Abstract
We have investigated here the anti-breast cancer properties of two novel curcumin analogues, EAC and PAC. Apoptosis was assessed by the annexin V/propidium iodide (PI) assay on different breast cancer and normal cells. Immunoblotting analysis determined the effects of these agents on different apoptotic and oncogenic proteins. Furthermore, flow cytometry and Elispot were utilised to investigate the effects on the cell cycle and the production of cytokines, respectively. Breast cancer tumour xenografts were developed in nude mice. Finally, 18F-radiolabeled PAC and curcumin were produced to study their bioavailability and tissue biodistribution in mice. PAC is five times more efficient than curcumin and EAC in inducing apoptosis, mainly via the internal mitochondrial route. This effect was 10-fold higher against ER-negative as compared to ER-positive cells, and ectopic expression of ERα rendered ER-negative breast cancer cells more resistant to PAC. In addition, PAC delayed the cell cycle at G2/M phase with a stronger effect on ER-negative cells. Moreover, PAC exhibited strong capacity as an immuno-inducer through reducing the secretion of the two major Th2 cytokines IL-4 and IL-10. Importantly, PAC significantly reduced tumour size, and triggered apoptosis in vivo. Furthermore, PAC inhibited survivin, NF-kB and its downstream effectors cyclin D1 and Bcl-2, and strongly up-regulated p21WAF1 both in vitro and in tumours. Besides, PAC exhibited higher stability in blood and greater biodistribution and bioavailability than curcumin in mice. These results indicate that PAC could constitute a powerful, yet not toxic, new chemotherapeutic agent against ER-negative breast tumours.
Similar content being viewed by others
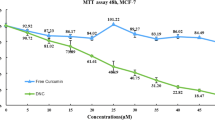
Avoid common mistakes on your manuscript.
Introduction
Breast cancer remains a major cause of morbidity and mortality worldwide [1]. Although breast cancer incidence has increased over the past four decades, mortality has remained stable, may be owing to the improvement in treatment options. However, tumour resistance and recurrence are still very frequent. Furthermore, there is relative lack of effective therapies for advanced-stage disease. This obligates scientists to keep seeking for new agents with higher efficiency and less side effects. Several dietary constituents exhibit anti-cancer properties without considerable adverse effects [2]. Curcumin (diferuloylmethane) is extracted from the root of Curcuma longa and has been widely used as a therapeutic agent for various illnesses including, inflammation, diabetes, Alzheimer, Arthritis and others [3]. Importantly, curcumin has been demonstrated to induce anti-carcinogenic effect both in vivo and in vitro [4]. This safe natural product mediates its effects through the modulation of several molecular targets including tumour suppressor proteins (p53, p21WAF1), oncoproteins (C-Myc, cyclin D1), transcription factors (NF-κB, β-catenin) and anti-apoptosis proteins (survivin, Bcl-2) [3]. Furthermore, it has been recently shown that curcumin induces immuno-restoration in tumour-bearing animals [5], indicating that the inclusion of this natural product in therapeutic regimens against cancer should be beneficial for great proportion of cancer patients, including those afflicted with breast carcinoma. Indeed, several publications have reported the anti-carcinogenic activity of curcumin against various breast cancer cell lines, including the multidrug-resistant ones [6–8]. Furthermore, numerous in vivo studies have shown its chemo-preventive effect against breast cancer [9]. It has been also shown that curcumin inhibits lung metastasis of human breast cancer in nude mice [10].
On the other hand, curcumin is limited in its clinical efficacy owing to its poor aqueous solubility and its low absorption in the gastrointestinal tract [11]. To circumvent this limitation, several approaches were carried out, including the synthesis of curcumin analogues [4, 12, 13]. Curcumin derivatives were synthesised in order to overcome the pharmacokinetics limitations, with the hope to preserve the same safety profile. Some of those analogues were more active than curcumin, whereas others were less active or exhibited similar activity [4]. CDF and EF24 are among the most promising anti-cancer curcumin analogues [14–17].
Youssef et al. have also synthesised different curcumin analogues including 1,7-Bis (4-hydroxy-3-ethoxyphenyl)-1,6-heptadien-3,5-dione (EAC) and 5-Bis (4-hydroxy-3-methoxybenzylidene)-N-methyl-4-piperidone (PAC) [18] that we have characterised in the present study.
Methods
Cell lines, chemicals and cell culture
MCF-10A, MDA-MB231, MCF-7 and T-47D were obtained from ATCC and were cultured following the instructions of the company. MDA-MB231 harbouring the control pIRESneo3 plasmid or the plasmid expressing the ERα gene were cultured as previously described [19]. BEC114 is a primary breast cancer cell culture that was grown in the same conditions.
HPLC purified curcumin and analogues, whose chemical structures are depicted in Fig. 1a were dissolved in DMSO.
