Abstract
The structure of Cerrado vegetation (sensu stricto) along roadsides was compared with that of protected areas (reserves). The superior stratum of reserves had 2.7 times more individuals and 1.4 times more species than on roadsides, while in the intermediate stratum there were 1.7 times more individuals and 25 % more species in reserves. Additionally, roadsides had a lower prevalence of tree species with thin bark and from forest physiognomies. In the intermediate stratum of roadsides there was a lower proportion of forest species, and also fewer species dispersed by animals. These differences occur, possibly because the vegetation of their edges is cleared during the construction of the roads and part of the soil is taken as landfill. Later, they suffer from a higher fire frequency and high coverage of exotic grasses (80 % average). Moreover, it is likely that the absence of some dispersers on roadsides may affect the recruitment of young plants. Despite these differences, the roadsides are able to maintain at least 70 % of tree species and 72 % of shrub species found in reserves. These results show that roadside vegetation can contribute to the conservation of the flora of Cerrado. This is particularly important considering that over 60 % of the original area of this ecosystem has been destroyed and that less than 4 % is protected. With 32,012 km of roads in the Cerrado alone, the roadside vegetation may represent a cumulative area of 96,000 of hectares, an area larger than many of the Cerrado’s national parks.
Similar content being viewed by others
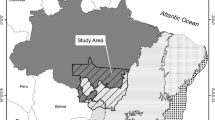
Avoid common mistakes on your manuscript.
Introduction
Roads are very important to local and national economic development. However, they can also be major causes of biodiversity loss (Fearnside 1990; Forman and Alexander 1998; Trombulak and Frissell 2000) because the access provided by them to previously isolated areas increases rates of deforestation (Trombulak and Frissell 2000; Fearnside et al. 2009). Roads may also affect hydrological processes via erosion and the disruption of watercourses (Campbell and Doeg 1989; Jones et al. 2000), act as barriers to dispersal (Mader 1984; Shepard et al. 2008), and alter animal behavior due to noise pollution (Reijnen et al. 1996). Finally, vehicle traffic can kill wild animals (Clevenger et al. 2003; Coelho et al. 2008; Bager and Rosa 2010) and generate dust and pollutants that have deleterious effects on roadside vegetation (Rogers et al. 1979; Thompson et al. 1984).
One of the principal negative impacts of roads is the introduction and spread of exotic and invasive plant species (Parendes and Jones 2000; Gelbard and Belnap 2003). Road construction exposes soil in which the seeds attached to tires (Veldman and Putz 2010) can germinate, increases light availability, and results in frequent fires that reduce native plant biomass and the availability of some soil nutrients (Dantonio and Vitousek 1992). While the way in which roads lead to the establishment of invasive taxa is increasingly well understood, surprisingly little is known about the structure of native plant communities along roadsides and the extent to which they persist trought the associated disturbances (Way 1977; Dunnett et al. 1998; Spooner and Smallbone 2009). If a sufficient proportion of native plant species are able to persist in roadsides verges, these areas could be an important component of conservation and restoration strategies (sensu Haan et al. 2012).
Not all native plant species will have the same likelihood of persisting when biotic and abiotic conditions change along roadsides. For example, frequent fires can favor species with thick bark and greater capacity for regrowth, both of which are traits that are positively correlated with resistance to fire (Coutinho 1990; Uhl and Kauffman 1990; Hengst and Dawson 1993). Similarly, the reduced density of animals along roadsides (Clevenger et al. 2003; Coelho et al. 2008; Bager and Rosa 2010) and absence of some birds due to noise pollution (Reijnen et al. 1996) may limit the recruitment of plant species that depend on these animals for dispersal. Nevertheless, few studies have evaluated the characteristics of the species that survive in roadside environments (Parendes and Jones 2000; Zeng et al. 2011).
Most investigations of plant communities along roadsides have been conducted in the temperate zone, especially grasslands in Europe (Way 1977; Ullmann and Heindl 1989; Dunnett et al. 1998; Cousins 2006) and China (Zhao et al. 2008; Leung et al. 2009; Zeng et al. 2011). However, the economic expansion of many developing countries in the tropics has led to a tremendous increase in road construction. For instance, Brazil currently has a network of paved and unpaved roads that extend more than 1.7 million kms (DNIT 2013). Besides, a major Brazilian government plan for economic growth (named “Programa de Aceleração do Crescimento”) proposes investments of over US$ 25 billion for the construction of 8,000 km of new roads and the widening of 55,000 km of existing roads by 2014 (Agência Brasil 2013). This proposed expansion is taking place with a paucity of data on the ecological impacts of road construction beyond a few studies focusing on wildlife roadkill (Vieira 1996; Gumier-Costa and Sperber 2009; Bager and Rosa 2010). Similar plans for the expansion of road networks have been put forward in other species-rich tropical locations (e.g., the Trans-African Highway, the Indian North–South-East–West Corridor; Laurance et al. 2006).
The savanna woodland of central Brazil known as the Cerrado, is a biodiversity hotspot with an estimated 12,000 plant species, of which approximately one third are endemic (Mendonça et al. 2008). Like many savanna ecosystems, the Cerrado is actually a gradient of vegetation types from open grasslands to closed canopy forests, all of which are influenced by frequent fires (Ribeiro et al. 1985; Oliveira-Filho and Ratter 2002). Because of its size (2 millions km2) and the critical role it plays in Brazil’s emergence as a global agricultural power (FAO 2004), the Cerrado’s extensive road network has been planned for expansion, making it an ideal model system to understand how roads alter the structure of native plant assemblages. The vegetation along roadsides were compared with that of nearby reserves to address the following questions:
-
(1)
Are there differences in the abundance and species richness of native and exotic plants between roadside vegetation and nearby Cerrado reserves? We predict lower abundance and richness on disturbed roadsides than in reserves given the frequent burning and other impacts in roadsides.
