to neuronal and axonal damage (Wittmann et al. 2012; Baud et al. 2004). Despite exclusive background studies on process of remyelination, the molecular mechanism underlying OPCs biology and survival is still unclear. Understanding of these key processes may have a great value for designing new therapeutics approaches for demyelinating diseases.
In the past few years, extensive studies have implicated that several signaling pathways specifically, integrin signaling has been linked to OPCs survival and is responsible for OPC-mediated remyelination (O’Meara et al. 2010; Milner and Ffrench-Constant 1994; Zhao et al. 2009; Scatena et al. 1998). The cell fate decision is directed by components of the extracellular matrix (ECM) (Sorokin 2010). Hence, functional interaction between cell adhesion receptors expressed by OPCs and ECM is crucial for anti-apoptotic pathways that preserve cell viability in response to apoptosis-induced oxidative stress by H2O2 that activates the intrinsic death pathway and leads to the transcriptional activity of p53. Most important adhesion receptors play a major role in signaling pathways. Among them, integrins are important as they trigger the expression and activity of several members of Bcl-2 family proteins and govern inhibition of p53-dependent apoptotic pathways (Sorokin 2010; Mouzannar et al. 2001; Hirrlinger et al. 2002; Kanduc et al. 2002; Polager and Ginsberg 2009).
Integrin receptors are heterodimeric membrane proteins containing α and β subunits. Mammalian cell contains eighteen types of α and β subunits. Combination of one α subunit with one β subunit makes 24 different types of integrin receptors. Upon binding of ECM ligands to integrins, assembling of the integrin cytoplasmic domains occurs which induces migration, proliferation, and cell survival, for remyelination. OPCs express αv subunit of integrin in a complex with β subunit no. 1, 3, 5, and 8 in a specific set of developmentally regulated pattern depending on the stage of cellular differentiation. These cells also express α6 subunit. It is demonstrated that α subunits specify integrin complexes to specific ECM components (O’Meara et al. 2010; Milner and Ffrench-Constant 1994; Zhao et al. 2009). For example, αv subunits specify integrin attachment to Arg–Gly–Asp (RGD) sequence—containing ligands such as Osteopontin (OPN) (Sodek et al. 2000). Osteopontin is a matricellular class of phosphorylated acidic glycoprotein that has several pleiotropic functions in physiological events, including inflammatory responses (Denhardt et al. 2001). Previous studies have indicated that OPN is one of the five most abundant transcripts, which is expressed extensively in MS lesions (Hur et al. 2007). However, the detailed roles of the protein in CNS remain unclear. In vivo studies for evaluation of OPN expression showed that OPN is secreted by astrocytes and microglial cells during demyelination, suggesting a novel stimulator role for OPN in promoting myelination and demyelination. Therefore, this factor enhances the expression of myelin basic protein (MBP) and increases myelin membrane formation, as well as differentiation and migration of OPCs (Zhao et al. 2008; Selvaraju et al. 2004; Merrill et al. 2003). OPN interacts with integrins αv (β1, β3, or β5) and (α4, α5, α8, or α9) β1 and induces specific integrin signaling in cell. Several studies have demonstrated that OPN delivers a pro-survival, anti-apoptotic signal to the cell. Further researches have indicated significant up-regulation of αv integrin expression during remyelination, strongly suggesting a significant role for αv integrins in regeneration of nervous tissues (Geissinger et al. 2002; Schordan et al. 2011; Song et al. 2008; Burdo et al. 2007; Behera et al. 2009). Accordingly, the aim of this study was to assess whether OPN plays a specific role in scavenging pathways in response to H2O2-induced apoptotic OPCs.
Materials and Methods
Ethical Standards
Permission to study human cell line was approved by the Royan institute ethics committee and therefore was performed in accordance with the ethical standards laid down in the 1964 Declaration of Helsinki and its later amendments.
Human Embryonic Stem Cell (hESC) Culture and Differentiation into Oligodendrocyte Lineage Cells
Royan H6 hESCs line was used in this study. This cell line was acquired from Department of Stem Cells and Developmental Biology at Cell Science Research Center (Royan Institute for Stem Cell Biology and Technology, Tehran, Iran) as described (Peymani et al. 2017; Pouya et al. 2011). Cells were cultured in GRM medium including DMEM/F12 supplemented with 2% (v/v) B27, 1% (v/v) N2, platelet-derived growth factor (PDGF) (Sigma, P3076), and epidermal growth factor (EGF, 20 ng/mL; Sigma) (Peymani et al. 2017), E9644. Differentiation procedure into OPCs and OLs lineage cells (Fig. 1) was started according to the previous protocol (Peymani et al. 2017; Pouya et al. 2011).
