Abstract
Differential migration reflects various constraints and selective forces on different individuals in a population, and has been explained by several hypotheses. Most previous studies about differential migration focused on only one aspect (e.g. spring timing, autumn timing or wintering area), which could not give a full picture of differential migration of the study population and carry out an overall investigation into the hypotheses. In this study, we analyse the migration patterns of European Ospreys Pandion haliaetus ringed in Germany based on the ring recovery database between 1980 and 2008. The migration routes of the study population form a broad front between south and southwest of the ringing area, and the wintering sites are in West Africa south of the Sahara and around the western Mediterranean. We demonstrate differential migration of the population in both spring and autumn timings as well as in the use of wintering grounds. Adult (third year and older) males return earliest to the breeding area in spring, followed by adult females and then two-year-old birds. In autumn, the adult females leave the breeding area earlier than adult males, while the migration of first-year Ospreys takes place over a wide time-span. Adult males were discovered mainly around the western Mediterranean in winter, closer to the ringing area than adult females and younger birds. We suggest that the selection for prior access to breeding resources on males shapes the patterns of differential migration in Ospreys.
Zusammenfassung
Der differenzierte Zug von Vögeln spiegelt verschiedene Selektionsfaktoren wider, die auf Individuen einer Population unterschiedlich einwirken. Er wurde mit mehreren Hypothesen erklärt, wobei die meisten bisherigen Untersuchungen über differenzierten Zug sich nur auf einen Aspekt (z. B. Frühjahrszug, Herbstzug, Überwinterungsgebiet) konzentrierten. Dadurch konnte kein Gesamtbild des Zuggeschehens einer untersuchten Population und keine umfassende Überprüfung der Hypothesen erreicht werden. Mit unserer Untersuchung analysieren wir das Zugmuster von in Deutschland über die Jahre 1980 bis 2008 nestjung beringten Fischadlern Pandion haliaetus, die in der Wiederfund-Datenbank der Beringungszentrale Hiddensee gespeichert sind. Die Zugwege der untersuchten Population verlaufen ausgehend vom Beringungsgebiet auf breiter Front zwischen südlichen und südwestlichen Richtungen. Die Überwinterungsgebiete liegen im Westen Afrikas südlich der Sahara und im westlichen Mittelmeergebiet. Wir zeigen auf, dass die Fischadler sowohl im zeitlichen Ablauf im Herbst als auch im Frühjahr einem differenzierten Zug unterliegen, genauso wie bei der räumlichen Nutzung des Überwinterungsgebietes. Adulte Männchen (3-jährige und ältere) kehren im Frühjahr zuerst ins Brutgebiet zurück, gefolgt von adulten Weibchen und dann von zweijährigen Vögeln. Im Herbst verlassen adulte Weibchen das Brutgebiet früher als adulte Männchen, wobei sich der Zug der Jungvögel noch über einen längeren Zeitraum erstreckt. Adulte Männchen wurden im Winter überwiegend im westlichen Mittelmeerraum und damit näher zum Brutgebiet gelegen gefunden als adulte Weibchen und jüngere Fischadler. Wir gehen davon aus, dass die Selektion, einen möglichst frühzeitigen Zugang zu den Brutplätzen zu erhalten, vorwiegend auf Männchen wirkt und damit das Muster des differenzierten Zuges bei Fischadlern formt.
Similar content being viewed by others
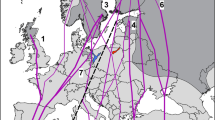
Avoid common mistakes on your manuscript.
Introduction
Differential migration, the variations in such aspects of migration as routes, timing or wintering grounds among distinguishable groups (e.g. sex or age) of a population, has been observed over diverse taxa (Berthold 2001; Nilsen et al. 1989; Stewart 1997). It is best documented in avian migration (e.g. Mazerolle and Hobson 2007; Morton 1984; Woodrey and Chadler 1997), and is suggested to be a widespread phenomenon in migratory birds (Cristol et al. 1999). The adoption of different migratory strategies may influence the reproductive success and survival of individuals (Gillis et al. 2008; Payevsky 1995), and is closely associated with their physiology, morphology and stopover ecology (Fernandez and Lank 2007; Lyons et al. 2008; O’Hara et al. 2006). Therefore, understanding the patterns and underlying mechanisms of differential migration plays an important role in the study of migratory bird species.