-
(2)
Are there differences in the proportion of individuals and species with thick bark and typical of savanna physiognomies (both proxies for adaptation to fire) between roadsides and reserves? We predict that roadsides will have a greater proportion of individuals with thick bark and from savanna physiognomies because frequent fires on roadsides lead to the loss of fire-sensitive species.
-
(3)
Are there differences in the proportion of species dispersed by animals between roadsides and reserves? We predict that roadsides will have lower proportions of species and individuals dispersed by animals due to the cumulative effects of reduced animal abundance.
Methods
Study sites
The study was conducted in areas of Cerrado sensu stricto in Minas Gerais State, Brazil. This vegetation type represents approximately 70 % of the Cerrado biome and is characterized by scattered trees, shrubs, and an herbaceous layer dominated by grasses (Eiten 1994). Average monthly temperature in the region where we conducted our surveys is 22.8 °C; the average annual rainfall is 1600 mm with more than 70 % occurring between November and March (Rosa et al. 1991; Cardoso et al. 2009). The dominant soils of the region are oxisols, with poor nutrient availability and moderate to strong acidity (Lopes and Cox 1977; Haridasan and Araújo 1987).
The surveys took place from May 2009 to March 2010. We surveyed 19 sites with remnant Cerrado vegetation along roadsides (Appendix A, Supplementary material) and 10 sites in private Cerrado reserves ranging in size from 17 to 441 hectares (Appendix B, Supplementary material). The roadside vegetation surveys were conducted in the area adjacent to the roads (Appendix C, Supplementary material) where legislation prevents any activity except the expansion of the road itself (DNIT 2013). These areas are subjected to disturbance during building of the road including the removal of vegetation and often topsoil for using in road surfacing. After the construction of the road is concluded, an area of about 20 m wide on each side of the road is abandoned allowing vegetation to regenerate. The 19 roadside sites were chosen based on their proximity to the reserves studied to minimize potential problems of geographic variation in plant species composition between sites. The sites also had similar geographic characteristics such as soil drainage and topography. There were no fires in the private reserves in the 10 years prior to our surveys (Carmo et al. 2011).
Sampling
Three strata were considered for vegetation sampling: an superior stratum predominantly of trees with trunks of circumference at breast height (CBH) ≥15 cm; an intermediate stratum comprising shrubs and trees with CBH <15 cm and ≥1 m in height, and a lower stratum composed primarily of herbaceous species with some individuals of woody taxa 20–100 cm tall. In each of the 29 sites we sampled the superior stratum in a 20 × 250 m2 (0.5 ha) plot with the exception of six roadside sites that were <20 m in width, in which case we established two plots of 10 × 250 m2 (one on each side of the road). For the intermediate stratum, we sampled an area of 10 × 250 m2 in all sites. The line intercept method (Canfield 1941; Munhoz and Araújo 2011) was used to determine the species composition and coverage of each species in the lower stratum. In each site we established two lines of 250 m each, one meter above the ground. In every other meter we recorded the length of the line that was intercepted by each individual bellow the line. To estimate the relative area covered by each species in each site the area intercepted by that species was divided by the total length of line (500 m). The species were grouped into the following categories: exotic grasses, native grasses, herbs, shrubs, trees. We recorded whether the soil was exposed or covered with litter in cases where no plants were found at a sampling point.
Vouchers of all plants we were unable to identify in the field were collected and identified with the assistance of plant taxonomists, keys to local flora, or comparison with material in the Herbarium Uberlandense (HUFU). The plants were classified according to APG III classification system (Chase and Reveal 2009).
Species traits
Three individuals of each species with CBH >20 cm were selected in the largest of our Cerrado reserves to measure the bark thickness of the species found in the superior stratum. The thickness of pieces of bark collected 30 cm above the soil surface was measured with calipers and classified each species as having thin (≤0.9 cm) or thick bark (≥1 cm). These categories were based on previous studies with 56 Cerrado tree species demonstrating that those with bark thickness >0.9 cm had a 50 % chance of surviving a fire (Hoffmann and Solbrig 2003; Hoffmann et al. 2009; Hoffmann et al. 2012).
The primary habitat preference (i.e., exclusive of savannas vs. from savannas and/or forests) of all species in both the superior and intermediate strata was identified using published studies (Mendonça et al. 2008; Rossatto et al. 2008) and our prior experience. We also used previously published literature to determine dispersal mechanisms (i.e., zoochoric vs. anemochoric/autochoric; Silva Júnior 2005; Saravy et al. 2003; Gottsberger and Silberbauer-Gottsberger 2006; Figueiredo 2008).
Data analyses
The density of individuals, species richness, and Fisher α diversity index (Fisher et al. 1943) were calculated for both the superior and intermediate strata. Fisher α was chosen because it is less influenced than other commonly used diversity indices by differences in sample size or the abundance of the most common species (Taylor et al. 1976). We used t tests to test if these parameters differed between roadsides and reserves. The density of individuals was log (x + 1) transformed to meet the assumptions of the test. The species composition of roadsides and reserves were compared using a non-metric multidimensional scaling. The NMDS ordination technique is considered one of the most effective ordination methods for ecological communities because it does not require a linear relationship between variables (McCune and Grace 2002); the ordinations were based on the relative abundance of species in each study site using the Bray–Curtis index as the similarity measure. T tests were also used to measure differences between roadsides and reserves in proportion of individuals and species with different characteristics (dispersal system, predominant physiognomy of occurrence, and bark thickness). Finally, t-tests were used with Bonferroni correction to measure for differences between roadsides and reserves in the coverage and number of species of exotic grasses, native grasses, herbs, shrubs, tree seedlings, litter, bare soil. All statistical analyzes were performed using the program Systat 10.2 (SPSS 2000).