Schematic representation of hESCs differentiation to OPCs. a HESCs were cultured in specific medium to produce EBs (stage 1). EBs were treated with a cocktail of EGF and RA for neural differentiation (stage 2) and gradually the size of the EBs increased (stage 3). EBs were plated again on matrigel in the presence of EGF to generate OPCs as described earlier (stage 4) (Peymani et al. 2017). Finally cells displayed oligodendroglial morphology (stage 5). b Expression level of OPCs markers, PDGFR and OLIG2 were assessed both in OPCs and hESCs (RH6 cell line). As shown there was a sharp increase in OLIG2 and PDGFR in OPCs with respect to the hESCs. All relative expressions were quantified and normalized with GAPDH transcript level. Represented value bars are the mean of triplicate independent experiments ± SEM. Bar is 100 µm
H2O2 Treatment
Differentiated OPCs were seeded in 96-well tissue culture plate which had been coated with matrigel (Sigma-Aldrich, E1270) and incubated at 37 °C overnight to allow cell adherence. Then, cells were treated with H2O2 in a serial concentration at 100, 200, 400, 800, 1600, and 3200 μM. One day after treatment, cell survival assay was performed. Finally, a concentration of 3200 μM was selected as optimal amount of H2O2 capable to decrease 50% of OPCs survival.
To examine the preservative effects of OPN, matrigel-coated culture plates were coated again with a serial concentration of recombinant OPN (R&D Systems) in a range of 12.5, 50, 100, and 200 ng/mL for 1 h at 4 °C prior to cell seeding. Oxidative stress was induced by addition of H2O2 (3200 μM). Next day, cell viability assay was carried out to find effective concentration of OPN to preserve the cell viability. As the most effective concentration of OPN was 12.5 ng/mL, this concentration was used for the rest of experiments.
Cell Survival Assay
One day after treatment, cell viability was measured by a colorimetric assay using MTS assay as described (Peymani et al. 2017).
Caspase Activity Assay
Caspase activation was measured with Caspa-TagTM Caspase-3/7 In Situ Assay Kit, Fluorescein (APT403, Millipore) according to the manufacturer’s protocol. Briefly, 24 h after treatment, cells were transferred to sterile tubes and incubated with FLICA reagent as permeable irreversible caspase inhibitor in darkness. One hour after incubation, cells were washed twice and incubated with PI solution. Cellular viability was assessed by FACS Caliber flow cytometer (BD Biosciences), and data were processed according to ModFit LT version 4.0 program as reported previously (Kajabadi et al. 2015).
RNA Isolation and Real-Time Quantitative PCR (RT-qPCR)
RNA isolation and cDNA production was carried out as described (Peymani et al. 2017). Expression of integrin subunits αv and β/1/3/5/8 at three stages of the oligodendrocyte differentiation was quantified by RT-qPCR as well as several apoptotic genes including P53, BCL-2, BCL-XL, BAX, and BID using specific primers (Table 1).
Statistical Analysis
Microsoft Excel (2010) and SPSS software were used to express data as mean ± SEM (standard error of mean) from three independent replicated observations. One-way analysis of variance (ANOVA) was performed to identify statistical differences among data at the level of p < 0.05.
Results
Differentiation and Characterization of hESCs into OPCs and OLs
Figure 1a illustrates the schematic representation of the differentiation procedure and the morphology of cells at different stages. According to the protocol that reported previously (Peymani et al. 2017; Pouya et al. 2011), hESCs were passaged at start point and cultured to form EBs (Stage 1). At the next stage, the induction of neuroglial differentiation was performed by treating with retinoic acid (RA; Sigma) for nine days (Stage 2). This process results in the appearance of yellow spheres. Cellular proliferation step started after removal of RA, and yellow spheres were cultured in EGF supplemented GRM with for next 18 days. In this stage, the size of EBs increased (Stage 3). On day 28 from the starting point, free-floating spheres were plated on matrigel followed by adherence of EBs. The differentiated cells started to migrate outward from the plated spheres and were proliferated in the culture. Morphology of these cells was similar to immature bipolar and multipolar OPCs (Stage 4). To estimate OLs maturation, EGF was removed from the medium and cells were re-plated on poly-L-laminin (PLL)/Lam (laminin) substrate for three weeks. Ultimately, plated cells displayed oligodendroglial morphology (stage 5) (Fig. 1a). Differentiated bipolar cells obtained from stage 4 were analyzed by immunofluorescence staining for expression of typical markers of OPCs. As previously described, expression of the OPCs markers, A2B5 and PDGFRα, was evident in the cell while no GFAP-positive cell was detected. Also myelination was observed in OLs (Peymani et al. 2017; Pouya et al. 2011). Additionally, RT-qPCR for OLIG2 and PDGFR confirmed the recent data obtained by immunostaining (Fig. 1b). Data confirmed appropriate OPCs induction in this study.