The coexistence of different migratory strategies in a population reflects various constraints and selective forces on different individuals. To explain the evolutionary mechanism of differential migration, several hypotheses have been proposed (see review in Kerlinger 1989; Mills 2005). These hypotheses may deal with different aspects of differential migration and are not necessarily contradictory. The variations in the choice of wintering grounds and in the timing of spring migration are often discussed together, with either one considered as the constraint and the other as the consequence. The arrival-time hypothesis emphasizes the selection for early arrival in spring (Ketterson and Nolan 1983; Myers 1981). It suggests that males are subject to intrasexual competition for the prior access to breeding territories. Therefore, they arrive earlier than females and may consequently winter closer to the breeding area. The body-size hypothesis, the dominance hypothesis and the migration-constraints hypothesis focus on different factors causing the variation in wintering grounds. The body-size hypothesis suggests that the sex with larger body size can better survive the harsh winter at high latitude and thus can winter closer to the breeding area to minimise the travelling cost (Ketterson and Nolan 1976). The dominance hypothesis (Gauthreaux 1982) argues that the dominant individuals, usually older ones, may out-compete the subordinates for food in winter, forcing the latter to migrate farther. The migration-constraints hypothesis proposes that young birds may be physiologically or behaviourally incapable of making long migrations during their first autumn, and therefore winter closer to the breeding area than adults (Prescott and Middleton 1990).
The variation in the timing of autumn migration is probably less common and less striking then that of spring migration, and has received less theoretical attention (Mills 2005). The rank-advantage hypothesis involves the same mechanism as the arrival-time hypothesis in spring migration, proposing that males may protect high quality territories for the subsequent year by outlasting competitors in autumn (Mills 2005). Thus, they are selected for late departure by intrasexual competition and remain longer in the breeding area than females. The mate-opportunity hypothesis also invokes the benefits in the subsequent year as the former hypothesis but suggests intersexual selection as the mechanism (Mills 2005). It proposes that males may maximise their future mating opportunities by remaining longer than females. The foraging efficiency hypothesis focuses on the different autumn timing between age classes (DeLong and Hoffman 1999). It suggests that young birds are less skilful in foraging and thus more sensitive to the deteriorating condition in autumn than adults. Therefore, they are expected to migrate earlier than older birds.
Although differential migration has been widely studied, most studies focused on only one aspect (e.g. spring timing, autumn timing or wintering area) of migration (e.g. Evans and Day 2001; Jenkins and Cristol 2002; Morris et al. 2003; Stewart et al. 2002). Very few have inspected the full picture of differential migration of a population and carried out an overall investigation into the hypotheses. Furthermore, most of our understanding about long-distance differential migration is derived from the study of passerines (e.g. Mazerolle and Hobson 2007; Morton 1984, Payevsky 1995; Rogers et al. 1989), waterfowl (e.g. Guillemain et al. 2009) and waders (e.g. Fernandez and Lank 2007; O’Hara et al. 2006). A comprehensive review about the migration patterns of raptors was recently given by Bildstein (2006), but information on differential migration is scarce and mostly generated from observations at migration passages or from a few individuals tracked by satellite. Individuals observed at passages may belong to different populations coming from different regions, and the patterns of differential migration could be blurred. As for satellite tracking, although it may provide very detailed information on specific individuals, the high costs often limit the sample size and thus the generalisation of the results (e.g. Mebs and Schmidt 2006; Schmidt and Meyburg 1998).
In this study, we analyse the migration patterns of the Osprey population breeding in Germany. The study population is present in the breeding area between March and October. Breeding pairs defend a small area around the nest sites and share the foraging grounds (lakes). Eggs are laid between mid-April and May, and hatch after about 38 days. Females incubate and tend the nestlings, while males forage for the whole family. Few Ospreys attempt to breed when 3 years old and most individuals start reproduction in their fourth year. Adult females average 1,800 g, about 350 g heavier than adult males (Schmidt et al. 2000). Using the long-term ring-recovery database of the Hiddensee Bird Ringing Centre (Schmidt 2009), we present the migration routes and wintering sites of German Ospreys at the population level. Then, we analyse the migration timing and wintering areas of different sex and age groups of the population, and examine the predictions of various hypotheses about differential migration (Table 1).
Methods
Osprey ringing and recovery
The Osprey was once a widespread breeding bird in Europe. Due to the extensive use of persistent organochlorine pesticides, its population crashed worldwide in the 1960s (Poole 1989). Breeding Ospreys vanished in West Germany in 1963, while a small population persisted in the former East Germany. Since the mid-1970s, the population has gradually recovered due to the ban on pesticides (Weber et al. 2003). The breeding range of Ospreys in Germany today is expanding west- and southwards (Bai et al. 2009), although 95% of the breeding population is still confined in the eastern federal states of Mecklenburg-Vorpommern, Brandenburg, Sachsen and Sachsen-Anhalt (Schmidt 2010). This population also represents the south-western front of the continuous breeding distribution of this species in Europe, apart from the small isolated subpopulations in central France and in the western Mediterranean Islands.