Results
Structure and species composition of the superior stratum
A total of 6,649 plants were recorded in the 10 Cerrado reserves and 5,152 in the 19 roadside sites. There were a total of 108 species from 40 families in the reserves, including four families sampled only in these sites (Combretaceae, Lauraceae, Myristicaceae, Symplocaceae). Roadside sites had a total of 99 species from 39 families, including the only representatives of the Meliaceae, Solanaceae, and Urticaceae (Appendix D, Supplementary material). The five most common species in reserves were Qualea grandiflora, Miconia albicans, Caryocar brasiliense, Qualea parviflora, and Ouratea hexasperma (Fig. 1a), which occurred in 90 % of the reserves sampled and together accounted for 28 % of individuals found. In contrast, these species occurred in only 34 % of the roadsides, representing only 6 % of the total individuals sampled there. The most common species on roadsides were Dalbergia miscolobium, Aspidosperma tomentosum, Pouteria ramiflora, Qualea grandiflora, Pouteria torta (73 % of the roadside sites, 25 % of all individuals), while these species occurred in 86 % of the reserves and represented 15 % of the individuals sampled.
Mean abundance of species in the a superior and b intermediate strata in reserves (n = 10) and roadsides (n = 19). The species were ranked according to their mean abundance in reserves and presence in more than half of the 29 sampled sites. Of the 64 species in the superior stratum, 44 had a higher mean abundance in the reserves while 20 were more abundant in the roadsides. Of the 104 species of the intermediate stratum, 70 had a higher mean abundance in reserves while 34 were more abundant in roadsides. We highlight the five most abundant species in reserves (bold) and roadsides
The 2.4 fold difference in plant density between reserve and roadside plots (1,329.8 ± 333.4 SD individuals ha−1 vs. 542.3 individuals ha−1 ± 154.4 SD, respectively) was significantly different (t = 8.17, P < 0.001, Fig. 2). Species richness per site was also significantly higher in reserves than along roadsides (53.2 ± 8.5 SD and 38.7 ± 5.4 SD, respectively; t = 5.57, P < 0.001). Fisher α, however, did not differ between the two habitats (t = 1.26, P = 0219). In addition to the differences in plant abundance and species richness, there were also clear differences in community composition based on the NMDS (stress = 0.196; proportion of variance explained = 0.783; Fig. 3).
Roadsides had a significantly lower percentage of ‘forest’ species (25.2 ± 5.9 SD vs. 19.6 ± 4.0 SD, t = 3.00, P = 0.006; Fig. 4a) and individuals (33.1 ± 14.8 SD vs. 18.5 ± 7.5 SD; t = 3.54, P = 0.001; Fig. 4b). There was no significant difference in the proportions of animal-dispersed species (t = 0.05, P = 0.957) and individuals (t = 1.80, P = 0.082) between roadsides and reserves. Finally, 37.4 % ± 7.7 SD of the individuals in the reserves belong to thin-bark species group, while in the roadsides the figure was only 24.1 % ± 6.5 SD (t = 4.66, P < 0.0001). There were also proportionately more species with thin bark in reserves than roadsides 36.1 % ± 3.8 SD vs. 31 % ± 4.5 SD; t = 3.0922, P = 0.0044).
Structure and species composition of the intermediate stratum
A total of 11,596 individual plants occurred in reserves and 12,855 along roadsides. There were 180 species from 50 families in reserves and including the only representatives of the Amaranthaceae, Myristicaceae, Oxalidaceae, Peraceae, Siparunaceae, Symplocaceae. Roadsides plots had a total of 239 species from 50 families with the Convolvulaceae, Emmotaceae, Menispermaceae, Phyllanthaceae, Polygalaceae, and Smilacaceae unique to roadsides (Appendix E, Supplementary material). The five most common species in the reserves were M. albicans, Matayba guianensis, Q. grandiflora, Eugenia calycina, Davilla elliptica (Fig. 1b). Together these species accounted for 32 % of individuals sampled and occurred in 94 % of the reserves. On roadsides, 34.5 % of individuals were from one of five species—Matayba guianensis, Banisteriopsis malifolia, Brosimum gaudichaudii, Bauhinia holophylla, Byrsonima intermedia—and these species occurred in 79 % of all roadsides sites.
On average there were 1.7 times more individuals (t = 3.82, P < 0.001) and 25 % more species (t = 3.77, P < 0.001) in reserves than on roadsides (density: 2319.3 ± 780.69 SD individuals ha−1 vs. 1353.1 ± 495.86 SD individuals ha−1; Fig. 2b). Although, there was only a marginal significance of higher α diversity in reserves than on roadsides (t = 1.96, P = 0.06), the NMDS indicated that the two communities clearly differed in their species composition (Stress = 0.207; Proportion of variance explained = 0.760; Fig. 3b).
The proportion of “forest” species was 28 % higher in the reserves (0.222 ± 0.046 SD vs. 0.173 ± 0.032 SD, respectively; t = 3.33, P = 0.002; Fig. 4a), whereas the proportion of “forest” individuals did not differ significantly between habitat types (0.3984 ± 0.161 vs. 0.305 ± 0.147 SD, respectively; t = 1.56, P = 0128; Fig. 4b). There were 15 % fewer individuals (0.717 ± 0.083 SD vs. 0.611 ± 0.112 SD, respectively; t = 2.61, P = 0.015) and 11 % fewer species (0.656 ± 0.0359 SD vs. 0.585 ± 0.052 SD, respectively; t = 3.79, P = 0.001) that depend on specialized dispersal agents in roadsides than in reserves.