Preservative Effects of OPN on Survival of H2O2 Treated OPCs
Initially, cells were treated with different concentrations of H2O2 as depicted in Fig. 2a. MTS assay showed that H2O2 induced a dose-dependent decrease in OPCs viability. Maximal effect of H2O2 on cell survival was achieved at 3200 μM of H2O2 (Fig. 2b). This concentration of H2O2 was repeated in the rest of experiments. Next, cells were pretreated with a several OPN concentrations prior to H2O2 exposure to avoid toxicological concentrations of OPN. Results indicated that OPN had no significant cytotoxicity effects on cells when used at concentration of 12.5 ng/mL. (Fig. 2c). This concentration of OPN prevented cytotoxicity of H2O2 (approximately 25% with respect to concentration of 0 ng/mL of OPN) (Fig. 2d). Therefore, 12.5 ng/mL of OPN was used for the rest of the experiments.
H2O2 modulated viability of OPCs. a A schematic diagram of cell pretreatment with OPN prevented the cell toxicity effect of H2O2. b OPCs were treated with H2O2, 24 h before cell number assay. As evident, maximal effect of H2O2 on cell survival was achieved at 3200 μM. c Cells were pretreated with a several OPN concentrations prior to H2O2. As shown OPN had no significant cytotoxicity effects on cells when was used at a concentration of 12.5 ng/mL. d OPN enhanced the survival rate of H2O2-treated OPCs. As evident, 12.5 ng/mL concentrations of OPN reversed the cytotoxic effect H2O2 (3200 μM). Represented value bars are the mean of triplicate independent experiments ± SEM. Similar alphabets indicate significant difference between the same samples at p < 0.05
Effects of OPN on H2O2-Induced Caspase Activation in OPCs
Caspase activation is considered as an important event in induction of apoptotic pathways (Kanduc et al. 2002). Therefore, caspase activity assay was performed to indicate whether OPN (12.5 ng/mL) prevented caspase activation of H2O2 (3200 μM)-induced apoptosis. As shown in Fig. 4, activity of caspase 3 was low in untreated OPCs (approximately 14%) while H2O2 treatment induced this activity significantly (approximately 92%). Interestingly, OPN pretreatment remarkably decreased the rate of caspase 3 activation near to 58% (Fig. 3).
Flow cytometry assessments indicated that OPN prevented inductive effects of H2O2 mediated induced caspase activation. Caspase activity assay in untreated cells (a) was compared with treated OPCs with H2O2 (3200 μM) in ± OPN (12.5 ng/mL) (b, c). As shown, activity of caspase 3 was low in untreated OPCs (approximately 14%, a), while H2O2 treatment induced this activity significantly (approximately 92%, b). Interestingly, OPN pretreatment remarkably decreased the rate of caspase 3 activation near to 58% (c)
OPN Protected Cells from H2O2-Induced Apoptosis via Modulation Apoptotic Genes
Since H2O2 is one of the inducers of P53-dependent apoptosis (Mouzannar et al. 2001), we evaluated the expression level of P53 in OPCs culture. Results revealed increased transcript level of P53 in H2O2 (3200 μM)-treated OPCs, whereas OPN (12.5 ng/mL) reversed this up-regulation (Fig. 5). Previous studies indicated once P53 was activated; it regulates the expression level of Bcl-2 family members (pro-apoptotic and anti-apoptotic genes) (Mouzannar et al. 2001). Here, we demonstrated increased expression levels of pro-apoptotic genes (BAX and BID) in H2O2-treated OPCs, whereas OPN significantly decreased the expression level of anti-apoptotic genes. In contrast, transcript levels of BCL-2 and BCL-XL (anti-apoptotic genes) were suppressed remarkably upon H2O2 treatment and increased by OPN pretreatment (Fig. 4).