PAC induces apoptosis more efficiently and specifically than curcumin and EAC. a Chemical structures of curcumin and its two analogues EAC and PAC. b Cells were treated with the indicated concentrations of curcumin, EAC, or PAC for 72 h and then cell death was assessed by annexin V/PI in association with flow cytometry. c Cells were challenged with 10 μM of each compound for 72 h and then cell death was assessed as in (b). d Histograms presenting the proportions of induced apoptosis and necrosis
Cellular lysate preparation and immunoblotting
SDS-PAGE was performed using 12% separating minigels. Equal amounts of protein from different samples were placed in boiling water for 10 min in the presence of SDS gel sample buffer (0.5 M Tris pH 6.8, 10% glycerol, 10% SDS, 5% 2-mercaptoethanol, 1% bromophenol) and electrophoresed for 2 h at 125 V. After transfer onto polyvinylidene difluoride membrane (PVDF), the membrane was first blocked with 5% powdered skimmed milk in PBST (138 mM NaCl, 2.7 mM KCl pH 7.4, 0.1% Tween) for 2 h and then incubated with the appropriate first antibody overnight. Visualisation of the second antibody was performed using a chemiluminescence detection procedure according to the manufacturer’s protocol (Amersham Pharmacia Biotech). The antibodies used: NF-κB (F-6), β-Catenin (9F2), Cyclin D1 (HD11), Survivin (C-19), Bcl-2 (100), Bax (H-9), pro-caspase3 (H-277), pro-caspase9 (F-7), p21WAF1 (F-5), GAPDH (FL-355), PCNA (PC-10) and β-actin (C-11) were purchased from Santa Cruz (USA); cleaved caspase-3 (ASP175) and cleaved caspase-9 (ASP315), Cell signalling; cleaved PARP (SP214), BD.
Quantification of protein expression level
The expression levels of proteins were measured using the densitometer (BIO-RAD GS-800 Calibrated Densitometer, USA). Films were scanned and the signal intensity of each band was determined. Next, dividing the obtained value of each band by the values of the corresponding internal control allowed the correction of the loading differences. The fold of change was determined by dividing the corrected values that corresponded to the treated samples by that of the non-treated one (time 0).
Annexin V and flow cytometry
Cells were either treated with DMSO or challenged with curcumin and its analogues. Detached and adherent cells were then harvested after 72 h, centrifuged and resuspended in 1 ml phosphate buffered saline (PBS). Cells were then stained with PI and Alexa Flour 488 annexin V. Annexin V staining was performed using Vybrant Apoptosis Assay kit # 2 (Molecular probe) following the manufacturer’s recommendations. Annexin V-stained cells were analysed by flow cytometry, measuring the fluorescence emission at 530 and >575 nm. The percentage of cells was determined by the FACScadibur apparatus and the Cell Quest Pro.
Cell cycle analysis by flow cytometry
This has been performed as previously described [20].
Tumour xenografts
Breast cancer xenografts were created in 15 nude mice by subcutaneous injection of the MDA-MB231 cells (5·106) into the right leg of each mouse. After the growth of the tumours the animals were randomised into three groups to receive intraperitonial injections of curcumin and PAC (100 mg/kg/75 μl in DMSO) or the same volume of DMSO daily for 2 weeks. Tumour size was measured with a calliper using the following formula (Length × Width × Height).
PBMC preparation and culture
Heparinised blood samples were obtained from healthy volunteers and then peripheral blood mononuclear cells (PBMCs) were obtained by centrifugation over ficol-hypaque gradients (Pharmacia, Uppsala, Sweden). 106 cells were cultured in RPMI-1640 medium supplemented with 10% FCS (Gibco, Island NY, PBS) and complements and incubated at 37°C in 5% CO2 incubator.
PBMC cytotoxicity
PBMCs were treated with different concentrations of curcumin and PAC for 6 h and cell death was measured with annexin V/PI-flow cytometry.
Elispot assay
Elispot assay was used as recommended by the manufacturer (Diaclone Research, France). 5·103 cells suspended in 100 μl complete media were inoculated in 96-well microtiter plates pre-coated with the appropriate antibody. Subsequently, cells were either treated with PHA (10 μg/ml) or LPS (1 μg/ml), for stimulating the production of IFN-γ, IL-4 and IL-10 or TNF-α and IL-6, respectively. The plates were incubated at 37°C in humidified atmosphere containing 5% CO2 for appropriate period of time according to the different cytokine kinetics. Afterwards, cells were either sham-treated or challenged with different concentrations of PAC and curcumin and re-incubated for 24 h (IFN-γ, TNF-α and IL-6) or 48 h (IL-4 and IL-10) in the same conditions. After washing, the plates were red using the Elispot AID reader version 3.0.
Radiosynthesis of 18F-PAC and 18F-curcumin
Fluorine-18 was produced by the 18O (p, n) 18F reaction on enriched water using 12 MeV RDS cyclotron. The reaction mixture was then purified by HPLC using C18 column (10 μm, 4.6 × 250 mm) with a gradient of 0.1% TFA in water (solvent A) and 0.1% TFA in CH3CN (solvent B) at a flow rate of 1 ml/min.
Plasma and tissue distribution of PAC and curcumin
Sixteen mice (Balb-c, 25–30 g) were randomised into two groups (n = 8) and then were injected via the tail vein with 100 μl of 18F-PAC or 18F-curcumin (20 μCi) formulated in PBS buffer. Animals were killed at 5 and 60 min post-injection (four animals for each time point) and samples of blood and organs of interest were dissected, weighed and assayed for radioactivity. The percentage of the injected dose per gram was then calculated for all tissues using a stored sample of the injection solution to estimate the total dose injected per mouse.
Partition coefficient
100 μl of the HPLC-purified 18F-PAC and 18F-curcumin was added 1 ml of n-octanol and water. After partial separation of the phases by gravity, 0.7 ml of each phase was transferred to separate tubes and centrifuged at 5000×g for 5 min. Duplicate 0.2 ml aliquots of each phase was taken for γ-radioactivity measurement and the partition coefficient was then determined by the function: Partition coefficient = Log10 (counts in octanol layer/counts in aqueous layer).