The first ringing of Ospreys in Germany can be traced back to 1928, but the ringing efforts in the early years were scarce and most of these old data were lost (Schmidt and Roepke 2001). Following the recovery of the Osprey population, systematic ringing activities have been carried out by independent ringers since 1980 (Roepke 1996). In 1995, a further coordinated colour-ringing programme was initiated (Schmidt 1995). Since then and until 2008, more than 7,000 Osprey nestlings have been ringed in Germany (Schmidt 2009). All the ringing data and subsequent recovery reports of the Ospreys ringed in the eastern federal states of Germany have been collected and compiled by the Hiddensee Bird Ringing Centre. Note that, in the following, "recovered" is taken to include both reports of dead birds and sightings of colour-ringed ones.
Data treatment and analysis
This study was based on the ring recovery database from the Hiddensee Bird Ringing Centre between 1980 and 2008. All records were used to demonstrate the spatial coverage of migration routes and wintering grounds of the study population on the map. In quantitative analysis, however, potential temporal and spatial bias of ring recovery data should be examined (Strandberg et al. 2009). In terms of temporal pattern, many ring recovery databases include a high proportion of dead recoveries, which could not properly represent the locations of the individuals at a specified time. Owing to the current German colour-ringing scheme, the majority of the Ospreys in our database were recovered alive. We used only the records in which the birds were observed alive or found ‘dead within 1 week’ for analysis. Although the time after death of a bird carcass can be difficult to assess, the bias was reduced by using season or month as the time unit for analysis. As for spatial pattern, the recovery and reporting rates can vary greatly in different regions, which limit the interpretation of ring recovery databases at a large scale (Strandberg et al. 2009). Recoveries are usually over-represented in regions with dense population or popular for bird-watching or hunting activities, and do not give a full picture of the spatial distribution of the population. However, assuming the recovering rate in each region is not biased by the sex and age of birds, comparison between birds of different sexes and age classes can be applied.
For each recovery record, the distance between the recovery site and the ringing site of the individual was calculated as the parameter for analyses, and hereafter referred to as the distance to ringing site. For an adult bird, the distance between the recovery site and its breeding site of the year would be a more exact indicator of its migratory distance, but the information of breeding sites was not available in the underlying database. Nevertheless, given the high natal site fidelity of the Ospreys (Schmidt et al. 2006), and more importantly the spatial scale of this study, the difference between the ringing sites and breeding sites was not likely to influence the analyses. Only very few individuals ringed as nestlings were known to breed far away (in central France). The recovery records of these individuals were excluded.
Individuals were grouped into ‘first-year birds (1Y)’, ‘second-year birds (2Y)’, ‘females third year and above (3+ female)’ and ‘males third year and above (3+ male)’. Age was counted from June; an Osprey was defined as 1Y from its birth to the end of May in the following year, 2Y from June in the second year of its life to the end of May in the third year, etc. Individuals for which the age-class could not be determined were excluded from the analysis. For birds ‘third year and above’, individuals with unidentified sex were also excluded. Preliminary analyses suggested no difference in the distance to ringing sites between sexes for 1Y and 2Y Ospreys.
Recovery months were classified into four seasons: spring (March–May = spring migration, courtship and incubation period of breeders), summer (June and July = nestling and fledging period), autumn (August–October = autumn migration) and winter (November–February = wintering). In each season, we compared the distance to ringing sites among bird groups. In spring and autumn, we further compared the distance to ringing sites in each month to that in summer and in winter, to examine the progress of migration for each bird group. As the distribution of the distance to ringing sites of each bird group differs and was often not normal, nonparametric Kruskal–Wallis test and Mann–Whitney U test were applied for comparison.
Only a few years after the start of the colour-ringing programme, many birds were identified several times per season, especially near their nest sites in spring and summer. To avoid the potential bias due to frequent recoveries of same individuals, random samples were drawn from the dataset, so that each season each year only one record per individual entered the sub-dataset for analyses. This procedure was repeated 100 times. Different sub-datasets could give rise to different test statistics in analyses, but, with the significance level of 0.05, all of them generated identical conclusions about the significance in all comparisons. The test statistics presented below were derived from one of the sub-datasets, for which the descriptive statistics were closest to the mean of the 100 sub-datasets for all bird groups in all seasons. All spatial analyses were performed in ARCGIS and all statistical analyses in SAS.
Results
Spatial distribution of recovery sites
Since 1980, a total of 3,092 recoveries of Ospreys ringed in Germany have been reported from 34 countries (Fig. 1). About 75% of these recoveries were located within the ringing area, as nest wardens intensively read the individually coded colour-rings of live birds with telescopes in the breeding season. The Ospreys recovered outside the ringing area at long distances were found widespread in west and south Europe, as well as in West Africa north and south of the Sahara (Fig. 1). The longest recovery distance to ringing site for 1Y Ospreys was about 5,637 km in the Ivory Coast, for 2Y Ospreys 5,298 km in the Gambia, for 3+ females 4,952 km in Senegal, and for 3+ males 2,365 km in southern Spain.
