Abstract
Generic equations are proposed for stem, branch and foliage biomass of individual trees in even-aged pure stands of Cryptomeria japonica, Chamaecyparis obtusa and Larix kaempferi. Biomass data was collected from a total of 1,016 individual trees from 247 stands throughout Japan, and five regression models were assessed by root mean square error, mean bias, fit index (FI), and AIC. The results show that a power equation using diameter at breast height (dbh) and height is the most suitable for all species and components. This equation is more accurate than the familiar power equation that uses ‘dbh2 height’, and it expresses the greater volume of branch and foliage mass of trees with a lower height/diameter ratio. A power equation using dbh is more reasonable for models with dbh as the only independent variable and more accurate than a power equation using ‘dbh2 height’ for estimating branch and foliage mass. Estimating error for branch and foliage mass is larger than that for stem mass, but the entire aboveground biomass can be estimated with an error of less than 19%, except in the case of small trees with dbh less than 10 cm.
Similar content being viewed by others
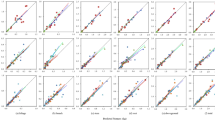
Avoid common mistakes on your manuscript.
Introduction
In view of the impact of global warming, particular attention is directed towards absorption and fixation of carbon dioxide by forests and the use of forest resources as an alternative to fossil fuels. On this account, the necessity increases for expressing forest stock and growth not only as stem volume but also as weight of forest stand biomass that includes branches, foliage and roots. Cases in which the results of field experiments or yield prediction must be expressed in both volume and biomass or carbon are increasing for forest researchers (e.g., Nilsen and Strand 2008).
In the accounting rules under the Kyoto Protocol, forest carbon sinks are calculated by converting stand stem volume into carbon stock by multiplying the ratio of aboveground biomass to stem biomass (biomass expansion factor, BEF), the ratio of entire above- and underground biomass to aboveground biomass, basic wood density, and carbon fraction (IPCC 2003). In Japan, active investigation to determine these coefficients resulted in obtaining BEFs according to forest species and age class (Greenhouse Gas Inventory Office of Japan; Ministry of the Environment of Japan 2008). As academic research, Fukuda et al. (2003) also used age-dependent BEFs by species for a stand volume model by region to estimate the national carbon stock of Japanese cedar (Cryptomeria japonica) and Japanese cypress (Chamaecyparis obtusa) in Japan.
These BEFs were determined at the stand level, originally for the purpose of calculating carbon stock using national or regional forest volume statistics from forest registers or the National Forest Inventory. A constant BEF can be selected by species and forest age, but individual diameter and height as well as size distribution of stands are not considered. This BEF approach at the stand level is not suitable for evaluating the effects of forest management on carbon sinks due to the absence of information on individual trees and stand structure. In this case, a biomass equation at the individual tree level is an alternative approach (Somogyi et al. 2007). This biomass equation approach is also a traditional allometric method in ecological studies. However, the destructive sampling required to develop the biomass equation involves extensive labor, time and cost.
Although the majority of published biomass equations are site-specific estimations using sample trees in each study site, detailed reviews of tree biomass equations have been reported by a number of developed countries, and common equations on a national or regional scale have since been proposed. Examples of equations have also been compiled into a database for wide distribution via the Internet (Eamus et al. 2000; Jenkins et al. 2003; Zianis et al. 2005). In Japan, on the other hand, the only similar example is from Tanouchi and Utsugi (2004) who reported an average allometric coefficient by rearranging a published power equation with ‘dbh2 height’. A generic nationwide biomass equation has not yet been proposed in Japan. Such a national scale equation is valuable for evaluating of carbon stock and the effects of forest management at the stand level by a common method.
Incidentally, diameter and height two-way stem volume equations for major species by region are available in Japan. The majority of these volume equations were accurately developed using data from numerous individual trees in the 1950s and 1960s, but branch, foliage and basic wood density data were not recorded, making it impossible to estimate their amount using the same dataset. Biomass equations estimate component biomass directly without basic wood density. We attempted to develop each component biomass equation using new datasets.