Water solubility
Saturated solutions were obtained by adding excess amounts of curcumin and PAC in water. These solutions were left for 1 week in a thermostat at room temperature. Samples of saturated solutions were withdrawn in triplicates, filtered and placed in different crucibles (previously dried and weighed). Water in each sample was evaporated by gentle heating until constant weigh and solubilities were calculated in mmol dm−3.
Results
PAC triggers apoptosis more effectively and more specifically than curcumin
It is well known that curcumin triggers apoptosis in various types of cancer cells [21]. Therefore, we sought to determine the efficiency of EAC and PAC in triggering cell death in breast cancer cells. To this end, sub-confluent normal as well as breast carcinoma cells were treated either with DMSO or challenged with different concentrations of each compound. Cells were re-incubated for 72 h and then harvested and stained with annexin V and PI and then analysed by flow cytometry. The real effect of each drug was obtained by discarding the proportion of dead cells obtained in the control samples from the treated ones for each cell line and each agent. The proportion of apoptotic cells was considered as the sum of both early and late apoptosis. Like curcumin, EAC and PAC triggered mainly apoptosis in all cell lines (Fig. 1c). Treatment of MCF-10A and MDA-MB231 cells with increasing doses of the three compounds showed dose-dependent increase in MCF-10A cell death reaching 30, 20 and 40% apoptosis in response to 40 μM of curcumin, EAC and PAC, respectively. Interestingly, the breast cancer MDA-MB231 cells were more sensitive to the three compounds with higher sensitivity to PAC (Fig. 1b). Interestingly, PAC was highly effective at 10 and 20 μM inducing apoptosis in about 60% of cells, while the effect of curcumin and EAC was only marginal at this concentration. By contrast the effect of the three compounds was similar at 40 μM, triggering apoptosis in about 55% of cells (Fig. 1b). Therefore, 10 μM was used for the rest of the study. At this concentration, PAC triggered apoptosis in 55% of the highly invasive MDA-MB231 cells, but only 10% of these cells died through necrosis (Fig. 1c, d). Likewise, when the primary breast cancer cells (BEC114) was treated similarly, 75% of cells died through apoptosis (Fig. 1d). Similar results were obtained with another primary cell culture (data not shown). On the other hand, PAC triggered only about 12% of apoptosis and 5% necrosis in the “normal” cell line MCF-10A (Fig. 1c, d). This shows that PAC induces apoptosis specifically in breast cancer cells. However, using the same concentration, both curcumin and EAC did not induce apoptosis in all these cell lines (Fig. 1c, d).
The proapoptotic effect of PAC depends on the ER status of breast cancer cells
By contrast to the ER− breast cancer cells MDA-MB231 and BEC114, the ER+ breast cancer cells MCF-7 and T-47D, showed resistance to the three compounds at 10 μM (Fig. 2a). However, the proportion of apoptotic cells increased by increasing the concentration of curcumin and the analogues. Indeed, using 40 μM, the proportion of PAC-induced cell death reached 35 and 70% in MCF-7 and T-47D cells, respectively. However, curcumin and EAC triggered apoptosis in <20% of these cells (Fig. 2a). These results confirm that PAC is more efficient than curcumin and EAC in triggering apoptosis and raise an important question regarding the potential role of the estrogen receptor status in the efficiency of PAC in inducing apoptosis. To test this hypothesis, we made use of MDA-MB231 cells transfected with a plasmid harbouring the ERα gene or an empty vector (control) [19]. Figure 2b shows that cells that express the ERα gene are more resistant to PAC than control cells. Indeed, in response to 5 μM of PAC about 50% of ER+ cells underwent apoptosis, but only 25% of ER− cells died through apoptosis. Likewise, ERα-expressing cells were more resistant than ER-negative cells to 10 μM of PAC. This shows that PAC-dependent induction of apoptosis is indeed modulated by the ER status of the cells.
PAC triggers apoptosis more efficiently in ER-negative cells. a Cells were challenged with the indicated concentrations of each compound and then apoptosis was assessed like in Fig. 1b. b MDA-MB231 cells expressing either control vector (ER−) or ER-expressing vector (ER+) were treated with PAC for 72 h and then apoptosis was assessed by annexin V/PI like in Fig. 1b. The error bars represent the standard deviations of three experiments
PAC triggers apoptosis through the mitochondrial pathway
To further elucidate the role of PAC in inducing apoptosis, MDA-MB231 cells were treated with 10 μM and harvested after different periods of time (0–72 h). Whole cell extracts were prepared and 50 μg of proteins were used to analyse the effect of PAC on the cleavage of the important effector caspase 3 (procaspase-3) by immunoblotting. Figure 3a shows that PAC treatment caused a time-dependent decrease in the level of procaspase 3, reaching at 72 h a level 5-fold lower than the basal level. Next, we evaluated the levels of the pro- and anti-apoptotic proteins (Bax and Bcl-2). While the level of Bax decreased only slightly (about 2-fold), PAC triggered a time-dependent decrease in the level of Bcl-2, reaching at 72 h a level 25-fold lower than the basal level (Fig. 3a). Therefore, PAC elicited a time-dependent increase in the Bax/Bcl-2 ratio, reaching its maximum (12-fold higher) after 72 h of treatment. This shows that PAC induces apoptosis mainly through the mitochondrial pathway via Bcl-2 decrease. To confirm this, we assessed the effect of PAC on the level of procaspase-9. Figure 3a shows a time-dependent decrease in the level of pro-caspase 9 and the protein became undetectable after 48 h.