Recovery sites of Ospreys Pandion haliaetus ringed in Germany. As the aim was to demonstrate migration routes and wintering areas, and the recovery sites in the ringing area were too dense to be properly displayed at this scale, only the 480 records of long-distance recoveries (>300 km from ringing site) are shown
In summer, most Ospreys had returned to the ringing area to breed while some non-breeding individuals were observed in Spain, The Gambia and Cameroon. Osprey migration routes in spring and autumn were similar, which spanned between south and southwest of the ringing area. Two Ospreys found south-eastwards in autumn were reported from southern Poland and from the Ionian Islands of Greece, which were both 1Y birds and found dead. Ospreys wintered mainly around the western Mediterranean and in West Africa south of the Sahara. Winter recoveries were most frequent near Gibraltar in Spain and on the coasts of The Gambia and Senegal.
There were 6 individuals that were recovered several times in different months in the same winter. In all cases, the distances between the sites of the same individual were less than 1 km. There were 4 individuals recovered in winters of different years. The distances between the recovery sites of the same individual in different winters were less than 2 km in all four cases.
Variations related to age and sex
Summer
In summer, the majority of all Osprey groups except 2Y were recovered in the ringing area (Fig. 2a). The distance to ringing sites was shortest in 1Y Ospreys, followed by 3+ males and 3+ females (Table 2). The recovery of 2Y Ospreys in summer was scarce (n = 6), and the recovery sites were scattered. Two 2Y Ospreys were recovered returning to the ringing area, two in other parts of Europe, while two were observed south of the Sahara.
Autumn
In autumn, the 2Y Ospreys, of which many did not return to the ringing area in the previous season, were recovered at the furthest distances (Fig. 2b; Table 2). The autumn distance to ringing sites of 2Y Ospreys showed no monthly variation (Kruskal–Wallis test, H 2 = 2.1, P = 0.35), and did not differ from that in summer and that in winter (Kruskal–Wallis test, H 2 = 3.2, P = 0.21).
Ospreys of the other three groups gradually moved further away from the ringing area, during successive autumn migrations (Fig. 3a). The migration of 1Y Ospreys begins in August (Mann–Whitney U test: comparison with summer: U = 15.2, P < 0.001; comparison with winter: U = 69.2, P < 0.0001). Although most of the 1Y Ospreys were still within the ringing area in August, some were already observed in other parts of Europe. One individual even arrived in Mali south of the Sahara in the middle of August. The 1Y Ospreys proceeded with their migration in September (Mann–Whitney U test: comparison with summer: U = 37.5, P < 0.0001; comparison with winter: U = 53.7, P < 0.0001); they were mostly recovered in Europe west and south of the ringing area, while 16 were still in the ringing area and two already south of the Sahara (n = 55). In October, the migration of 1Y Ospreys was still underway (Mann–Whitney U test: comparison with summer: U = 40.4, P < 0.0001; comparison with winter: U = 11.6, P < 0.001). Further individuals arrived south of the Sahara (8 out of 26 recoveries), and none was observed remaining in the ringing area.
In August, most 3+ females were recovered in the ringing area as in summer (Mann–Whitney U test: comparison with summer: U = 0.4, P = 0.54; comparison with winter: U = 6.2, P < 0.05). While in September, the distance to ringing sites of 3+ females was larger than that in summer (Mann–Whitney U test: U = 8.8, P < 0.01), and similar to that in winter (Mann–Whitney U test, U = 1.8, P = 0.18). There was no recovery of 3+ female in October.
In August, the majority of 3+ males still remained in the ringing area as in summer (Mann–Whitney U test: comparison with summer: U = 3.5, P = 0.06; comparison with winter: U = 20.9, P < 0.0001). The migration of 3+ males was underway in September (Mann–Whitney U test: comparison with summer: U = 7.4, P < 0.01; comparison with winter: U = 15.5, P < 0.0001), though most recoveries were still in the ringing area. There were only two recoveries of 3+ males in October, and both recovery sites were in southern Europe.
Winter
In winter, the 3+ males were closest to the ringing sites, while no difference in the distance to ringing sites was detected between 1Y, 2Y and 3+ females (Fig. 2c; Table 2). Using the Sahara as the boundary, the composition of Osprey populations wintering in the north and in the south differed (Chi-square test, χ 2 = 12.73, df = 3, P < 0.01). Of the 36 recovery reports in Europe and in Africa north of the Sahara, the percentage of 1Y, 2Y, 3+ females and 3+ males were 50, 17, 11 and 22%, respectively. While in the region south of the Sahara (n = 34), 1Y, 2Y and 3+ female,s respectively, made up 85, 12 and 3% of the population. No 3+ male was observed wintering south of the Sahara.