Therefore, the objective of this study was to propose an equation for estimating stem, branch or foliage biomass at the individual tree level for an even-aged pure stand of Japanese cedar, Japanese cypress and Japanese larch (Larix kaempferi) as the major plantation species in Japan, by using destructive sampling data collected from throughout Japan during forest carbon sink projects under the Forestry Agency of Japan and other parties. The data used in this study, as well as data pertaining to other species, will be published in the near future.
Materials and methods
Data
We used individual tree biomass data on Japanese cedar (Cryptomeria japonica), Japanese cypress (Chamaecyparis obtusa) and Japanese larch (Larix kaempferi) collected during the course of the following projects: (1) Project for Evaluating the Effects of Thinning on Forest Sinks (Forestry Agency of Japan, FY1999–2000); (2) Project for Developing a System for Measuring Forest Sinks (Forestry Agency of Japan, FY2001–2002); (3) Project for the Institutional Development of a System for Measuring and Using Forest Sinks (Forestry Agency of Japan, FY2003–2006); and (4) Study on Transparent and Verifiable Method of Evaluating Carbon Sinks (Forestry and Forest Products Research Institute (FFPRI), FY2001–2003, funded by the Ministry of the Environment of Japan). Projects (1)–(3) were implemented mainly for non-national forests. Prefectural forestry research institutes selected the sample stands and trees, carried out the stand inventory, destructive sampling of trees and drying/weighing of samples, and FFPRI was in charge of the master investigation plan and data summary. The object of (4) was a national forest, and the majority of investigated stands are the growth and yield experimental sites managed jointly by Regional Forest Offices and FFPRI.
The investigations were conducted by following the same manual. In the case of conifers, the method for measuring tree biomass was as follows. From among the trees with average branches and foliage, one dominant tree, two intermediate-size trees (slightly larger or slightly smaller than the average diameter) and one suppressed tree were selected. These four trees were measured for diameter at breast height (dbh) and tree height, and then cut down. Felled trees were crosscut at 1-m intervals. Each log was then divided into stem, living branches, and dead branches, which were each weighed. About three main living branches were chosen from each log, and separated into branches, foliage, dead branches, and dead foliage which was remained on living or dead branches. Then, the weight of each was measured. Green or mixed green/brown parts of fine branches were included in the foliage in this procedure. A sample of about 300 g was collected from the branches, foliage, and dead branches and foliage of each log, and a disc from the stem about 5 cm thick was cut from the bottom end of each log. All samples and discs were brought to the laboratory, and weighed after oven-drying. Total fresh and dry weight of all samples was converted to whole tree dry weight of stems, branches, foliage, and dead branches and foliage by proportion calculation.
Biomass data was obtained on four individual trees per stand (in Project 1, five or more trees were often measured) as described above, and 20 × 20 m stand inventory data was also used (Table 1). However, nine individuals were rejected due to deviation from the general trend shown by all three species in the relationship between dbh and height, stem mass, branch mass, foliage mass, and dead branch and foliage mass of individual trees. Seven stands were excluded from the analysis of stand data due to deviation from the general trend in relationship between forest age and mean height, mean dbh, number of stems, and total basal area of stands. Dead branches and foliage were not estimated in the present study.
Statistical analysis
The following five representative regression models were chosen from among Western and Japanese studies for practical application in estimating individual stem, branch or foliage mass.
where W is stem, branch or foliage mass (kg), D is dbh (cm), H is height of a tree (m), and a–c are coefficients.
