The chloroform-insoluble part of chemoorganoheterotrophic aerobic bacteria Arthrobacter sp. RV and Pseudomonas aeruginosa RM is subjected to thermolysis and catalytic thermolysis (aluminosilicate as catalyst). The thermolysis and catalytic thermolysis products are analyzed by chromatomass spectroscopy. It is noticed for the first time that the products of thermolysis of the insoluble part contain hydrocarbons with typical ions of adamantanes (m/z 135, 136, 149, 163) and diamantanes (m/z 187, 188, 201). It is shown by isomerization over aluminum bromide that these hydrocarbons are protoadamantanes and protodiamantanes, respectively. Unlike thermolysis, catalytic thermolysis of the insoluble part of the bacteria generates simultaneously protoadamantanes and adamantanes of the C10–C13 composition and protodiamantanes and diamantanes of the C14–C16 composition. It is suggested that one of the routes of formation of hydrocarbons having diamond-like (adamantine) structure in oils could be catalytic transformation of the bacterial biomass.
Similar content being viewed by others
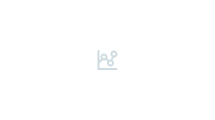
Avoid common mistakes on your manuscript.
Diamond-like hydrocarbons (HC) (adamantanes, diamantanes, trimantanes, etc.) occur in oils of various ages and origins and different degrees of transformation that are generated in clayey and carbonate strata [1–6]. Diamantanes are also found in the organic matter (OM) of the crystalline basement [7]. We showed earlier that HC of the adamantane and diamantane series were generated by thermal cracking and transformations on an acidic catalyst of high-molecular-weight (boiling above 350°C) paraffin-naphthene fractions of various types of oil and also by thermal cracking of oil resins and asphaltenes [2, 8–10]. Furthermore, these HC were formed by high-temperature cracking of high-molecular-weight n-alkanes [11]. However, the only thing that is now known about the genesis of diamond-like HC is that they are absent in the initial (biosynthesized) matter.
According to the sedimentation-migration hypothesis of oil formation, the HC were considered to arise from remains of living organisms that inhabited the earth during past geological eras. Most attention was paid to eukaryotic organisms (animals, plants, fungi). As a rule, prokaryotes (bacteria and archaea) were assigned the role of transforming the eukaryote OM. Nevertheless, formation of HC (alkanes, alkenes, alkadienes, isoprenoids, arenes) by both eukaryotes and prokaryotes was reported [12–14]. However, the role of prokaryotes in HC formation with respect to oil genesis has drawn little attention, in contrast with eukaryotes. Moreover, we showed [15–17] that relatively low-molecular-weight n-alkanes with an odd number of C atoms (C7, C9, C11, C13, C15, C17, C19, C21) and the corresponding unsaturated fatty n-acids with an even number of C atoms (C8, C10, C12, C14, C16, C18) were primarily found in the soluble part of the native biomass of bacteria Ar throbacter sp. RV and Pseudomonas aeruginosa RM. Homologs with an even number of C atoms (C22, C24, C30, C32, C34) dominated the higher-molecular-weight n-alkanes. Both strains were found to synthesize the unsaturated irregular isoprenane squalene (2,6,10,15,19,23-hexamethyltetracosa-2,6,10,14,18,22-hexaene.
Thermolysis and catalytic thermolysis of the initial OM is currently of most interest to the sedimentation-migration theory of oil generation. It was found that various classes of HC were produced at different rates during OM ripening by kerogen. Thermolysis and catalytic thermolysis of kerogen generated the same HC that were found in oil [2]. Kerogen (insoluble OM) is known to be a complicated geobiopolymer. Therefore, it can be assumed that it could also contain the insoluble part of the bacterial biomass, in particular, of Arthrobacter sp. RV and P. aeruginosa RM.
Therefore, it seemed interesting to determine if diamond-like HC are formed by thermolysis and catalytic thermolysis of the insoluble part (debris) of prokaryotes. The present study is focused on this question.
We studied the chemoorganoheterotrophic aerobic bacteria Arthrobacter sp. RV and P. aeruginosa RM, which are capable of aerobic and anaerobic growth during denitrification. Figure 1 shows the scheme that was used to study HC in the thermolysis and catalytic thermolysis products of the bacterial biomass.