Structure and species composition of the lower stratum
A total of 221 species were recorded in the lower stratum of the reserves: 37 native grasses, three exotic grasses, 44 herbaceous species, and 137 woody species. In roadsides there were 240 species, including 25 native grasses, six exotic grasses, 73 herbaceous species, and 136 woody species. Plots in reserves contained three times more species of native grasses (12.8 ± 2.52 SD vs. 3.9 ± 2.73 SD, respectively; t = 8.48, P < 0.001, Fig. 5) covering 35 % ± 16.1 SD of the soil surface while in roadsides they covered only 4 % ± 5 SD (t = 7.59, P < 0.001). Differences between roadsides and reserves in the species richness of exotic grasses were not significant (1.5 ± 0.7 SD vs. 2.21 ± 1.13 SD; t = 1.79, P = 0.084). However, on roadsides exotic grasses covered 81.5 % ± 6.9 SD of the surface, with most of this coverage of species of the genera Brachiaria (79.8 % ± 8 SD of total coverage). This is in sharp contrast to the reserves, in which exotic grasses covered only 3 % ± 5.4 SD of the soil surface (t = 31.12, P < 0.001). Echinolaena inflexa, Tristachya leiostachya, and Loudetiopsis chrysothrix were the most common of native grasses in the reserves.
There was no significant difference between roadsides and reserves in either the number of herbaceous species per plot (9.9 ± 3.7 SD vs.9.6 ± 4.4 SD; t = 0.13, P = 0.896), nor in their total coverage per plot (3.2 ± 1.9 SD vs. 2.47 ± 1.6 SD; t = 1.07, P = 0290). However, the dominant species in each habitat were different. Ananas ananassoides and Andira humilis were 42 % ± 27.8 SD of the herbaceous ground cover in reserves, whereas on roadsides the dominant herbaceous species were Stylosanthes sp. 3 and Stylosanthes guianensis (21 % ± 28.3 SD of herbaceous cover).
Species richness of trees and shrubs (<20 cm in height) in plots located in reserves was double that of roadside plots (58 ± 12.48 SD vs. 29.42 ± 10.78 SD, t = 6.42, P < 0.001). They also covered an area in plots three times greater than in reserves than roadsides (27 % ± 12.6 SD vs.9.1 ± 4.5 SD; t = 5.60, P < 0.001). The dominant species also tended to differ between habitats: M. albicans, M. guianensis, Syagrus comosa, Cordiera myrciifolia, Cordiera obtusa represented 31 % of the woody individuals sampled in the reserves, whereas 38 % of the individuals sampled in roadsides were M. guianensis, B. intermedia, B. malifolia, Campomanesia adamantium, Dalbergia miscolobium. Roadsides and reserves differed in litter cover (29 % ± 12.4 SD in reserves and 1 % ± 1.2 SD on roadsides; t = 9.96, P < 0.001), while there was only a marginal significance of more exposed soil in reserves (t = 1.86, P = 0.074).
Discussion
The study of roads and their ecological impacts has emerged as an important area of research in conservation biology (Hughes et al. 1997; Forman and Alexander 1998). To date, however, most studies assessing changes in plant communities along roadsides have been conducted in the temperate zone (Dunnett et al. 1998; Way 1977; Zeng et al. 2011 but see Hogbin et al. 1998; Spooner and Smallbone 2009). To our knowledge, the present study is the first of its type conducted in Neotropical savannas—a highly threatened and species rich biodiversity hotspot. Based on our sample of over 36 000 plants, we found that the number of both individuals and species along roadsides is lower than in nearby natural areas, as might be expected given the disturbances that accompany roads. However, we also found that ca. 70 % of the 180 species found in the Cerrado reserves we sampled were also found in our roadside sites. This suggests roadside verges may play an important role in conserving Cerrado biodiversity, as a stepping stone for isolated animal populations, and as a reservoir of plant genetic diversity.
In the light of the focus on roads as a conduit for plant invasions, it is perhaps not unexpected that so little is known regarding the traits of species that manage to persist in roadside habitats (Parendes and Jones 2000; Zeng et al. 2011). Thick bark enhances survivorship of plants exposed to fire (Uhl and Kauffman 1990; Hengst and Dawson 1993), which is probably the mechanism underlying why plots on roadsides had fewer plants with thin bark than those in reserves. Frequent fires may also be why species and individuals typical of savanna formations were significantly more prevalent in the reserves than in roadsides. Savanna species tend to have a greater capacity for resprouting after fire due to large investments in underground biomass (Hoffmann and Moreira 2002; Hoffmann and Franco 2003), while forest species are adapted to flower and set seeds in environments with little fire (Coutinho 1977; Landim and Hay 1995). Thus, while forest species are selected against on roadsides, they succeed in protected areas with low fire frequency.
We also observed changes in traits related to plant-animal interactions that may also have helped shape plant communities and abundance on roadsides. Seed dispersal on roadsides may be reduced due to the absence of some mammals, birds, or insects (Fahrig and Rytwinski 2009) in much the way it is in other disturbed habitats (Frankel and Soulé 1981); over time this may lead to reductions in the recruitment of obligatory zoochoric species. Our results are consistent with this hypothesis—although it is unknown if disperser abundance is lower on roadsides than in Cerrado reserves, in roadside sites we found a lower number of species and individuals in the intermediate stratum that depend on animals for dispersal. Although this pattern was not observed in the superior strata, this is likely because insufficient time has passed since road establishment to observe changes in the adult tree community resulting from reduced disperser abundance (Vanthomme et al. 2010).