PAC triggers apoptosis through the mitochondrial pathway and modulates the expression of several oncoproteins. a The MDA-MB231 cells were treated with 10 μM of PAC, and then were harvested after the indicated periods of time. 50 μg of extracted proteins were used for western blot analysis utilising the indicated antibodies. The numbers below the bands represent the corresponding expression levels as compared to time 0 and after normalisation against GAPDH. b MDA-MB231 cells were either sham-treated or challenged with 5, 10, 20 or 40 μM of curcumin and PAC. Subsequently, cells were collected after 24 h and 50 μg of extracted proteins were used for western blot analysis using the indicated antibodies. The numbers under the bands represent the corresponding expression levels as compared to time 0 and after normalisation against GAPDH
PAC is an efficient inhibitor of cancer promoting genes
MDA-MB231 cells were treated with increasing concentrations of PAC and curcumin, harvested after 24 h, and then protein levels were monitored by immunoblotting. Curcumin treatments had only slight effect on the NF-κB level. However, 20 and 40 μM of PAC led to 2.5- and 3.3-fold decrease in the level of NF-κB, respectively (Fig. 3b). Similarly, while the effect of curcumin on the β-catenin level was only marginal, 20 μM of PAC led to 5-fold decrease in the level of this protein. To confirm the inhibitory effect of PAC on NF-kB and β-catenin, we studied the effect of curcumin and PAC on the common downstream effector cyclin D1 [22–24]. While curcumin treatment (40 μM) decreased cyclin D1 level about 2-fold, significant decrease (3.3- and 5-fold) was obtained after treatment with 20 and 40 μM of PAC, respectively (Fig. 3b). Therefore, PAC has stronger effect than curcumin in inhibiting the cyclin D1 oncoprotein, which confirms a potent PAC-dependent inhibition of both signalling pathways NKT/NF-κB and WNT/β-catenin.
In addition, we studied the effect of curcumin and PAC on the level of survivin, a cancer anti-apoptosis marker [25]. Therefore, while treatment by 40 μM of curcumin led to 2.5-fold decrease, 20 and 40 μM of PAC decreased the level of survivin 3.3- and 5-fold, respectively (Fig. 3b). Together, these results show that PAC also inhibits several cancer-related pathways, and has stronger effect than curcumin.
PAC arrests breast cancer cells at the G2/M phase of the cell cycle
Sub-confluent cells were treated with either DMSO or 10 μM of each compound for different periods of time and DNA content was analysed by flow cytometry. PAC (10 μM) treatment of MDA-MB231 cells resulted in significant increase in the proportion of G2/M cells from 20% at time 0 to 37% at 16 h. Then, it decreased slightly but remained at higher level as it reached 30% at 36 h (Fig. 4a). Therefore, PAC caused a strong delay of the MDA-MB231 cells at the G2/M phase of cell cycle, while at the same concentration curcumin and EAC had only slight effect (Fig. 4a). The ER-positive MCF-7 cells were also delayed in G2/M in response to PAC. However, the delay was not as persistent as for MDA-MB231 cells (Fig. 4a). On the other hand, while curcumin and EAC had a slight effect on the cell cycle of the normal MCF-10A cells, PAC had no effect on these cells (data not shown). This shows that PAC is more efficient and specific than curcumin and EAC in delaying the cell cycle at G2/M phase.
PAC delays the cell cycle at G2/M and induces p21WAF1. a Sub-confluent cells were treated with 10 μM of each compound and harvested after different periods of time. DNA contents were analysed by flow cytometry. The curves show the proportions of cells in G2/M phase. b MDA-MB231 cells were treated with 10 μM of each compound for the indicated periods of time, and then cell lysates were prepared and 50 μg of proteins were used for western blot analysis utilising the indicated antibodies. c Curves indicating the level of p21WAF1 as compared to time 0 and after normalising against GAPDH. Error bars represent SDs of three different experiments
PAC strongly up-regulates p21WAF1 in breast cancer cells
MDA-MB231 cells were treated with 10 μΜ of each compound and harvested after different periods of time for western blot analysis. Figure 4b, c shows that the level of p21WAF1 increased only 2-fold by both curcumin and EAC. On the other hand, PAC triggered time-dependent up-regulation of p21WAF1, starting early at 2 h post-treatment and reached its maximum (7.4-fold) at 8 h, then decreased (Fig. 4b, c). Therefore, PAC is a strong inducer of p21WAF1 expression more efficiently than curcumin.
PAC is a potent inhibitor of Th2 cytokines IL-4 and IL-10
Next, we sought to assess the efficiency of PAC in modulating the immune system. Therefore, we first studied the cytotoxic effect of curcumin and PAC on PBMCs obtained from healthy individuals. Cells were treated with different concentrations of both agents, incubated for 6 h and then cell viability was assessed using annexin V/PI-flow cytometry. Figure 5a shows that both agents were not cytotoxic against PBMCs for up to 40 μM. Next, we investigated the effect of curcumin and PAC on the production by PBMCs of different immuno-modulator molecules that are implicated in carcinogenesis. To this end, PBMCs were treated with increasing concentrations of both curcumin and PAC, after being stimulated for the production of the cytokines. Figure 5b shows that up to 10 μM both curcumin and PAC had only marginal effect on the production of IFN-γ. However, at 40 μM the effect of PAC on the production of IFN-γ was more significant leading to 4-fold decrease as compared to non-treated cells, and 2-fold as compared to curcumin-treated cells (Fig. 5b). Similar results were obtained for TNF-α (data not shown). On the other hand, PAC had a strong effect on the production of the Th2 cytokines IL-4 and IL-10. Indeed, as compared to non-treated cells or those challenged with curcumin, the treatment with 10 μM of PAC reduced the production of IL-4 to levels 60% lower (Fig. 5c). Importantly, the effect of PAC on IL-10 production was more significant with 3.5-fold reduction in response to only 1 μM, while curcumin had no effect at this concentration (Fig. 5d). At 5 μM both curcumin and PAC reduced the production of IL-10, but the effect of PAC was 10-fold more efficient (Fig. 5d). Together, these results indicate that PAC is a potent immuno-modulator agent.