The HC-oxidizing strains Arthrobacter sp. RV and P. aeruginosa RM were cultivated in liquids. The latter was grown in mineral-organic medium containing (g/L) NaNO3 (2), KH2PO4 (1), MgSO4·7H2O (0.25), CaCl2·2H2O (0.01), yeast extract (2), glucose (20), and distilled H2O at pH 7 [18]. The former was grown in “rich” liquid medium containing (g/L) peptone (2), yeast extract (1), casein hydrolysate (1), glucose (1), chalk (2), glycerin (10 mL/L), and tap water at pH 6.7-7.2 [19]. The strains were cultivated in flasks on a rocker at 28°C for 24 h (P. aeruginosa RM) and 96 h (Arthrobacter sp. RV). Biomass of the entrifugation (6000rpm).The biomass was lyophilized at 25°C and 1·10–3 kPa for 24 h.
The lyophilized bacterial biomass was extracted by CHCl3 in an ultrasonic (US) bath for 16-18 h at room temperature. The CHCl3 was a good solvent for polar and non-polar components. The CHCl3 was purified beforehand by fractional distillation.
Bacterial biomass that was insoluble in CHCl3 underwent thermolysis and catalytic thermolysis at 340°C and 280°C, respectively. Activated (450°C, 4 h) aluminosilicate was used as the catalyst. It was selected because catalysis in nature occurs in clayey strata and clay is a natural aluminosilicate. The soluble parts of the thermolysis and catalytic thermolysis products were isomerized by AlBr3, which is an active acidic catalyst and can isomerize protoadamantanes into adamantanes. The products were analyzed by capillary GC and GCMS. Diamond-like HC received the most attention.
GCMS was carried out on an Agilent 6890N/5975C instrument. HC were separated over an HP-1MS capillary column (25 m × 0.25 mm × 0.5 μm). The temperature was programmed from 70 to 290°C at 4°C/min. The carrier gas was He. All mass spectra were obtained at ionization energy 70 eV. The ionization chamber temperature was 250°C. Compounds were identified by adding presumed standards to the studied samples and by using the NIST mass-spectra library.
Thermolysis and catalytic thermolysis of the insoluble parts of the biomass of both strains formed HC with characteristic C10–C13 adamantane ions with m/z 135, 136, 149, and 163 and C14–C16 diamantane ions with m/z 187, 188, and 201 (Fig. 2, a and 3, a). As shown earlier, the situation was similar for adamantanes, diamantanes, trimantanes, and tetramantanes during a study of oil primarily of marine genesis (e.g., oil of the Salym deposit in the Bazhenov oil field) [2, 4, 5, 20]. These studies established that side peaks in the GCMS with m/z 135, 136, 149, and 163 and with m/z 187, 188, and 201 belonged to protoadamantane and protodiamantane HC, respectively.
GCMS of C10-C13 protoadamantanes and adamantanes in thermolysis (a) and catalytic thermolysis (b) products of the insoluble part of Arthrobacter sp. RV biomass and in isomerization products of the thermolysis (c) and catalytic thermolysis (d) products. Numbers denote: adamantane (1), 1-methyladamantane (2), 2-methyladamantane (3),1-ethyladamantane (4), 2-ethyladamantane (5), 1,3-dimethyladamantane (6), cis- and trans-1,4-dimethyladamantanes (7 and 8), 1,2-dimethyladamantane (9), 1-ethyl-3-methyladamantane (10), 1,3,5-trimethyladamantane (11), 1,3,6-trimethyladamantane (12), cis- and trans-1,3,4-trimethyladamantanes (13 and 14).
GCMS of C14-C16 protodiamantanes and diamantanes in thermolysis (a) and catalytic thermolysis (b) products of the insoluble part of Arthrobacter sp. RV biomass and in isomerization products of the thermolysis (c) and catalytic thermolysis (d) products. Numbers denote: diamantane (1), 4-methyldiamantane (2), 1-methyldiamantane (3), 3-methyldiamantane (4), 4,9-dimethyldiamantane (5), 1,4- and 2,4-dimethyldiamantanes (6), 4,8-dimethyldiamantane (7), 3,4-dimethyldiamantane (8).