Spring
In spring, the 1Y Ospreys were recovered furthest from the ringing sites compared to the other three groups (Fig. 2d; Table 2). The spring distance to ringing sites of 1Y birds showed no monthly variation (Kruskal–Wallis test, H 2 = 4.8, P = 0.09), and did not differ from that in winter or that in their following (2Y) summer (Kruskal–Wallis test, H 2 = 3.1, P = 0.20).
Among the other three groups, the distance to ringing sites of 2Y Ospreys was greater than that of 3+ females, which was further greater than that of 3+ males (Fig. 3b). The 2Y Ospreys gradually approached the ringing area from March to May (Fig. 3b). The number of recoveries of 2Y birds in March (n = 3) was too small to analyse. In both April and May, the 2Y birds were closer to their ringing sites than in winter (Mann–Whitney U test: April: U = 11.1, P < 0.001; May: U = 13.3, P < 0.001) and more distant than in summer (Mann–Whitney U test: April: U = 17.3, P < 0.0001; May: U = 14.5, P < 0.001).
The migration of 3+ females was underway in March (Mann–Whitney U test: comparison with summer, U = 10.5, P < 0.01; comparison with winter: U = 6.4, P < 0.05). Since April, the distances to ringing sites of 3+ females showed no difference from that in summer (Mann–Whitney U test: April: U = 3.5, P = 0.06; May: U = 0.0, P = 0.99).
In March, the majority of 3+ males were already discovered in the ringing area as in summer (Mann–Whitney U test: U = 1.4, P = 0.23). Their distances to ringing sites in May also showed no difference from that in summer (Mann–Whitney U test: U = 0.0, P = 0.98). In April, however, the 3+ males were recovered closer to their ringing sites than in summer (median of the distance to ringing sites 20.8 km in April and 23.6 km in summer; Mann–Whitney U test: U = 4.4, P < 0.05).
Discussion
Migration routes and wintering sites
The migration routes of the German Osprey population form a broad front between south and southwest of the ringing area. The only two autumn recoveries in south-easterly directions were both dead 1Y birds, which probably reflect an exceptional orientation of juveniles unfavourable for central European Ospreys. Among Swedish and Finnish Ospreys, a minor proportion is known to have a south-eastern orientation (Fransson and Pettersson 2001; Saurola 1994; Thorup et al. 2003). There is no apparent discrepancy between the migration routes in autumn and in spring of the study population. Note that this suggestion applies to the population level and is a pooled pattern of data over two decades. When looking at single birds tracked by satellite, individual routes may show marked differences between seasons (Alerstam et al. 2006).
The study population winters in West Africa south of the Sahara and around the western Mediterranean. As mentioned earlier, the map of the recovery sites in Fig. 1 demonstrates the known range of the wintering grounds. It should not be interpreted as the representation of population distribution, as it was masked by recovery rate (Strandberg et al. 2009). The proportion of the population wintering in Africa should be much more than that indicated by the dots on the map, as the recoveries in Africa are under-represented relative to Europe. Similarly, the local concentration of recoveries in Gibraltar and The Gambia should be at least partly due to the popular bird-watching activity there. Nevertheless, the migration routes and wintering areas of the German Osprey population are generally consistent with those of the Osprey populations breeding in Scotland (Dennis 2008) and Fennoscandia (Fransson and Pettersson 2001; Saurola 1994; Thorup et al. 2003). These results are also in agreement with the findings of an earlier investigation on the same population (Schmidt and Roepke 2001).
From the few multiple recoveries of same individuals in one winter, we suggest that individual Ospreys probably stay in a confined area instead of being nomadic in winter. And they are also likely to return to the same wintering ground in different years. This was supported by detailed documentation for one individual that wintered in Sardinia in the same lagoon for several consecutive years (Loi and Pisano 2006). The satellite-tracking of Ospreys breeding in Sweden also found that they are highly faithful to their wintering sites between years and have small home ranges in winter (Alerstam et al. 2006; Hake et al. 2001; R. Strandberg, personal communication).
Differential migration of the Osprey
Our analyses demonstrate the differential migration of central European Ospreys in both spring and autumn timings as well as in wintering grounds. In spring, the 3+ males return earliest to the breeding area, followed by 3+ females and then 2Y birds. The fact that no Osprey was recovered in the ringing area in February, and that the 3+ males already arrive at their summer locations in March, indicates that the migration of 3+ male Ospreys is concentrated in late February and early March. The spring migration of 3+ females is underway in March and completed in April. The migration of 2Y Ospreys starts in March and covers a longer time-span to finish in June. In Sweden, the limited number of individuals satellite-tracked in spring did not reveal a sexual difference in arrival time. But field observations also suggested that most males arrive earlier than females (M. Hake and R. Strandberg, personal communication).