OPN modulated apoptotic regulator markers in H2O2-treated OPCs. The expression level of pro-apoptotic genes (BAX and BID), BCL-2 and BCL-XL (anti-apoptotic genes), and P53 was checked. As indicated, H2O2 increased P53, BAX, and BID transcript levels significantly. On the other hand, transcript level of BCL-2 and BCL-XL decreased. Pretreatment with OPN modulate expression levels of these genes when samples were treated with H2O2. In this experiment 12.5 ng/mL of OPN was implemented. The concentration of H2O2 was 3200 µM. Star indicates significant difference between samples at p < 0.05
Expression Analysis of αv, β1-, β3-, β5-, and β8- Integrins During Differentiation of hESCs to OPCs and OLs
RT-qPCR was performed to evaluate transcript levels of OPN interacting integrins in hESCs, OPCs, and OLs. Data revealed that expression levels of αv have no significant change in hESCs vs OPCs, but up-regulated considerably in OLs. Expression pattern analysis of β1 and β8 showed an increasing trend during differentiation of hESCs into OPCs and OLs, while β3 has significant change in hESCs vs OPCs and down-regulated considerably in OLs (Fig. 5).
Expression analysis of αv, β1-, β3-, β5-, and β8- integrins during differentiation of hESCs to OPCs and OLs. RT-qPCR was performed to evaluate transcript levels of OPN interacting integrins in hESCs, OPCs, and OLs. Data revealed that expression level of αv has no significant change in hESCs versus OPCs but, it up-regulated considerably in OLs. Expression pattern analysis of β1 and β8, showed an increasing trend during differentiation of hESCs into OPCs and OLs. All relative expressions were quantified and normalized with GAPDH transcript level. Represented value bars are the mean of triplicate independent experiments ± SEM. Star indicates significant difference between samples at p < 0.05
Modulation in Transcript Levels of αv-, β1-, β3-, β5-, and β8- Integrins Upon H2O2-Induced Apoptosis in the Presence and Absence of OPN
OPN is an ECM ligand which exerts its function through interacting with integrins (Denhardt et al. 2001). To elucidate whether the expression of OPN interacting integrins (αv-, β1-, β3-, β5-, and β8) modulate with H2O2 treatment, this experiment was performed. Data indicated that OPN treatment induced expression of αv-, β1-, β3-, and β8- integrins, except β5 (Fig. 6a). Moreover, H2O2 treatment reduced the expression levels of αv-, β1, β5-, and β8-. Interestingly, this significant reduction was suppressed when cells were pretreated with OPN except for the case of β5 (Fig. 6b).
OPN increased transcript level of interacting integrins in H2O2-treated OPCs. a OPN induced mRNA level of αv-, β1-, β3-, and β8- integrins, except β5 in OPN-treated OPCs. b Moreover, H2O2 treatment reduced the expression levels of αv-, β1, β5-, and β8-, while OPN was able to reverse this reduced expression levels of αv-, β1, β8-, except β3 and β5. All relative expressions were quantified and normalized with GAPDH transcript level. Represented value bars are the mean of triplicate independent experiments ± SEM. Star indicates significant difference between samples at p < 0.05
Discussion
OPN is a secretory glycosylated phosphoprotein, involved in a number of physiological events including bone formation and remodeling, immune responses, and tumor progression. The protective function of OPN, as a negative regulator of tumorigenesis, was already investigated which acts through inactivation of caspase 8 (Kim et al. 2009). Recent studies have revealed that increasing of OPN expression contributes to anti-apoptotic effects through expression or activity of other mediators including AKT, mTOR, PTEN, and β-catenin (Zahed Panah et al. 2017; Hsu et al. 2014). Also, neuroprotective role of OPN has been well defined (Albertsson et al. 2014) as well as positive effects of OPN on survival, proliferation, migration, and neuronal differentiation of NSC (Rabenstein et al. 