An analogous scenario was also observed in our instance. We isomerized the thermolysis and catalytic thermolysis products over AlBr3 in order to confirm that the side peaks in the GCMS with characteristic adamantane ions were protoadamantanes; diamantanes, protodiamantanes. Many peaks with ions characteristic of C10–C13 adamantanes (m/z 135, 136, 149, 163) and C14–C16 diamantanes (m/z 187, 188, 201), the retention times of which did not agree with those of adamantanes and diamantanes, were visible in the GCMS of the thermolysis products (Fig. 2, a and 3, a). However, Fig. 2, b and 3, b (catalytic thermolysis products) showed that peaks of adamantanes and diamantanes (shown as numbers over peaks) were observed in addition to the aforementioned peaks. GCMS of the isomerization products showed that all HC isomerized into thermodynamically more stable adamantanes and diamantanes (Fig. 2, c; 2, d and 3, c; 3, d contain only peaks of adamantanes and diamantanes, respectively). Thus, it was concluded that many peaks in GCMS with the same characteristic ions as adamantanes and diamantanes belonged to protoadamantanes and protodiamantanes, like the oils. We obtained similar results for thermolysis and catalytic thermolysis of the insoluble part of P. aeruginosa RM biomass.
The equilibrium concentrations of thermodynamically more stable C11–C13 adamantane isomers are known to be similar (92.5-98%) [21, 22]. However, it was shown earlier that the relative concentrations of thermodynamically more stable adamantane isomers were far from the equilibrium values in oils and decreased with increasing molecular weight [2].
An analogous pattern was observed in our instance. Table 1 presents the distribution of adamantanes in the thermolysis and isomerization products. It can be seen that adamantanes were also formed in ratios close to those in oils as a result of catalytic thermolysis of the insoluble part of the bacterial biomass. Thus, the relative content of 1-methyladamantane was 59.3 and 71.4% for Arthrobacter sp. RV and P. aeruginosa RM, respectively; the relative content of 1,3-dimethyladamantane – 22.2 and 22%; of 1,3,5-trimethyladamantane – 11 and 8.6%.
As expected, the relative distribution of C11–C13 adamantanes in the isomerization products was close to the equilibrium value (Table 1). However, the relative concentrations of the more stable C11–C13 methyladamantane isomers also decreased with increasing molecular weight. Apparently, this was due to the fact that di- and trimethyl-substituted adamantanes reached the equilibrium concentrations more difficultly than the mono-methyl derivatives.
Table 2 compares the characteristics of the thermolysis and catalytic thermolysis products of the insoluble parts of both strains for C10–C13 adamantanes. It can be seen that the adamantane ratios were similar in both instances. Thus, the C11/C13 and C12/C13 ratios in the catalytic thermolysis products were 0.23 and 0.89 for Arthrobacter sp. RV and 0.11 and 0.75 for P. aeruginosa RM. These ratios in the isomerization products were 0.03 and 0.36-0.38 and 0.02-0.04 and 0.33-0.38, respectively.
It is noteworthy that the relative content of adamantane itself (C10) and monomethyladamantanes (C11) in both the catalytic thermolysis and isomerization products were significantly less than the relative content of di- and trisubstituted adamantanes (Table 2).
As shown above, thermolysis and catalytic thermolysis of the insoluble part of biomass from both Arthrobacter sp. RV and P. aeruginosa RM formed protoadamantanes and adamantanes in addition to C14–C16 protodiamantanes and diamantanes (characteristic ions with m/z 187, 188, 201) (Fig. 3 a and 3 b). However, Fig. 3 shows that the relative content of the C14–C16 diamantanes (with the exception of the isomerization products, Fig. 3, c and 3, d) was difficult to calculate because of the presence of a large quantity of protodiamantane HC.
Thus, the ability to form C10–C13 protoadamantanes, protodiamantanes, adamantanes and C14–C16 diamantanes via thermolysis and catalytic thermolysis of the insoluble parts of the chemoorganoheterotrophic aerobic bacteria Arthrobacter sp. RV and P. aeruginosa RM was demonstrated for the first time. It was found that the relative distribution of protoadamantanes, protodiamantanes, adamantanes, and diamantanes was analogous to that in marine oils. It could be assumed that an important formation pathway for diamond-like HC in oils could be catalytic transformation of bacterial biomass.