Eamus et al. (2000) proposed a generalized biomass equation using actual survey data on numerous stands, and Jenkins et al. (2003) and Muukkonen (2007) presented one obtained by meta-analysis of published equations. They then used Eq. 1 or its logarithmic form. Muukkonen (2007) also used Eq. 2 and reported that it was preferable to Eq. 1, particularly in the case of small trees. Equation 3 is the most typical allometric equation (Kira and Shidei 1967) and was used in almost all previous studies in Japan. Equation 4, a stem volume equation referred to as the Yamamoto–Schumacher equation (Yamamoto 1918; Schumacher and Hall 1933), is adopted for most stem volume tables used in Japan, and thus a good fit is expected for stem mass. Equation 5, also a stem volume equation, can be referred to as Takada’s equation and is reported to be the best for Japanese cedar among the various stem volume equations, including Eq. 4 (Nagumo and Minowa 1990).
Like later descriptions, Eq. 4 was not precise relatively for branch and foliage mass of Japanese larch described, we tried adding explanatory variables to influence the tree shape, such as age, relative spacing, ratio of height to dbh of individual tree, relative dbh (ratio of individual dbh to mean dbh of the stand), relative height (ratio of individual height to mean height of the stand), mean annual increment of individual height.
A nonlinear least-squares method was used for all models since log-transformation bias correction is not necessary. Component biomass varied a great deal, so tree size variation was large (Fig. 1), and it was clear that homogeneity of dispersion was low. Thus, regression equations for estimating standard deviation, s, of component biomass from dbh were computed (Table 2), and the weighted nonlinear least-squares method with a weight of 1/s 2 (Okuno 1992) was adopted. The nls function in R software Version 2.6.2 (R Development Core Team 2008) was used for fitting via the Gauss–Newton method and the weight option.
For evaluation indexes for model selection, we used root mean square error (RMSE), mean bias, fit index (FI) and Akaike’s information criterion (AIC). RMSE, mean bias, FI and AIC (Suzuki 1995) are expressed in Eqs. 6–9. In addition, percentage RMSE expressed in Eq. 10 is used to indicate expected estimation error.
where y i , \( \overset{\lower0.5em\hbox{$\smash{\scriptscriptstyle\frown}$}}{y}_{i} \), \( \bar{y} \), n and p are observed biomass, estimated biomass, average observed biomass, sample size, and number of parameters, respectively. RMSE is an index of precision mainly expressed by estimating error. Mean bias is an index of unbiasedness of estimation. FI is analogous to the coefficient of determination in ordinary linear regression, and although it might not reach the 0–1 range, it is an index with few weak points in comparison with other similar indexes (Kvålseth 1985). Equations 6–10 were calculated without using the 1/s 2 weight above.
Results
Difference by species in the relationship between tree size and biomass
As the size of an individual tree increases, it is natural for the stem, branch and foliage mass to increase at the same time, and so does the variation of these values. However, the degree of increase differed by species (Fig. 1). When the dbh was more than 20 cm, the stem mass of Japanese larch tended to be greater than the stem mass of Japanese cedar and Japanese cypress. The branch mass of Japanese cedar was less than the branch mass of Japanese cypress and Japanese larch for almost any dbh. By contrast, foliage mass appeared in the order of Japanese larch < Japanese cypress < Japanese cedar. Foliage mass of Japanese larch as a deciduous tree was only about 1/3 that of Japanese cedar and about 1/2 that of Japanese cypress of the same size. The above results clearly demonstrate the difference in size–mass relationship by species. Therefore, we conducted our analysis according to species as follows.
Stem mass
Each evaluation index is shown in Table 3 according to species, component, and regression model. In the case of stem mass of Japanese cedar and Japanese cypress, RMSE was the smallest, mean bias was near zero, and FI was the highest in Eq. 4. In Japanese larch, RMSE and FI in Eq. 4 were approximately equal to those in Eq. 5, but mean bias was closer to zero in Eq. 4. When Eqs. 1 and 2, using dbh as the only explanatory variable, were compared, Eq. 1 was more precise and less biased for Japanese cedar. In Japanese cypress and Japanese larch, ‘good or bad’ was not the same for each evaluation index, but the indexes of Eqs. 1 and 2 were approximately equal. AIC of Eqs. 3–5, in which both dbh and height of a tree are the variables, was smaller than that in Eqs. 1 and 2. Even if the number of parameters were increased, it was judged that Eqs. 3–5 would be more suitable models. FI was better than 0.9 in all species and models. All equations generally fit well.