PAC is a potent immuno-modulator. a PBMCs were either sham-treated (DMSO) or challenged with the indicated concentrations of curcumin and PAC for 6 h and the cytotoxic effect was assessed with the annexin V/PI-flow cytometry assay. b–d The production of IFN-γ and IL-4 or IL-10 was stimulated with PHA or LPS, respectively, and cells were treated with the indicated concentrations of curcumin and PAC. Cytokine production was assed by Elispot using the appropriate antibodies
PAC is less lipophilic and more soluble in water than curcumin
HPLC-purified 18F-PAC and 18F-curcumin were mixed with n-octanol and water and after complete separation of the phases, γ-radioactivity was measured and the partition coefficient was determined in order to evaluate the lypophilicity of both compounds. Figure 6a shows that both exhibit lypophilic characteristic, however, 18F-curcumin is 2-fold (Log10) more lipophilic than 18F-PAC. When the solubility in water of both compounds was tested, we have found that 18F-PAC is 27-fold more soluble in water than 18F-curcumin (Fig. 6b).
Biodistribution and anti-cancer effect of curcumin and PAC in vivo
Next, we studied tissue biodistribution and stability of PAC and curcumin in mice using 18F-PAC and 18F-curcumin. 100 μl of radiotracers (20 μCi) were administered i.v. and the animals were killed after 5 and 60 min and samples of blood as well as various organs were removed, weighed and counted. Figure 7a shows that both agents were very well distributed in the body and reached different organs including the brain. The level of 18F-PAC presents in the blood after 5 min of administration was about 2-fold higher than the level of 18F-curcumin. Interestingly, at 60 min only 1% ID/g of 18F-curcumin was still present in the blood as compared to 5% ID/g of 18F-PAC (Fig. 7a). This indicates that during 1 h time 18F-PAC is 5-fold more stable than 18F-curcumin in the blood in mice. Similarly, the uptake and stability of 18F-PAC in most of the studied organs was higher than 18F-curcumin. Indeed, the liver uptake of 18F-PAC was 45% ID/g as compared to 18F-curcumin (10% ID/g) at 5 min postinjection (Fig. 7a). For the other organs the differences were more pronounced at 60 min than at 5 min post-injection. 18F-PAC and 18F-curcumin were present in the kidney at 60 min postinjection at 1.5% ID/g and 5% ID/g, respectively (Fig. 7a). Similar results were obtained for the heart (Fig. 7a). This indicates that 18F-PAC has a better bioavailability than curcumin.
Biodistribution and anti-cancer effect of curcumin and PAC in mice. a 18F-PAC and 18F-curcumin (20 μCi) were injected in Balb-c mice via the tail vein and the animals were killed at the indicated periods of time. Samples of blood and the indicated organs were collected, weighed and counted. b Breast cancer xenografts were created by injecting MDA-MB231 cells subcutaneously into nude mice. When tumours reached a reasonable volume, both products (dissolved in DMSO) were given i.p. at a dose of 100 mg/kg/day. Control animals were treated with DMSO only. The error bars represent the standard deviations. c Following the treatments tumours were excised and protein extracts were prepared and used for immunoblotting analysis using the indicated antibodies
To study the anti-cancer effect of PAC and curcumin in mice, breast cancer xenografts were created by injecting 5·106 MDA-MB231 cells subcutaneously into nude mice. When tumours reached a reasonable volume, both products (dissolved in DMSO) were given i.p. at a dose of 100 mg/kg/day for 2 weeks. Control animals were treated with DMSO only. Interestingly, in the mock-treated animals, the volume of the tumours increased in a time-dependent manner and became 3-fold bigger than the initial ones (Fig. 7b). On the other hand, treatment with PAC halved the tumours during the first 5 days of treatment, then their volume increased very slowly, but it did not doubled even after 14 days of treatment (Fig. 7b). This shows that PAC inhibits tumour growth and is more efficient than curcumin in vivo as well.
Subsequently, we investigated the effect of PAC and curcumin on the expression of various cancer-related genes in tumour xenografts. Figure 7c shows that PAC had a strong effect on various cancer-related proteins. Indeed, PAC increased the levels of the cleaved PARP and the active forms of caspase-3 and caspase-9 and decreased the level of the anti-apoptosis proteins Bcl-2 and survivin, suggesting the induction of apoptosis in vivo (Fig. 7c). Concomitantly, PAC had strong inhibitory effect on the cell proliferation marker PCNA and augmented the level of the proliferation inhibitor p21WAF1, reflecting an inhibitory effect on cell proliferation. Furthermore, treatment with PAC led to a great decrease in the level of NF-κB and its downstream effector cyclin D1 (Fig. 7c). This shows that PAC has strong effect on breast tumours in vivo.