Burning also kills woody plant seedlings and adults (Sato and Miranda 1996), which can reduce local density and diversity (Hoffmann 1998) as well as increase the presence of invasive grasses (Milberg and Lamont 1995). These grasses, such as species of Brachiaria and Melinis minutiflora, produce large amounts of biomass providing fuel for fires (Coutinho 1990). This biomass can also inhibit the establishment of seedlings or reduce their growth and survivorship (Hoffmann et al. 2004; Hoffmann and Haridasan 2008). We found a lower number of regenerating woody species in roadsides, suggesting seedling establishment is indeed reduced in these locations. That an average of 29 woody species are found in the lower stratum, however, suggests the mechanisms underlying recruitment are complex; potential factors permitting these species to become established include larger seeds sizes (Hobbs and Mooney 1998), the absence of predators (Hobbs and Mooney 1998) or presence of secondary dispersers (Bangert et al. 2005), and differences in seed rain and propagule pressure (Gilpin and Soulé 1986). Further observations and careful experiments are needed to distinguish among these possibilities and elucidate why some species appear able to establish despite the detrimental effects of exotic grasses (Hoffmann and Haridasan 2008).
Although roadsides contain a large proportion of the species found in reserves, it is notable that the occurrence of some characteristic Cerrado species is very low in these locations. For instance, Qualea grandiflora, Caryocar brasiliense, Miconia albicans were the three most common species in the superior stratum and were also very common in the intermediate class, but they were rare or missing in roadside plots (Fig. 1a). Despite the similarity of these results, however, the lower occurrence of these species is probably caused by other factors. For Q. grandiflora the mechanism appear to be primarily biotic, namely the poor competitive ability of its seedlings against invasive grasses (Hoffmann and Haridasan 2008). In contrast, M. albicans is very sensitive to fire, which can both alter its phenology and topkill large plants (Hoffmann 1998; Hoffmann and Solbrig 2003). These fires may also explain why the five most abundant species on roadsides have thick bark (1.72 cm ± 0.43 SD), and hence are more characteristic of savanna physiognomies. These results show why one must be cautious when attempting to generalize about the mechanisms responsible for declines of populations on roadsides - failure to identify the correct mechanisms could result in incorrect or inefficient strategies for management and conservation. They also show that while roadsides appear to be reservoirs of a large proportion of Cerrado plant diversity, reserves away from roads are needed to maintain larger populations of some characteristic elements of the Cerrado flora.
Several important caveats to our conclusions should be mentioned. First, we did not make allowance for the age of roads in our analyses. The roads we studied were built 24-48 years ago and while studies of roads of similar ages have revealed patterns comparable to ours (Sýkora et al. 2002; Zeng et al. 2011), older roads have previously been shown to maintain a larger number of individuals (Spooner and Smallbone 2009). With the exception of a major highway (BR-050; Appendix A), however, most of the roads in the Cerrado region are similar in age to those used in our study, suggesting our results will be geographically applicable. Second we have no data on the frequency with which our roadside verges were burned. Some of the negative effects we observed should therefore be interpreted cautiously because fire frequency is an important predictor of vegetation structure (Hoffmann and Moreira 2002). Finally, paved roads may (Barbosa et al. 2010) or may not (Craig et al. 2010) contain more exotic species than those unpaved because of the chemicals used in their construction. Four of our roadside sites were unpaved, but there is no evidence that the vegetation bordering them is different in composition or plant abundance. Nevertheless, we suggest future work should emphasize increased sampling along unpaved roads to study possible effects of paving. Finally, we have no information on plant abundance and community composition in our sites prior to road construction, it is possible—although we believe unlikely—that some of the differences we observed existed prior to road construction.
Conclusion
Even small habitat patches such as the vegetation along roadsides can play an important role in biodiversity conservation (Hogbin et al. 1998; Cousins 2006; Spooner and Smallbone 2009; Zeng et al. 2011). Indeed, in the Netherlands more than half the country’s flora can be found on roadsides (Sýkora et al. 2002), while in England plant species diversity in these environments is 43.5 % higher (Way 1977). Roadsides are also important sites for the restoration of native plant communities (Norcini et al. 2008, 2009), and which may reduce the risk of fire (Velloso and Andrade 2008), erosion and pollution (Dolan et al. 2006). In Australia there is a well implemented program that balances road safety and infrastructure with biodiversity conservation of roadsides through management (www.coorong.sa.gov.au/page.aspx?u=439, Stokes et al. 2006). Federal legislation in Brazil (Law No 6766) requires that a 15 meter buffer strip adjacent to the road remains undisturbed. Based on 32,012 km of roads in the Cerrado alone (DNIT 2013), this represents a total area of over 96,000 hectares—a cumulative area larger than several of the Cerrado region’s flagship state and national parks. Our results indicate that while these buffer strips are susceptible to colonization by invasive grasses, they can also harbor a large diversity of native plants. This could make roadside verges an essential component of conservation strategies for highly fragmented biodiversity hotspots (Cavalcanti and Joly 2002) in which less than 4 % is formally protected in conservation units (Klink and Machado 2005).