Branch mass
Equation 4 was the most precise and least biased for Japanese cedar, Japanese cypress and Japanese larch combined, and also, AIC was the smallest. In comparing Eqs. 1 and 2, RMSE and FI were slightly superior in Eq. 2 for all species. However, in Eq. 2 for Japanese larch, significance of regression coefficients a and c was low (p > 0.05). Mean bias in Eqs. 1 and 2 was the same, or Eq. 1 was slightly closer to zero, for all three species. RMSE and AIC in Eqs. 1 and 2 were smaller than in Eq. 3 normally used as the allometric equation in Japan. Even FI in Eq. 4 at the best was 0.72–0.83, with low precision when compared to stem mass.
In Eq. 4 for Japanese larch, where the error was relatively large, we tried adding explanatory variables to influence the tree shape. When ‘relative height’ was added, RMSE, FI and AIC improved slightly, but RMSE became 6.53 and FI became 0.747 at most. Additional variables did not greatly improve the model.
Foliage mass
Equation 4 was the most precise and least biased for all species, the same as for branch mass, and also, AIC was the smallest. However, mean bias in Eq. 4 reached zero later than in Eq. 3 for Japanese cypress. When Eqs. 1 and 2 were compared for Japanese cedar and Japanese cypress, RMSE, FI and AIC in Eq. 2 were slightly better, but mean bias in Eq. 1 was closer to zero. In Japanese larch, FI in Eq. 1 was a negative value, and FI in Eq. 2 was 0.303, not at all precise. Moreover, significance of regression coefficients a and c in Eq. 2 was low (p > 0.05). FI in Eq. 4 was 0.37–0.71, and precision was still lower than for branch mass.
As in the case of branch mass, the addition of a variable was attempted in Eq. 4 for Japanese larch. When ‘relative height’ was added, the evaluation criterion improved, but there were also a few other effects, and RMSE became 2.75 and FI became 0.388 at most.
Discussion
As described earlier, it is generally assumed that forest biomass investigation requires destructive sampling and allometric equations for each stand. However, in the case of stem mass, the difference in allometric equation by stand can be small if dbh2 height is used as the explanatory variable, and there are reports that its expression of the best allometric equation can be more or less common by species (Yoda 1971; Migita et al. 2001; Tanouchi et al. 2004). In the present study, the application of Eqs. 3–5 to stem mass using dbh and height as explanatory variables achieved sufficient accuracy.
A regression coefficient of Eq. 3 (Table 4) was compared with the average coefficients of Japanese cedar and Japanese cypress obtained by Tanouchi and Utsugi (2004) using rearranged published equations. It cannot be said that these coefficients are one and the same, but there are many points of similarity. That is, coefficient a for stem mass is in the order of Japanese cypress < Japanese cedar, and coefficient b is vice versa. Coefficient a for branch mass is in the order of Japanese cedar < Japanese cypress, and coefficient b is the reverse. Coefficient b for stem mass is close to 1, but coefficient b for foliage mass is about 0.5.
For stem mass, the difference in precision and unbiasedness between Eqs. 3 and 5 is not particularly large in the case of Japanese cypress and Japanese larch. However, RMSE in Eq. 4 for Japanese cedar is small, mean bias in Eq. 4 for all species is close to zero, and Eq. 4 is widely used as a stem volume equation, but the significance of coefficient b in Eq. 5 for Japanese larch is low. Thus, Eq. 4 should be chosen when both dbh and height are used as explanatory variables. In equations with dbh as the only explanatory variable, Eq. 1 should be adopted for stem mass, because RMSE in Eq. 1 for Japanese cedar was small, although there was no great difference in RMSE between Eqs. 1 and 2 for Japanese cypress and Japanese larch.