Discussion
In the present study, we investigated the anti-breast cancer effects of two curcumin analogues (EAC and PAC). We have found that while EAC properties are very similar to curcumin, PAC exhibited stronger anti-cancer features with more potent effect on ER-negative cells. Therefore, PAC could be considered as a potential therapeutic agent for ER-negative breast tumours for the following reasons.
First, PAC triggered apoptosis in great proportion of different breast cancer cell lines as well as primary cells. This apoptosis induction is mediated through the mitochondrial pathway via Bcl-2 decrease, and is p53-independent since it occurred in the p53-defective cells, MDA-MB231 [26]. Importantly, this effect is related to the status of the oestrogen receptor. Indeed, the pro-apoptotic effect of PAC was 10-fold higher against the ER− cells (MDA-MB231, BEC114) than against the ER+ cells (MCF-7, T-47D). Furthermore, ectopic expression of the ERα gene rendered the MDA-MB231 cells more resistant than their counterpart control cells (Fig. 2). Thereby, PAC could be used to consolidate the adjuvant treatment of the clinically aggressive ER-negative breast cancer patients, whose prognosis is still poor.
Second, PAC is a potent inhibitor of cell proliferation by delaying the cell cycle at G2/M phase. This effect was also more pronounced on ER-negative breast cancer cells than ER-positive cells (Fig. 4a). Interestingly, this effect was accompanied by a great increase in the level of the cyclin-dependent kinase inhibitor p21WAF1, which could play a major role in the cell cycle arrest at G2/M. Since MDA-MB231 is a p53-defective cell line, this result suggests the ability of PAC to induce p21WAF1 through p53-independent mechanism. Overexpression of p21WAF1 can block both the G1/S and G2/M transitions of the cell cycle [27]. Furthermore, p21WAF1 is a modulator of apoptosis in a number of systems [28–30]. Therefore, the strong PAC-dependent up-regulation of p21WAF1 in a p53-independent manner could be of great value for the inhibition of cancer cells proliferation and the induction of cell death in various p53-detective breast tumours.
Third, like curcumin, PAC down-regulated several onco-proteins known to be highly expressed in breast cancer cells and tissues. However, the effect of PAC was higher on all the proteins tested, namely, NF-κB, β-catenin, cyclin D1, Bcl-2 and survivin. NF-κB up-regulation is implicated not only in tumour growth and progression, but also in the resistance to chemo- and radiotherapies. Several studies have documented the elevated activity of this protein in breast cancer cells [31, 32], which makes it an excellent target for cancer therapy [33]. β-catenin is another transcription factor that has been found highly expressed in various types of cancer, including breast carcinomas [34, 35]. Cyclin D1 is an oncogene that is over-expressed in about 50% of all breast cancer cases [36], and its down-regulation is an important target in breast cancer therapy [37]. Therefore, PAC-related down-regulation of NF-κB and β-catenin and their common downstream target cyclin D1 could have a great inhibitory effect on breast cancer growth. Furthermore, PAC had a strong inhibitory effect on the two major apoptosis inhibitor proteins Bcl-2 and survivin, which are both related to breast cancer pathology and therapeutic outcome [25, 38, 39]. Importantly, the inhibitory effect of PAC on these onco-proteins was also observed in vivo in tumour xenografts. Therefore, like curcumin, PAC seems to have multiple molecular targets.
Fourth, PAC is a strong immune-modulator. It has a great effect on the production of the Th2 cytokines IL-4 and IL-10, which have immunosuppressive and tumour growth stimulating functions. Cumulative evidence indicate that IL-4 is a key enzyme not only for Th2 type immune reactions, but also for tumour cell growth itself in various human cancers, including breast carcinomas [40]. It is believed now that IL-4 promotes cancer cell survival through up-regulation of anti-apoptosis genes. Similarly, high systemic levels of IL-10 correlated well with poor survival of patients suffering different types of cancer [41]. Therefore, down-regulation of these cytokines could boost the immune response and could also sensitise tumour cells to radio- and chemotherapeutic agents.
Fifth, the biological activities of PAC could be explained by its chemical structure. Thus, replacing the methylene group and the two carbonyl groups in curcumin by N-Methyl-4-Piperdone in PAC could increase both stability and hydrophilicity. Indeed, PAC is 27-fold more soluble in water than curcumin (Fig. 5b). This led to better pharmacokinetics and tissue bio-distribution in mice (Fig. 6a). Indeed, the level of 18F-PAC in the blood and the different other organs was 5-fold higher than that of 18F-curcumin, 1 h after the injection of the products. Similar results were previously reported for 18F-curcumin [42]. Furthermore, the higher uptake of 18F-PAC in the heart and the brain suggests that this agent could be of great value for molecular imaging in these organs.
Sixth, PAC suppressed the growth of tumour xenografts in nude mice more efficiently than curcumin, with no adverse effects. PAC triggered apoptosis and inhibited several breast cancer-related proteins, such as survivin, NF-κB and cyclin D1.
It is noteworthy that various curcumin analogues showed anti-carcinogenic effect against breast cancer cell lines in vitro and in vivo. The most effective and well-studied compound is the EF24 [9, 12, 43]. EF24 also triggers cell cycle arrest and apoptosis in MCF-7 and MDA-MB231 cells [12, 43]. Interestingly, the pro-apoptotic effect of PAC is 2-fold higher than that of EF24 against the MDA-MB231 cells [14]. Besides, PAC showed strong immuno-modulator effect and more efficiency against ER-negative than ER-positive cells.