In addition, we found that the April distance to ringing sites of 3+ males is closer than that in summer. This might reflect on one hand the high intensity of colour-ring reading by nest wardens at nest sites early in the breeding season, and on the other hand a behavioural change of male Ospreys. Nest building and courtship of Ospreys takes place mainly in April, and therefore it is likely that relatively more Ospreys are recovered at their nest sites in April. Later in the season, male Ospreys spend more time foraging at lakes which are often several kilometres away from their nest sites. Therefore, recovery sites of male Ospreys in summer can differ from those in April. Such difference was not detected in females, which remain most of the time at the nest sites until late in the breeding season.
In autumn, the 3+ females migrate earlier than 3+ males, while the migration of 1Y Ospreys takes place over a wider time-span. The 3+ females were recovered at their summer locations in August and at their winter locations in September, which suggests that their migration may concentrate between late August and the beginning of September. The migration of 3+ males is underway in September and probably finishes in October. The pattern that adult females leave the breeding area earlier than adult males was also observed in the Osprey populations in Sweden (Hake et al. 2001; Kjellén et al. 2001) and in the United States (Martell et al. 2001) via satellite telemetry. For 1Y Ospreys, the timing of autumn migration shows large variation among individuals. Some 1Y birds have already arrived in the wintering grounds in August, while some are still close to the ringing area in October. Satellite tracking in Sweden also found that many 1Y birds had prolonged stays in specific foraging areas before setting out on autumn migration (R. Strandberg, personal communication).
In winter, the 3+ males stay closer to the ringing area than females and younger birds. These older male Ospreys probably do not winter south of the Sahara as often as do other groups. This view is supported by Dennis (2008) who noted that Ospreys wintering in the Iberian Peninsula show a high proportion of males. In North America, adult female Ospreys were also found to travel farther than adult males (Martell et al. 2001). However, both adult males and adult females satellite-tracked in Sweden wintered in West Africa (Alerstam et al. 2006; Hake et al. 2001). Further study is necessary to investigate if Osprey populations in central Europe and Fennoscandia adopt different migratory strategies.
Mechanisms for differential migration in Osprey
The sexual difference in the timing of spring migration and in the choice of wintering grounds in Ospreys is consistent with the arrival-time hypothesis and does not support the body-size hypothesis. Therefore, males being selected for prior access to breeding territories (Ketterson and Nolan 1983; Myers 1981) is the most likely explanation for 3+ males wintering closer to the breeding area and returning earlier in spring than females.
The difference in wintering grounds between Ospreys of different age classes refuted the migration-constraints hypothesis, as young birds do not winter closer to the breeding area than older ones. The observed phenomenon partly agrees with the dominance hypothesis, as 1Y and 2Y Ospreys migrate further than 3+ males. However, no further differences between wintering grounds of 1Y, 2Y and 3+ female Ospreys can be confirmed in the current data. Ospreys are known to share foraging sites. Although Prevost (1982) reported that Ospreys defend winter territories, this acts only on a small spatial scale. The mechanism that food competition drives differential migration as suggested by the dominance hypothesis is less likely to apply to Ospreys.
We suggest that the difference in choosing wintering grounds between Ospreys of different age classes is also generated by the same mechanism as proposed by the arrival-time hypothesis. The difference arises due to the fact that the 3+ males, the potential breeders of the following years, are selected to winter closer for earlier arrival (arrival-time hypothesis), not that the young birds are driven further (dominance hypothesis). It has also been demonstrated by theoretic models that birds with high opportunity to breed are subject to strong selection of early arrival, because they have “the most to lose” if falling behind (Kokko 1999).
In autumn, female Ospreys leave the breeding area earlier than males, which can be explained by both the rank-advantage hypothesis and the mate-opportunity hypothesis (Mills 2005). The current data could not tell the two hypotheses apart, but they both suggest that male Ospreys may increase their breeding success for the subsequent year by remaining longer in the breeding area. The foraging efficiency hypothesis is not supported, as 1Y Ospreys do not in general leave earlier. In summary, we suggest that the competition for breeding resources shaped the patterns of differential migration in Ospreys, including the differences in the timing of spring and autumn migrations and the differences in wintering grounds.