For branch and foliage mass, Eq. 4 was the best for all three species. Additionally, RMSE in Eq. 4 was 17–26% smaller than in Eq. 3 and FI in Eq. 4 was 0.07–0.47 higher than in Eq. 3 commonly used in Japan. So, Eq. 4 is effective when both dbh and height can be used as explanatory variables. However, even for Eq. 4, the expected precision was slightly low when compared with the case of stem mass. In the two equations using only dbh, Eq. 1 is also better for branch and foliage mass because there is little difference in precision and unbiasedness, and Eq. 1 has a simpler expression. However, for foliage mass of Japanese larch, Eqs. 1 and 2 must not be adopted judging from the low precision and significance of regression coefficient.
When the best equation, Eq. 4, was used, the expected accuracy of both foliage and branch mass was much lower than that for stem mass, and the error may have been greater than 50% (Table 5). According to previous studies, the difference in allometric equations by stand can be reduced if the diameter at just below the lowest living branch is used as an explanatory variable for branch and foliage mass (Yoda 1971; Sato 1973; Komiyama 1999). However, since the measurement of an upper diameter requires considerable time and labor, and most aboveground biomass is stem, large estimation errors in branch and foliage mass are unavoidable in practical application. The estimation error for total aboveground biomass that includes all components is less than 19% in the case of Eq. 4, except for small trees with dbh less than 10 cm (Table 5).
It should be noted that the power coefficient for height is a negative number in Eq. 4 for branch and foliage mass for each of the three species. This means that a taller tree has fewer branches and less foliage even if the dbh is the same. Such phenomenon is hard to be imaged when observing the difference between individual trees in a forest stand. However, as shown in Fig. 2, it means that trees with a lower height/diameter ratio have more branches and foliage even if the height is the same. In other words, a dominant tree or a tree in a relatively low-density stand has more branches and foliage. Therefore, it is rational that the equation should apply not only to a specific stand but also to individual trees of varying age and stand density. Peichl and Arain (2007) reported an allometric equation for Pinus strobus, and described Eq. 1 as being adequate for estimating the entire individual biomass, but to estimate the component biomass of individuals, the forest age or tree height should be added as a second variable. Also, they obtained a negative value for the power coefficient in Eq. 4 or one that replaced height with age for branch and foliage mass. This is in agreement with the results of the present study. In the case of a temporary investigation of a specific stand, or the necessity to avoid the contradiction that a taller tree has fewer branches and foliage even when the diameter is the same, it is time to use Eq. 3, not Eq. 4.
From the above discussion, we concluded that it is appropriate to adopt Eq. 4 when both dbh and height can be used, and Eq. 1 when dbh is used as the only explanatory variable, for each component and species, except for foliage mass of Japanese larch. Equations according to components and species are shown in Table 4. The percentage RMSE according to diameter class is shown in Table 5. For comparison reference, Eq. 3 was also shown except for foliage mass of Japanese larch due to remarkably low precision. Care should be taken to ensure that the object of these equations is an individual tree in an even-aged pure stand, and extrapolation is not recommended beyond the range of stand statistics, individual tree size and region shown in Table 1.
Notes
Titles of “in Japanese” articles are our tentative translation from the original Japanese title.
References
Titles of “in Japanese” articles are our tentative translation from the original Japanese title.