2015). Anti-apoptotic effect of OPN was assessed in this study on H2O2-treated OPCs. To perform this experiment, hESC-derived OPCs were yielded as previously described and characterized. Both staining (Peymani et al. 2017) and RT-qPCR confirmed the production of appropriate OPCs which were capable to differentiate into OLs as already elucidated (O’Meara et al. 2010; Milner and Ffrench-Constant 1994; Peymani et al. 2017; Pouya et al. 2011). At the next step, OPCs were used as a model cell to study the protective role of OPN against H2O2-induced apoptotic condition. In order to examine this hypothesis, stress oxidative was induced by treatment of the cell with H2O2. As caspases are central component of apoptosis (Dash 1994), caspase activity assay was carried out to confirm the occurrence of apoptosis. Our data indicated that caspase activation occurred upon H2O2 treatment (92%) delineating H2O2-induced apoptosis of the cell. Pro-survival role of OPN was testified in similar condition and strongly indicated the protective effect of OPN against apoptotic conditions. Furthermore, apoptosis is triggered by activation of tumor suppressor P53, a transcription factor, in response to various stress signals, including H2O2 (Polager and Ginsberg 2009). Data indicated apoptosis was mediated by the induction in P53 transcript levels. In this respect, OPN was able to reverse H2O2-induced expression level of P53. P53 regulates the expression of several apoptotic targets including Bcl-2 family genes pathways (Polager and Ginsberg 2009). Therefore, the expression of BAX and BID as were assessed and showed to be up-regulated in response to H2O2, and inversely anti-apoptotic factors as BCL-2 and BCL-XL were down-regulated. OPN reversed these outcomes. To answer how the cells regulate their fate in response to OPN pretreatment, transcript levels of several integrin subunits responsible for OPN interaction were measured. Notably, our findings showed that expression of αV β 1/β 3/β 5 and β8 integrins (in the context of OPN) elevated in response to OPN alone and treatment with H2O2 down-regulated αV β 1/β 5 and β8 integrins expression significantly. In conclusion, OPN may act via αV integrin signaling and trigger the suppression of p53-dependent apoptotic cascades. However, further experiments are required to elucidate the detailed mechanism of OPN in prevention of cytotoxicity. In the present article, we demonstrated that OPN plays a role preventing OPCs apoptosis. However, this is necessary to show that survivor OPCs are capable to differentiate in OLs. This could be a key point that should be examined in further experiments.
Abbreviations
- ANOVA:
-
One-way analysis of variance
- bFGF:
-
Basic fibroblast growth factor
- CNS:
-
Central nervous system
- EBs:
-
Embryoid bodies
- ECM:
-
Extracellular matrix
- EGF:
-
Epidermal growth factor
- FITC:
-
Fluorescein isothiocyanate
- hESC:
-
Human embryonic stem cell
- Lam:
-
Laminin
- MBP:
-
Myelin basic protein
- MS:
-
Multiple sclerosis
- OLs:
-
Oligodendrocytes
- OPCs:
-
Oligodendrocyte progenitor cells
- OPN:
-
Osteopontin
- PBS:
-
Phosphate-buffered saline
- PDGF:
-
Platelet-derived growth factor
- PLL:
-
Poly-l-laminin
- RA:
-
Retinoic acid
- RGD:
-
Arg–Gly–Asp
- ROS:
-
Reactive oxygen species
- RT-qPCR:
-
Real-time quantitative PCR
- SEM:
-
Standard error of mean
- TRITC:
-
Tetramethyl rhodamine isothiocyanate
References
Albertsson AM, Zhang X, Leavenworth J, Bi D, Nair S, Qiao L, Hagberg H, Mallard C, Cantor H, Wang X (2014) The effect of osteopontin and osteopontin-derived peptides on preterm brain injury. J Neuroinflamm 11:197
Baud O, Greene AE, Li J, Wang H, Volpe JJ, Rosenberg PA (2004) Glutathione peroxidase-catalase cooperativity is required for resistance to hydrogen peroxide by mature rat oligodendrocytes. J Neurosci 24:1531–1540
Behera R, Kumar V, Lohite K, Karnik S, Kundu GC (2009) Activation of JAK2/STAT3 signaling by osteopontin promotes tumor growth in human breast cancer cells. Carcinogenesis 31:192–200