References
Alerstam T, Hake M, Kjellén N (2006) Temporal and spatial patterns of repeated migratory journeys by ospreys. Anim Behav 71:555–566
Bai ML, Schmidt D, Gottschalk E, Mühlenberg M (2009) Distribution pattern of an expanding Osprey (Pandion haliaetus) population in a changing environment. J Ornithol 150:255–263
Berthold P (2001) Bird migration. A general survey. Oxford University Press, Oxford
Bildstein K (2006) Migrating raptors of the world—their ecology and conservation. Cornell University Press, London
Cristol DA, Baker MB, Carbone C (1999) Differential migration revisited: latitudinal segregation by age and sex class. Curr Ornithol 15:33–88
DeLong J, Hoffman SW (1999) Differential autumn migration of sharp-shinned and Cooper’s hawks in western North America. Condor 101:674–678
Dennis R (2008) A life of ospreys. Whittles, Caithness
Evans DM, Day KR (2001) Migration patterns and sex ratios of diving ducks wintering in Northern Ireland with specific reference to Lough Neagh. Ringing Migr 20:358–363
Fernandez G, Lank DB (2007) Variation in the wing morphology of Western Sandpipers (Calidris mauri) in relation to sex, age class, and annual cycle. Auk 124:1037–1046
Fransson T, Pettersson J (2001) Swedish bird ringing atlas vol. 1. Divers—raptors. Swedish museum of natural history, Stockholm
Gauthreaux SA (1982) The ecology and evolution of avian migration systems. Avian Biol 6:93–168
Gillis EA, Green DJ, Middleton HA, Morrissey CA (2008) Life history correlates of alternative migratory strategies in American dippers. Ecology 89:1687–1695
Guillemain M, Hearn R, King R, Gauthier-Clerc M, Simon G, Caizergues A (2009) Differential migration of the sexes cannot be explained by the body size hypothesis in Teal. J Ornithol 150:685–689
Hake M, Kjellén N, Alerstam T (2001) Satellite tracking of Swedish ospreys Pandion haliaetus: autumn migration routes and orientation. J Avian Biol 32:47–56
Jenkins KD, Cristol DA (2002) Evidence of differential migration by sex in white-throated sparrows (Zonotrichia albicollis). Auk 119:539–543
Kerlinger P (1989) Flight strategies of migrating hawks. University of Chicago press, Chicago
Ketterson ED, Nolan V Jr (1976) Geographic variation and its climatic correlates in the sex ratio of eastern-wintering dark-eyed juncos (Junco hyemalis hyemalis). Ecology 57:679–693
Ketterson ED, Nolan V Jr (1983) The evolution of differential bird migration. Curr Ornithol 1:357–402
Kjellén N, Hake M, Alerstam T (2001) Timing and speed of migration in male, female and juvenile ospreys Pandion haliaetus between Sweden and Africa as revealed by field observations, radar and satellite tracking. J Avian Biol 32:57–67
Kokko H (1999) Competition for early arrival in migratory birds. J Anim Ecol 68:940–950
Loi V, Pisano S (2006) Svernamento di Falco pescatore Pandion haliaetus di origine tedesca in Sardegna. Alula 13:201–203
Lyons JE, Collazo JA, Guglielmo CG (2008) Plasma metabolites and migration physiology of semipalmated sandpipers: refueling performance at five latitudes. Oecologia 155:417–427
Martell MS, Henny CJ, Nye PE, Solensky MJ (2001) Fall migration routes, timing, and wintering sites of North American ospreys as determined by satellite telemetry. Condor 103:715–724
Mazerolle DF, Hobson KA (2007) Patterns of differential migration in white-throated sparrows evaluated with isotopic measurements of feathers. Can J Zool 85:413–420
Mebs T, Schmidt D (2006) Die Greifvögel Europas, Nordafrikas und Vorderasiens—Biologie, Kennzeichen, Bestände. Kosmos, Stuttgart
Mills AM (2005) Protogyny in autumn migration: do male birds ‘play chicken’? Auk 122:71–81
Morris SR, Pusateri CR, Battaglia KA (2003) Spring migration and stopover ecology of common yellowthroats on Appledore Island, Maine. Wilson Bull 115:64–72
Morton ML (1984) Sex and age ratios in wintering white-crowned sparrows. Condor 86:85–87
Myers JP (1981) A test of three hypotheses for latitudinal segregation of the sexes in wintering birds. Can J Zool 59:1527–1534
Nilsen R, Gutvik OK, Nilssen EM, Hopkins CCE (1989) Population parameters of the witch flounder Glyptocephalus cynoglossus (L.) (Pisces: Pleuronectidae) from Malangen, northern Norway. Fish Res 12:259–278
O’Hara PD, Fernandez G, Haase B, de la Cueva H, Lank DB (2006) Differential migration in western sandpipers with respect to body size and wing length. Condor 108:225–232
Payevsky VA (1995) Differential wintering and survival rates of age and sex groups in some species of finches. Zool Zh 74:129–135