References
G. N. Gordadze and O. A. Aref’ev, Neftekhimiya, 37, No. 5, 387-395 (1997).
G. N. Gordadze, Thermolysis of Organic Compounds in Oil-and-Gas Exploration Geochemistry [in Russian], IGiRGI, Moscow (2002), 336 pp.
G. N. Gordadze, Neftekhimiya, 48, No. 4, 243-255 (2008).
M. V. Giruts, Ch. M. Badmaev, O. G. Erdnieva, et al., Neftekhimiya, 52, No. 2, 83-85 (2012).
M. V. Giruts, N. B. Derbetova, O. G. Erdnieva, et al., Neftekhimiya, 53, No. 5, 323-326 (2013).
V. A. Kashirtsev, I. I. Nesterov, V. N. Melenevskii, et al., Geol. Geofiz., 54, No. 8, 1227-1235 (2013).
G. N. Gordadze and G. V. Rusinova, Geokhimiya, No. 11, 1228-1232 (2004).
M. V. Giruts and G. N. Gordadze, Neftekhimiya, 47, No. 1, 11-22 (2007).
M. V. Giruts, G. V. Rusinova, and G. N. Gordadze, Neftekhimiya, 45, No. 3, 163-177 (2005).
M. V. Giruts, G. V. Rusinova, and G. N. Gordadze, Neftekhimiya, 46, No. 4, 251-261 (2006).
G. N. Gordadze and M. V. Giruts, Neftekhimiya, 48, No. 6, 412-517 (2008).
E. G. Dedyukhina, N. Ladygina, and M. B. Vainshtein, Process Biochem., 41, No. 5, 1001-1014 (2006).
M. Blumenberg, B. Oppermann, R. Guyoneaud, et al., FEMS Microbiol. Lett., 293, 73-78 (2009).
M. Rohmer, P. Bouvier, and G. Ourisson, Eur. J. Biochem., 112, 557-560 (1980).
A. R. Stroeva, M. V. Giruts, V. N. Koshelev, et al., Neftekhimiya, 53, No. 5, 1-4 (2013).
G. N. Gordadze and A. R. Stroeva, J. Pet. Environ. Biotechnol., 4, No. 6, 94 (2013).
I. V. Botvinko, O. V. Popova, A. R. Stroeva, et al., Mikrobiologiya, 83, No. 2, 152-159 (2013).
T. N. Nazina, D. Sh. Sokolova, A. A. Griogor’yan, et al., Mikrobiologiya, 72, 206-211 (2003).
T. G. Dobrovol’skaya, I. N. Skvortsova, and L. V. Lysak, Methods for Determining and Identifying Soil Bacteria [in Russian], MGU, Moscow (1990), 72 pp.
G. N. Gordadze, I. A. Matveeva, M. N. Zabrodina, et al., Neftekhimiya, No. 1, 42-50 (1998).
A. A. Petrov, Chemistry of Naphthenes [in Russian], Nauka, Moscow (1971), 388 pp.
E. I. Bagrii, Adamantanes [in Russian], Nauka, Moscow (1989), 264 pp.
Acknowledgments
The work was supported financially by the Russian Ministry of Education and Science under the auspices of the base part of state project No. 2470, “Studies of the products of mild thermolysis, properties, and kinetic stability of various asphaltenes.”
Author information
Authors and Affiliations
Additional information
Translated from Khimiya i Tekhnologiya Topliv i Masel, No. 4, pp. 15 – 20, July – August, 2014.
Rights and permissions
About this article
Cite this article
Giruts, M.V., Gordadze, G.N., Stroeva, A.R. et al. Generation of Hydrocarbons Having Adamantine Structure from Bacterial Biomass. Chem Technol Fuels Oils 50, 290–298 (2014). https://doi.org/10.1007/s10553-014-0526-0
Published:
Issue Date:
DOI: https://doi.org/10.1007/s10553-014-0526-0