Buchet D, Baron-Van Evercooren A (2009) In search of human oligodendroglia for myelin repair. Neurosci Lett 456:112–119
Burdo TH, Wood MR, Fox HS (2007) Osteopontin prevents monocyte recirculation and apoptosis. J Leukoc Biol 81:1504–1511
Dash P (1994) Apoptosis; basic medical sciences. St.George’s University of London, London
Denhardt DT, Noda M, O’Regan AW, Pavlin D, Berman JS (2001) Osteopontin as a means to cope with environmental insults: regulation of inflammation, tissue remodeling, and cell survival. J Clin Invest 107:1055
Dhib-Jalbut S (2007) Pathogenesis of myelin/oligodendrocyte damage in multiple sclerosis. Neurology 68:S13–S21
Geissinger E, Weisser C, Fischer P, Schartl M, Wellbrock C (2002) Autocrine stimulation by osteopontin contributes to antiapoptotic signalling of melanocytes in dermal collagen. Cancer Res 62:4820–4828
Glass CK, Saijo K, Winner B, Marchetto MC, Gage FH (2010) Mechanisms underlying inflammation in neurodegeneration. Cell 140:918–934
Hirrlinger J, Resch A, Gutterer JM, Dringen R (2002) Oligodendroglial cells in culture effectively dispose of exogenous hydrogen peroxide: comparison with cultured neurones, astroglial and microglial cells. J Neuroche 82:635–644
Hsu KH, Tsai HW, Lin PW, Hsu YS, Lu PJ, Shan YS (2014) Anti-apoptotic effects of osteopontin through the up-regulation of Mcl-1 in gastrointestinal stromal tumors. World J Surg Oncol 12:189
Hur EM, Youssef S, Haws ME, Zhang SY, Sobel RA, Steinman L (2007) Osteopontin-induced relapse and progression of autoimmune brain disease through enhanced survival of activated T cells. Nat Immunol 8:74–83
Kajabadi NS, Ghoochani A, Peymani M, Ghaedi K, Kiani-Esfahani A, Hashemi MS, Nasr-Esfahani MH, Baharvand H (2015) The synergistic enhancement of cloning efficiency in individualized human pluripotent stem cells by peroxisome proliferative-activated receptor-γ (PPARγ) activation and rho-associated kinase (ROCK) inhibition. J Biol Chem 290(43):26303–26313
Kanduc D, Mittelman A, Serpico R, Sinigaglia E, Sinha AA, Natale C, Santacroce R, Di Corcia MG, Lucchese A, Dini L, Pani P, Santacroce S, Simone S, Bucci R, Farber E (2002) Cell death: apoptosis versus necrosis. Int J Oncol 21:165–170
Kim HJ, Lee HJ, Jun JI, Oh Y, Choi SG, Kim H, Chung CW, Kim IK, Park IS, Chae HJ, Kim HR, Jung YK (2009) Intracellular cleavage of osteopontin by caspase-8 modulates hypoxia/reoxygenation cell death through p53. Proc Natl Acad Sci U S A 106(36):15326–15331
Merrill J, Pu S.-F, Khorkova O, Ji Z, Camacho F, Busch S, Wang M, Chen T, Dinerstein, R, Yao Z (2003) Osteopontin, oligodendrocytes and myelination. Google Patents
Milner R, Ffrench-Constant C (1994) A developmental analysis of oligodendroglial integrins in primary cells: changes in alpha v-associated beta subunits during differentiation. Development 120:3497–3506
Miron VE, Kuhlmann T, Antel JP (2011) Cells of the oligodendroglial lineage, myelination, and remyelination. Biochim Biophys Acta 1812:184–193
Mouzannar R, Miric SJ, Wiggins RC, Konat GW (2001) Hydrogen peroxide induces rapid digestion of oligodendrocyte chromatin into high molecular weight fragments. Neurochem Int 38:9–15
O’Meara RW, Michalski JP, Kothary R (2010) Integrin signaling in oligodendrocytes and its importance in CNS myelination. J Signal Transduct 2011:354091
Peymani M, Ghaedi K, Hashemi MS, Ghoochani A, Kiani-Esfahani A, Nasr-Esfahani MH, Baharvand H (2017) Ameliorating the effect of pioglitazone on LPS-induced inflammation of human oligodendrocyte progenitor cells. Cell Mol Neurobiol. https://doi.org/10.1007/s10571-017-0500-6
Polager S, Ginsberg D (2009) p53 and E2f: partners in life and death. Nat Rev Cancer 9:738–748
Pouya A, Satarian L, Kiani S, Javan M, Baharvand H (2011) Human induced pluripotent stem cells differentiation into oligodendrocyte progenitors and transplantation in a rat model of optic chiasm demyelination. PLoS ONE 6:e27925