Poole AF (1989) Osprey: a natural and unnatural history. Cambridge University Press, Cambridge
Prescott DRC, Middleton ALA (1990) Age and sex differences in winter distribution of American goldfinches in eastern North America. Ornis Scanda 21:99–104
Prevost YA (1982) The wintering ecology of ospreys in Senegambia. Dissertation, University of Edinburgh
Roepke D (1996) Fünfzehn Jahre Beringungsarbeit am Fischadler (Pandion. h. haliaetus). Naturschutzarb Mecklenburg-Vorpommern 39:22–35
Rogers CM, Theimer TL, Nolan V Jr, Ketterson ED (1989) Does dominance determine how far dark-eyed juncos, Junco hyemalis, migrate into their winter range? Anim Behav 37:498–506
Saurola P (1994) African non-breeding areas of Fennoscandian ospreys Pandion haliaetus: a ring recovery analysis. Ostrich 65:127–136
Schmidt D (1995) Beringung nestjunger Fischadler Pandion haliaetus in Ostdeutschland mit Farbkennringen. Ber Vogelw Hiddensee 12:133–136
Schmidt D (2009) 15 Jahre Farbberingung von Fischadlern Pandion haliaetus in Deutschland. Ber Vogelw Hiddensee 19:47–52
Schmidt D (2010) Der Brutbestand des Fischadlers Pandion haliaetus in Deutschland im frühen 21. Jahrhundert. Charadrius 46:10–17
Schmidt D, Meyburg B-U (1998) Forschung an Fischadlern (Pandion haliaetus) im strelitzer land. Labus (Neustrelitz). Neue Reihe 8:22–27
Schmidt D, Roepke D (2001) Zugrouten und Überwinterungsgebiete von in Deutschland beringten Fischadlern Pandion haliaetus. Vogelwelt 122:141–146
Schmidt D, Rochus Chr, Jackson F (2000) Methoden zur Bestimmung der Körpermasse von Fischadlern Pandion haliaetus. Populationsökol Greifvogel Eulenarten 4:143–152
Schmidt D, Herold S, Lange H, Reusse P (2006) Zur Philopatrie des Fischadlers Pandion haliaetus in Deutschland—Zwischenergebnisse des Farbringprogramms 1995–2004. Populationsökol Greifvogel Eulenarten 5:133–142
Stewart BS (1997) Ontogeny of differential migration and sexual segregation in northern elephant seals. J Mammal 78:1101–1116
Stewart RLM, Francis CM, Massey C (2002) Age-related differential timing of spring migration within sexes in passerines. Wilson Bull 114:264–271
Strandberg R, Klaassen RHG, Thorup K (2009) Spatio-temporal distribution of migrating raptors: a comparison of ringing and satellite tracking. J Avian Biol 40:500–510
Thorup K, Alerstam T, Hake M, Kjellén N (2003) Can vector summation describe the orientation system of juvenile ospreys and honey buzzards?—An analysis of ring recoveries and satellite tacking. Oikos 103:350–359
Weber M, Schmidt D, Haedrich J (2003) Chlororganische Rückstände in Eiern des Fischadlers (Pandion haliaetus) aus Deutschland. J Ornithol 144:45–58
Woodrey MS, Chadler CR (1997) Age-related timing of migration: geographic and interspecific patterns. Wilson Bull 109:52–67
Acknowledgments
We are grateful to Dr. R Strandberg for sharing his expert knowledge which has enriched the manuscript. We thank especially all the volunteer ringers and their teams who have worked hard for many years to make this publication possible: Dr. M. Dornbusch, H. Gabriel, P. Hauff, S. Herold, Dr. P. Kneis, W. Köhler, M. Kuhnert, G. Lohmann, Dr. R. Möckel, P. Reuße, D. Roepke, Ch. Scharnweber and P. Sömmer. We are also grateful to those who reported sightings of ringed Ospreys. The following nest wardens and coordinators made it possible that D. Schmidt could ring many Osprey nestlings: M. Dittrich, B. Ewert, M. Graef, E. Hemke, B. Heuer, H. Lange, O. Manowsky, K. Ridder, G. Röber und D. Ruhle. R. Wahl provided the information about the Ospreys breeding in France. H. Dansberg produced the coded colour-rings and ringing instruments. The Forestry and Nature Conservation authorities, the Hiddensee Bird Ringing Centre, the power companies enviaM, E.ON edis and Vattenfall Europe Transmission and the DB Energie GmbH supported the ringing. Most of the work of D. Schmidt was funded by the Adler Modemärkte Haibach and the Deutsche Ornithologen-Gesellschaft (DO-G). This study complies with the current laws in Germany.
Author information
Authors and Affiliations
Corresponding author
Additional information
Communicated by F. Bairlein.
This is the ring recovery report no. 4/2011 of the Hiddensee Bird Ringing Centre.
Rights and permissions
About this article
Cite this article
Bai, ML., Schmidt, D. Differential migration by age and sex in central European Ospreys Pandion haliaetus . J Ornithol 153, 75–84 (2012). https://doi.org/10.1007/s10336-011-0697-y
Received:
Revised:
Accepted:
Published:
Issue Date:
DOI: https://doi.org/10.1007/s10336-011-0697-y