References
Agência Brasil (2013) http://agenciabrasil.ebc.com.br/noticia/2013-02-22/pac-2-investimentos-em-obras-de-transporte-totalizam-r-277-bilhoes-em-dois-anos. Accessed 2 July 2014
Bager A, Rosa CA (2010) Priority ranking of road sites for mitigating wildlife roadkill. Biota Neotrop 10(4):149–154
Bangert RK, Turek RJ, Martinsen GD (2005) Benefits of conservation of plant genetic diversity on arthropod diversity. Conserv Biol 19:379–390
Barbosa NPU, Wilson Fernandes G, Carneiro MAA, Junior LAC (2010) Distribution of non-native invasive species and soil properties in proximity to paved roads and unpaved roads in a quartzitic mountainous grassland of southeastern Brazil (rupestrian fields). Biol Invasions 12(11):3745–3755
Campbell IC, Doeg TJ (1989) Impact of timber harvesting and production on streams—a review. Aust J Mar Fresh Res 40(5):519–539
Canfield R (1941) Application of line interception in sampling range vegetation. J Forestry 39:388–394
Cardoso E, Moreno MIC, Bruna EM, Vasconcelos HL (2009) Mudanças fitofisionômicas no cerrado: 18 anos de sucessão ecológica na estação ecológica do Panga, Uberlândia - MG. RCG - revista on line 10:254–268
Carmo AB, Vasconcelos HL, Araújo GM (2011) Estrutura da comunidade de plantas lenhosas em fragmentos de cerrado: relação com o tamanho do fragmento e seu nível de perturbação. Rev Bras Bot 34:31–38
Cavalcanti R, Joly C (2002) The conservation of the Cerrados. In: Oliveira PS, Marquis RJ (eds) The Cerrado of Brazil. Ecology and natural history of a neotropical savanna. Columbia University Press, New York, pp 351–367
Chase MW, Reveal JL (2009) A phylogenetic classification of the land plants to accompany APG III. Bot J Linn Soc 161(2):122–127
Clevenger AP, Chruszczc B, Gunson KE (2003) Spatial patterns and factors influencing small vertebrate fauna road-kill aggregations. Biol Conserv 109(1):15–26
Coelho IP, Kindel A, Coelho AVP (2008) Roadkills of vertebrate species on two highways through the Atlantic Forest Biosphere Reserve, southern Brazil. Eur J Wildl Res 54(4):689–699
Cousins SAO (2006) Plant species richness in midfield islets and road verges—the effect of landscape fragmentation. Biol Conserv 127(4):500–509
Coutinho LM (1977) Aspectos ecológicos do fogo no cerrado: as queimadas e a dispersão de sementes de algumas espécies anemocóricas do estrato herbáceo e subarbustivo. B Bot USP 5:57–64
Coutinho LM (1990) Fire in the ecology of the Brazilian Cerrado. In: Goldammer JG (ed) Fire in the Tropical Biota. Springer-Verlag, Berlin, pp 82–105
Craig DJ, Craig JE, Abella SR, Vanier CH (2010) Factors affecting exotic annual plant cover and richness along roadsides in the eastern Mojave Desert, USA. J Arid Environ 74(6):702–707
Dantonio CM, Vitousek PM (1992) Biological invasions by exotic grasses, the grass fire cycle and global change. Annu Rev Ecol Syst 23:63–87
DNIT (2013) Departamento Nacional de Infraestrutura de Transportes; Manual de vegetação rodoviária, vol 2, Flora dos ecossistemas brasileiros http://www.dnit.gov.br/planejamento-e-pesquisa/planejamento/planejamento-rodoviario. Accessed 11 June 2013
Dolan LMJ, Van Bohemen H, Whelan P, Akbar KF, O’Malley V, O’Leary G, Keizer PJ (2006) Towards the sustainable development of modern road ecosystem. In: Davenport J, Davenport JL (eds) The ecology of transportation: managing mobility for the environment. Springer, Dordrecht, pp 275–331
Dunnett NP, Willis AJ, Hunt R, Grime JP (1998) A 38-year study of relations between weather and vegetation dynamics in road verges near Bilbury, Gloucestershire. J Ecol 86:610–623
Eiten G (1994) Cerrado: caracterização, ocupação e perspectiva. Universidade de Brasília, Brasília, Vegetação do cerrado
Fahrig L, Rytwinski T (2009) Effects of roads on animal abundance: an empirical review and synthesis. Ecol Soc 14(1):21
FAO (2004) Fertilizer use by crop in Brazil. FAO, Rome
Fearnside PM (1990) A ocupação humana de Rondônia: impactos, limites e planejamento. CH 11(61):47–52
Fearnside PM, Graca PMLDA, Keizer EWH, Maldonado FD, Barbosa RI, Nogueira EM (2009) Modeling of deforestation and greenhouse-gas emissions in the area of influence of the Manaus-Porto Velho (BR-319) highway. Rev Bras Meteorol 24(2):208–233
Figueiredo PS (2008) Fenologia e estratégias reprodutivas das espécies arbóreas em uma área marginal de cerrado, na transição para o semi-árido no nordeste do Maranhão, Brasil. R Trop Ci Agr Biol 2:1–8
Fisher RA, Corbet AS, Willians CB (1943) The relation between the number of species and the number of individuals in a random sample of an animal population. J Anim Ecol 12:42–58
Forman RTT, Alexander LE (1998) Roads and their major ecological effects. Annu Rev Ecol Syst 29:207–231
Frankel OH, Soulé ME (1981) Conservation and evolution. Cambridge University Press, Cambridge
Gelbard JL, Belnap J (2003) Roads as conduits for exotic plant invasions in a semiarid landscape. Conserv Biol 17(2):420–432
Gilpin ME, Soulé ME (1986) Minimum viable populations: the processes of species extinctions. In: Soulé M (ed) Conservation biology: the science of scarcity and diversity. Sinauer Associates, Sunderland, pp 13–34
Gottsberger G, Silberbauer-Gottsberger I (2006) Life in the cerrado. A South American tropical seasonal ecosystem, vol II. Pollination and seed dispersal.Reta Verlag, Ulm