Rabenstein M, Hucklenbroich J, Willuweit A, Ladwig A, Fink GR, Schroeter M, Langen KJ, Rueger MA (2015) Osteopontin mediates survival, proliferation and migration of neural stem cells through the chemokine receptor CXCR4. Stem Cell Res Ther 6:99
Scatena M, Almeida M, Chaisson ML, Fausto N, Nicosia RF, Giachelli CM (1998) NF-κB mediates αvβ3 integrin-induced endothelial cell survival. J Cell Biol 141:1083–1093
Schordan S, Schordan E, Endlich K, Endlich N (2011) α V-Integrins mediate the mechanoprotective action of osteopontin in podocytes. Am J Physiol Renal Physiol 300:F119–F132
Selvaraju R, Bernasconi L, Losberger C, Graber P, Kadi L, Avellana-Adalid V, Picard-Riera N, Baron Van Evercooren A, Cirillo R, Kosco-Vilbois M, Feger G, Papoian R, Boschert U (2004) Osteopontin is upregulated during in vivo demyelination and remyelination and enhances myelin formation in vitro. Mol Cell Neurosci 25:707–721
Sodek J, Ganss B, McKee MD (2000) Osteopontin. Crit Rev Oral Biol Med 11:279–303
Song G, Ming Y, Mao Y, Bao S, Ouyang G (2008) Osteopontin prevents curcumin-induced apoptosis and promotes survival through Akt activation via αvβ3 integrins in human gastric cancer cells. Exp Biol Med 233:1537–1545
Sorokin L (2010) The impact of the extracellular matrix on inflammation. Nat Rev Immunol 10:712–723
Stangel M, Hartung HP (2002) Remyelinating strategies for the treatment of multiple sclerosis. Prog Neurobio 68:361–376
Watzlawik J, Warrington AE, Rodriguez M (2010) Importance of oligodendrocyte protection, BBB breakdown and inflammation for remyelination. Expert Rev Neurother 10:441–457
Wittmann C, Chockley P, Singh SK, Pase L, Lieschke GJ, Grabher C (2012) Hydrogen peroxide in inflammation: messenger, guide, and assassin. Adv Hematol 2012:541471
Zahed Panah M, Nikbakht M, Sajjadi SM, Rostami S, Norooznezhad AH, Kamranzadeh Fumani H, Ghavamzadeh A, Mohammadi S (2017) Anti-apoptotic effects of osteopontin via the up-regulation of AKT/mTOR/β-catenin loop in acute myeloid leukemia cells. Int J Hematol Oncol Stem Cell Res 11(2):148–157
Zhao C, Fancy SP, French-Constant C, Franklin RJ (2008) Osteopontin is extensively expressed by macrophages following CNS demyelination but has a redundant role in remyelination. Neurobiol Dis 31:209–217
Zhao C, Fancy SP, Franklin RJ, French-Constant C (2009) Up-regulation of oligodendrocyte precursor cell αV integrin and its extracellular ligands during central nervous system remyelination. J Neurosci Res 87:3447–3455
Acknowledgements
We thank our colleagues for their association and helpful discussions in this study.
Author information
Authors and Affiliations
Contributions
NM was involved in concept and design, acquisition, analysis and interpretation of data, and drafting of the manuscript. MP was involved in supervision, and contributed to the design of work, analysis, and interpretation of data, and drafted sections of manuscript. HG was involved in supervision and contributed to design of work. KG was involved in supervision, and contributed to the design of work, analysis, and interpretation of data, critical revision of the manuscript for important intellectual content, and finalized the manuscript. AG contributed to design the work. AKE was involved in acquisition of data and drafted sections of manuscript. MHNE was involved in supervision, and contributed to the design of work, administrative, technical, or material support.
Corresponding authors
Ethics declarations
Conflict of interest
The authors declare that they have no conflict of interest.
Rights and permissions
About this article
Cite this article
Mazaheri, N., Peymani, M., Galehdari, H. et al. Ameliorating Effect of Osteopontin on H2O2-Induced Apoptosis of Human Oligodendrocyte Progenitor Cells. Cell Mol Neurobiol 38, 891–899 (2018). https://doi.org/10.1007/s10571-017-0563-4
Received:
Accepted:
Published:
Issue Date:
DOI: https://doi.org/10.1007/s10571-017-0563-4