These data show that PAC warrants further investigations for its potential use as chemotherapeutic agent against ER-negative and also p53-defective tumours.
References
Smigal C, Jemal A, Ward E, Cokkinides V, Smith R, Howe HL, Thun M (2006) Trends in breast cancer by race and ethnicity: update 2006. CA Cancer J Clin 56:168–183
Garg AK, Buchholz TA, Aggarwal BB (2005) Chemosensitization and radiosensitization of tumors by plant polyphenols. Antioxid Redox Signal 7:1630–1647
Goel A, Kunnumakkara AB, Aggarwal BB (2007) Curcumin as “Curecumin”: from kitchen to clinic. Biochem Pharmacol 19:19
Anand P, Thomas SG, Kunnumakkara AB, Sundaram C, Harikumar KB, Sung B, Tharakan ST, Misra K, Priyadarsini IK, Rajasekharan KN et al (2008) Biological activities of curcumin and its analogues (Congeners) made by man and mother nature. Biochem Pharmacol 76:1590–1611
Bhattacharyya S, Mandal D, Saha B, Sen GS, Das T, Sa G (2007) Curcumin prevents tumor-induced T cell apoptosis through Stat-5a-mediated Bcl-2 induction. J Biol Chem 282:15954–15964
Labbozzetta M, Notarbartolo M, Poma P, Maurici A, Inguglia L, Marchetti P, Rizzi M, Baruchello R, Simoni D, D’Alessandro N (2009) Curcumin as a possible lead compound against hormone-independent, multidrug-resistant breast cancer. Ann NY Acad Sci 1155:278–283
Kim HI, Huang H, Cheepala S, Huang S, Chung J (2008) Curcumin inhibition of integrin (alpha6beta4)-dependent breast cancer cell motility and invasion. Cancer Prev Res (Phila PA) 1:385–391
Moiseeva EP, Almeida GM, Jones GD, Manson MM (2007) Extended treatment with physiologic concentrations of dietary phytochemicals results in altered gene expression, reduced growth, and apoptosis of cancer cells. Mol Cancer Ther 6:3071–3079
Anand P, Sundaram C, Jhurani S, Kunnumakkara AB, Aggarwal BB (2008) Curcumin and cancer: an “old-age” disease with an “age-old” solution. Cancer Lett 267:133–164
Aggarwal BB, Shishodia S, Takada Y, Banerjee S, Newman RA, Bueso-Ramos CE, Price JE (2005) Curcumin suppresses the paclitaxel-induced nuclear factor-kappaB pathway in breast cancer cells and inhibits lung metastasis of human breast cancer in nude mice. Clin Cancer Res 11:7490–7498
Anand P, Kunnumakkara AB, Newman RA, Aggarwal BB (2007) Bioavailability of curcumin: problems and promises. Mol Pharm 4:807–818
Amolins MW, Peterson LB, Blagg BS (2009) Synthesis and evaluation of electron-rich curcumin analogues. Bioorg Med Chem 17:360–367
Simoni D, Rizzi M, Rondanin R, Baruchello R, Marchetti P, Invidiata FP, Labbozzetta M, Poma P, Carina V, Notarbartolo M et al (2008) Antitumor effects of curcumin and structurally beta-diketone modified analogs on multidrug resistant cancer cells. Bioorg Med Chem Lett 18:845–849
Adams BK, Cai J, Armstrong J, Herold M, Lu YJ, Sun A, Snyder JP, Liotta DC, Jones DP, Shoji M (2005) EF24, a novel synthetic curcumin analog, induces apoptosis in cancer cells via a redox-dependent mechanism. Anticancer Drugs 16:263–275
Padhye S, Banerjee S, Chavan D, Pandye S, Swamy KV, Ali S, Li J, Dou QP, Sarkar FH (2009) Fluorocurcumins as cyclooxygenase-2 inhibitor: molecular docking, pharmacokinetics and tissue distribution in mice. Pharm Res 26:2438–2445
Padhye S, Yang H, Jamadar A, Cui QC, Chavan D, Dominiak K, McKinney J, Banerjee S, Dou QP, Sarkar FH (2009) New difluoro Knoevenagel condensates of curcumin, their Schiff bases and copper complexes as proteasome inhibitors and apoptosis inducers in cancer cells. Pharm Res 26:1874–1880
Selvendiran K, Tong L, Vishwanath S, Bratasz A, Trigg NJ, Kutala VK, Hideg K, Kuppusamy P (2007) EF24 induces G2/M arrest and apoptosis in cisplatin-resistant human ovarian cancer cells by increasing PTEN expression. J Biol Chem 282:28609–28618
Youssef KM, El-Sherbeny MA, El-Shafie FS, Farag HA, Al-Deeb OA, Awadalla SA (2004) Synthesis of curcumin analogues as potential antioxidant, cancer chemopreventive agents. Arch Pharm (Weinheim) 337:42–54
Abukhdeir AM, Blair BG, Brenner K, Karakas B, Konishi H, Lim J, Sahasranaman V, Huang Y, Keen J, Davidson N et al (2006) Physiologic estrogen receptor alpha signaling in non-tumorigenic human mammary epithelial cells. Breast Cancer Res Treat 99:23–33
Al-Mohanna MA, Manogaran PS, Al-Mukhalafi Z, Al-Hussein AK, Aboussekhra A (2004) The tumor suppressor p16(INK4a) gene is a regulator of apoptosis induced by ultraviolet light and cisplatin. Oncogene 23:201–212