Gumier-Costa F, Sperber CF (2009) Roadkills of vertebrates in Carajas National Forest, Para, Brazil (Atropelamentos de vertebrados na Floresta Nacional de Carajas, Para, Brasil). Acta Amaz 39(2):459–466
Haan NL, Hunter MR, Hunter MD (2012) Investigating predictors of plant establishment during roadside restoration. Restor Ecol 20(3):315–321
Haridasan M, Araújo GM (1987) Aluminium accumulating species in two forest communities in the cerrado region of central Brazil. Forest Ecol Manag 24:15–26
Hengst GE, Dawson JO (1993) Bark properties and fire resistance of selected tree species from the central hardwood region of North America. Can J For Res 24:688–696
Hobbs RJ, Mooney HA (1998) Broadening the extinction debate: population deletions and additions in California and Western Australia. Conserv Biol 12:271–283
Hoffmann WA (1998) Post-burn reproduction of woody plants in a neotropical savanna: the relative importance of sexual and vegetative reproduction. J Appl Ecol 35:422–433
Hoffmann WA, Franco AC (2003) Comparative growth analysis of tropical forest and savanna woody plants using phylogenetically-independent contrasts. J Ecol 91:475–484
Hoffmann WA, Haridasan M (2008) The invasive grass, Melinis minutiflora, inhibits tree regeneration in a neotropical Savanna. Austral Ecol 33(1):29–36
Hoffmann WA, Moreira AG (2002) The role of fire in population dynamics of woody plants. In: Oliveira PS, Marquis RJ (eds) The Cerrados of Brazil: ecology and natural history of a neotropical Savanna. Columbia University Press, New York, pp 159–177
Hoffmann WA, Solbrig OT (2003) The role of topkill in the differential response of savanna woody species to fire. For Ecol Manag 180(1–3):273–286
Hoffmann WA, Lucatelli VMPC, Silva FJ, Azeuedo INC, Marinho MD, Albuquerque AMS, Lopes AD, Moreira SP (2004) Impact of the invasive alien grass Melinis minutiflora at the savanna-forest ecotone in the Brazilian Cerrado. Divers Distrib 10(2):99–103
Hoffmann WA, Adasme R, Haridasan M, de Carvalho MT, Geiger EL, Pereira MAB, Gotsch SG, Franco AC (2009) Tree topkill, not mortality, governs the dynamics of savanna-forest boundaries under frequent fire in central Brazil. Ecology 90(5):1326–1337
Hoffmann WA, Geiger EL, Gotsch SG, Rossatto DR, Silva LCR, Lau OL, Haridasan M, Franco AC (2012) Ecological thresholds at the savanna-forest boundary: how plant traits, resources and fire govern the distribution of tropical biomes. Ecol Lett 15(7):759–768
Hogbin PM, Ayre DJ, Whelan RJ (1998) Genetic variation and reproductive success of road verge populations of the rare shrub Grevillea barklyana (Proteaceae). Heredity 80:180–186
Hughes JB, Daily GC, Ehrlich PR (1997) Population diversity: its extent and extinction. Science 278:689–692
Jones JA, Swanson FJ, Wemple BC, Snyder KU (2000) Effects of roads on hydrology, geomorphology, and disturbance patches in stream networks. Conserv Biol 14(1):76–85
Klink CA, Machado RB (2005) Conservation of the Brazilian Cerrado. Conserv Biol 19(3):707–713
Landim MF, Hay JD (1995) Impacto do fogo sobre alguns aspectos da biologia reprodutiva de Kielmeyera coriacea Mart. R Bras Biol 56:127–134
Laurance WF, Nascimento HEM, Laurance SG, Andrade A, Ribeiro JELS, Giraldo JP, Lovejoy TE, Condit R, Chave J, Harms KE, D’Angelo S (2006) Rapid decay of tree-community composition in Amazonian forest fragments. P Natl Acad Sci USA 103(50):19010–19014
Leung GPC, Hau BCH, Corlett RT (2009) Exotic plant invasion in the highly degraded upland landscape of Hong Kong, China. Biodivers Conserv 18(1):191–202
Lopes AS, Cox FR (1977) A survey of the fertility status of surface soils under cerrado vegetation in Brazil. Soil Sci Soc Am J 41:742–747
Mader HJ (1984) Animal habitat isolation by roads and agricultural fields. Biol Conserv 29(1):81–96
McCune B, Grace JB (2002) Analysis of ecological communities. MjM Software Design, Gleneden Beach
Mendonça RC, Felfili JM, Walter BMT, Silva Júnior MC, Rezende AV, Filgueiras TS, Nogueira PE, Fagg CW (2008) Flora vascular do Bioma Cerrado: checklist com 12.356 espécies. In: Sano SM, Almeida SP, Ribeiro JF (eds) Cerrado: ecologia e flora. Embrapa Cerrados, Brasília, pp 423–1279
Milberg P, Lamont BB (1995) Fire enhances weed invasion of roadside vegetation in southwestern Australia. Biol Conserv 73(1):45–49
Munhoz CBR, Araújo GM (2011) Métodos de Amostragem do estrato herbáceo-subarbustivo. In: Felfili JM, Eisenlohr PV, Melo MMRF, Andrade LA, Meira Neto JAA (eds) Fitossociologia no Brasil: Métodos e Estudos de Casos, vol 1. UFV: Viçosa, pp 213–230
Norcini JF, Frances AL, Adams CR (2008) Establishment of Lanceleaf Tickseed (Coreopsis lanceolata) in Roadside Right-of-Ways. EDIS Publ ENH 1103 http://www.edisifasufledu/ep367, Environmental Horticulture Department, University of Florida, Gainesville
Norcini JF, Frances AL, Adams CR (2009) Establishment of Leavenworth’s Tickseed (Coreopsis leavenworthii) on Roadside Right-of-Ways. EDIS Publ ENH1104, http://www.edisifasufledu.com/ep368 Department of Environmental Horticulture, Fla Coop Ext Serv, Institute of Food and Agricultural Sciences, University of Florida, Gainesville
Oliveira-Filho AT, Ratter JT (2002) Vegetation physiognomies and woody flora of the Cerrado biome. In: Oliveira PSMR (ed) The Cerrados of Brazil: ecology and natural history of a Neotropical savanna. Columbia University Press, New York, pp 91–120
Parendes LA, Jones JA (2000) Role of light availability and dispersal in exotic plant invasion along roads and streams in the H. J. Andrews Experimental Forest, Oregon. Conserv Biol 14(1):64–75
Reijnen R, Foppen R, Meeuwsen H (1996) The effects of traffic on the density of breeding birds in Dutch agricultural grasslands. Biol Conserv 75(3):255–260
Ribeiro JF, Silva JCS, Batmanian GJ (1985) Fitossociologia de tipos fisionômicos de Cerrado em Planaltina (DF). Rev Bras Bot 8:131–142
Rogers HH, Jeffries HE, Witherspoon AM (1979) Measuring air pollution uptake by plants: nitrogen dioxide. J Environ Qual 8:551–557
Rosa R, Lima SC, Assunção WL (1991) Abordagem preliminar das condições climáticas de Uberlândia (MG). S & N 3:91–108
Rossatto DR, Toniato MTZ, Durigan G (2008) Non-arboreal phanerogamic cerrado flora of Assis Ecological Station, state of São Paulo. Rev Bras Bot 31:409–424
Saravy FP, Freitas PJ, Lage MA, Leite SJ, Braga LF, Sousa MP (2003) Síndrome de dispersão em estratos arbóreos em um fragmento de floresta ombrófila aberta e densa em alta floresta—MT. RCAA 2:1–12
Sato MN, Miranda HS (1996) Mortalidade de plantas lenhosas do cerrado sensu stricto submitidos a diferentes regimes de queima. In: Miranda HS, Saito CH, Dias BFDS (eds) Impactos de queimadas em áreas de cerrado e restinga. Universidade de Brasília, Brasília, pp 102–111
Shepard DB, Kuhns AR, Dreslik MJ, Phillips CA (2008) Roads as barriers to animal movement in fragmented landscapes. Anim Conserv 11(4):288–296
Silva Júnior MC (2005) 100 Árvores do Cerrado: guia de campo. Rede de Sementes do Cerrado, Brasília
Spooner PG, Smallbone L (2009) Effects of road age on the structure of roadside vegetation in south-eastern Australia. Agric Ecosyst Environ 129(1–3):57–64
SPSS (2000) Systat version 10. SPSS Inc, San Francisco
Stokes AL, Heard LMB, Carruthers S, Reynolds T (2006) Guide to roadside vegetation survey methodology in south Australia. Draft report Department for Environment and Heritage, Adelaide Working Document
Sýkora KV, Kalwij JM, Keizer PJ (2002) Phytosociological and floristic evaluation of a 15-year ecological management of roadside verges in the Netherlands. Preslia 74:421–436
Taylor LR, Kempton RA, Woiwod IP (1976) Diversity statistics and the log-series model. J Anim Ecol 45:255–272
Thompson JR, Mueller PW, Fluckiger W, Rutter AJ (1984) The effect of dust on photosynthesis and its significance for roadside plants. Environ Pollut 34:171–190
Trombulak SC, Frissell CA (2000) Review of ecological effects of roads on terrestrial and aquatic communities. Conserv Biol 14(1):18–30
Uhl C, Kauffman JB (1990) Deforestation, fire susceptibility, and potential tree responses to fire in the eastern Amazon. Ecology 71:436–449
Ullmann I, Heindl B (1989) Geographical and ecological differentiation of roadside vegetation in temperate Europe. Bot Acta 102(4):261–269
Vanthomme H, Belle B, Forget P (2010) Bushmeat hunting alters recruitment of large-seeded plant species in central Africa. Biotropica 42:672–679
Veldman JW, Putz FE (2010) Long-distance dispersal of invasive grasses by logging vehicles in a tropical dry forest. Biotropica 42(6):697–703
Velloso SL, Andrade CFS (2008) Queimadas e o projeto de arborização da rodovia Rio-Santos (BR-101 - município de Paraty). Rev Ed Amb BE597 1:4-13
Vieira EM (1996) Highway mortality of mammals in central Brazil. Cien Cult 48(4):270–272
Way JM (1977) Roadside verges and conservation in Britain: a review. Biol Conserv 12:65–74
Zeng SL, Zhang TT, Gao Y, Ouyang ZT, Chen JK, Li B, Zhao B (2011) Effects of road age and distance on plant biodiversity: a case study in the Yellow River Delta of China. Plant Ecol 212(7):1213–1229
Zhao J, Ma Y, Zhu H, Li H, Liu W, Li Z (2008) Invasion patterns of seven alien plant species along roadsides in southern mountainous areas of Yunnan Province. Biodivers Sci 16(4):369–380
Acknowledgments
We thank Heraldo L. Vasconcelos for helpful discussions and comments on the manuscript. We also thank the experts who helped with identification of plant vouchers: A. Angélica, I. Schiavini, A. Terra, J. Nakajima, E. K. O. Hattori, B. C. Vargas, A. R. Rezende, R. Romero, D. Marques, G. Paiva, P. Mel, and P. Oliveira. Financial support was provided by a graduate scholarship from Coordenação de Aperfeiçoamento de Pessoal de Nível Superior (CAPES) to PBV and a Science Without Borders Visiting Researcher Fellowship to EMB (Project No. 061/2012).
Author information
Authors and Affiliations
Corresponding author
Additional information
Communicated by Jefferson Prado.
Electronic supplementary material
Below is the link to the electronic supplementary material.
Rights and permissions
About this article
Cite this article
Vasconcelos, P.B., Araújo, G.M. & Bruna, E.M. The role of roadsides in conserving Cerrado plant diversity. Biodivers Conserv 23, 3035–3050 (2014). https://doi.org/10.1007/s10531-014-0762-y
Received:
Revised:
Accepted:
Published:
Issue Date:
DOI: https://doi.org/10.1007/s10531-014-0762-y