Aggarwal BB, Kumar A, Bharti AC (2003) Anticancer potential of curcumin: preclinical and clinical studies. Anticancer Res 23:363–398
Rowlands TM, Pechenkina IV, Hatsell S, Cowin P (2004) Beta-catenin and cyclin D1: connecting development to breast cancer. Cell Cycle 3:145–148
Guttridge DC, Albanese C, Reuther JY, Pestell RG, Baldwin AS Jr (1999) NF-kappaB controls cell growth and differentiation through transcriptional regulation of cyclin D1. Mol Cell Biol 19:5785–5799
Hinz M, Krappmann D, Eichten A, Heder A, Scheidereit C, Strauss M (1999) NF-kappaB function in growth control: regulation of cyclin D1 expression and G0/G1-to-S-phase transition. Mol Cell Biol 19:2690–2698
Altieri DC (2008) Survivin, cancer networks and pathway-directed drug discovery. Nat Rev Cancer 8:61–70
Lacroix M, Toillon RA, Leclercq G (2006) p53 and breast cancer, an update. Endocr Relat Cancer 13:293–325
Dotto GP (2000) p21(WAF1/Cip1): more than a break to the cell cycle? Biochim Biophys Acta 1471:M43–M56
Gartel AL, Tyner AL (2002) The role of the cyclin-dependent kinase inhibitor p21 in apoptosis. Mol Cancer Ther 1:639–649
Hickman ES, Moroni MC, Helin K (2002) The role of p53 and pRB in apoptosis and cancer. Curr Opin Genet Dev 12:60–66
Stanelle J, Putzer BM (2006) E2F1-induced apoptosis: turning killers into therapeutics. Trends Mol Med 12:177–185
Cao Y, Karin M (2003) NF-kappaB in mammary gland development and breast cancer. J Mammary Gland Biol Neoplasia 8:215–223
Haffner MC, Berlato C, Doppler W (2006) Exploiting our knowledge of NF-kappaB signaling for the treatment of mammary cancer. J Mammary Gland Biol Neoplasia 11:63–73
Van Waes C (2007) Nuclear factor-kappaB in development, prevention, and therapy of cancer. Clin Cancer Res 13:1076–1082
Paul S, Dey A (2008) Wnt signaling and cancer development: therapeutic implication. Neoplasma 55:165–176
Prasad CP, Gupta SD, Rath G, Ralhan R (2007) Wnt signaling pathway in invasive ductal carcinoma of the breast: relationship between beta-catenin, dishevelled and cyclin D1 expression. Oncology 73:112–117
Bartkova J, Lukas J, Strauss M, Bartek J (1995) Cyclin D1 oncoprotein aberrantly accumulates in malignancies of diverse histogenesis. Oncogene 10:775–778
Yang C, Trent S, Ionescu-Tiba V, Lan L, Shioda T, Sgroi D, Schmidt EV (2006) Identification of cyclin D1- and estrogen-regulated genes contributing to breast carcinogenesis and progression. Cancer Res 66:11649–11658
Tanaka K, Iwamoto S, Gon G, Nohara T, Iwamoto M, Tanigawa N (2000) Expression of survivin and its relationship to loss of apoptosis in breast carcinomas. Clin Cancer Res 6:127–134
Callagy GM, Pharoah PD, Pinder SE, Hsu FD, Nielsen TO, Ragaz J, Ellis IO, Huntsman D, Caldas C (2006) Bcl-2 is a prognostic marker in breast cancer independently of the Nottingham Prognostic Index. Clin Cancer Res 12:2468–2475
Nagai S, Toi M (2000) Interleukin-4 and breast cancer. Breast Cancer 7:181–186
Mocellin S, Marincola FM, Young HA (2005) Interleukin-10 and the immune response against cancer: a counterpoint. J Leukoc Biol 78:1043–1051
Ryu EK, Choe YS, Lee KH, Choi Y, Kim BT (2006) Curcumin and dehydrozingerone derivatives: synthesis, radiolabeling, and evaluation for beta-amyloid plaque imaging. J Med Chem 49:6111–6119
Adams BK, Ferstl EM, Davis MC, Herold M, Kurtkaya S, Camalier RF, Hollingshead MG, Kaur G, Sausville EA, Rickles FR et al (2004) Synthesis and biological evaluation of novel curcumin analogs as anti-cancer and anti-angiogenesis agents. Bioorg Med Chem 12:3871–3883
Acknowledgments
We are grateful to Ben Ho Park for providing us with the ER-expressing pIRESneo3 plasmid. We would like also to thank Dr. Ra’afat El-Sayed, Falah Al-Mohanna and Jonathan Caijas for their help with animal studies. We are also thankful to Dr. Bedri Karakas and Dr. Abde Abukhdeir for their help and fruitful discussions. This work was performed under the RAC proposal # 2050039 and was supported by King Abdelaziz city of Sciences and technology (KACST proposal # 08-MED476-20).
Conflict of interest
None.
Author information
Authors and Affiliations
Corresponding author
Rights and permissions
About this article
Cite this article
Al-Hujaily, E.M., Mohamed, A.G., Al-Sharif, I. et al. PAC, a novel curcumin analogue, has anti-breast cancer properties with higher efficiency on ER-negative cells. Breast Cancer Res Treat 128, 97–107 (2011). https://doi.org/10.1007/s10549-010-1089-3
Received:
Accepted:
Published:
Issue Date:
DOI: https://doi.org/10.1007/s10549-010-1089-3