Eamus D, McGuinness K, Burrows W (2000) Review of allometric relationships for estimating woody biomass for Queensland, the Northern Territory and Western Australia, National Carbon Accounting System Technical Report 5a. Australian Greenhouse Office, Canberra
Fukuda M, Iehara T, Matsumoto M (2003) Carbon stock estimates for sugi and hinoki forests in Japan. For Ecol Manage 184:1–16
IPCC (2003) Good practice guidance for land use, land-use change and forestry. IPCC National Greenhouse Gas Inventories Programme, Hayama
Jenkins JC, Chojnacky DC, Heath LS, Birdsey RA (2003) National-scale biomass estimators for United States tree species. For Sci 49:12–35
Kira T, Shidei T (1967) Primary production and turnover of organic matter in different forest ecosystems of the western Pacific. Jpn J Ecol 17:70–87
Komiyama A (1999) Measuring forest toward the future: Broad-leaved secondary forest. Shinrin-kagaku 27:41–43 (in Japanese)
Kvålseth TO (1985) Cautionary note about R 2. Am Stat 39:279–285
Migita C, Tanouchi H, Utsugi H, Nakamura T (2001) Analysis of factors to prescribe allometric coefficient using Japanese cypress and cedar. Trans Jpn For Soc 112:85 (in Japanese)
Ministry of the Environment of Japan (2008) National Greenhouse Gas Inventory Report of Japan, May 2008. National Institute for Environmental Studies, Tsukuba
Muukkonen P (2007) Generalized allometric volume and biomass equations for some tree species in Europe. Eur J For Res 126:157–166
Nagumo H, Minowa M (1990) Forest mensuration. Chikyusha, Tokyo (in Japanese)
Nilsen P, Strand LT (2008) Thinning intensity effects on carbon and nitrogen stores and fluxes in a Norway spruce (Picea abies (L.) Karst.) stand after 33 years. For Ecol Manage 256:201–208
Okuno T (ed) (1992) Applied statistics handbook. Yokendo, Tokyo (in Japanese)
Peichl M, Arain MA (2007) Allometry and partitioning of above- and below-ground tree biomass in an age-sequence of white pine forests. For Ecol Manage 253:68–80
R Development Core Team (2008) R: a language and environment for statistical computing. http://www.R-project.org
Sato T (1973) Material production of land plant community I a. Kyoritsu Shuppan, Tokyo (in Japanese)
Schumacher FX, Hall FDS (1933) Logarithmic expression of timber-tree volume. J Agric Res 47:719–734
Somogyi Z, Cienciala E, Mäkipää R, Muukkonen P, Lehtonen A, Weiss P (2007) Indirect methods of large-scale forest biomass estimation. Eur J For Res 126:197–207
Suzuki G (1995) Introduction to statistical analysis with information criterion. Kodansha Scientific, Tokyo (in Japanese)
Tanouchi H, Utsugi H (2004) National evaluation of forest site productivity considering growth environment characteristics. In: FFPRI Project Report 3: advancement of evaluation of the CO2 income and expenditure in forests and oceans. Forest and Forest Products Research Institute, Tsukuba, pp 24–28 (in Japanese)
Tanouchi H, Utsugi H, Shiono K (2004) Allometry of major tree species in Japan: can a common coefficient be found? Abstr Jpn For Soc Kanto Branch 56:31 (in Japanese)
Yamamoto W (1918) Stem volume and breast height form factor table for Pinus densiflora. Bull Imperial For Exp Stat Jpn 16:147–164 (in Japanese)
Yoda K (1971) Forest ecology. Tsukiji Shokan, Tokyo (in Japanese)
Zianis D, Muukkonen P, Mäkipää R, Mencuccini M (2005) Biomass and stem volume equations for tree species in Europe. Silva Fenn Monogr 4:1–63
Acknowledgments
We thank the many researchers at the prefectural forestry research institutes who greatly contribute to forest carbon sink projects. We also express our appreciation for the cooperation of private forest owners, Regional and District Forest Offices, and the Research, Extension, and Environment Policy Division of the Forestry Agency of Japan. Miki Fukuda, Satoshi Ishibashi, Tomohiro Nishizono, Hiroshi Kondo, Kunihiro Tanaka, Eiji Kodani (FFPRI), Iwao Noda (Japan International Research Center for Agricultural Sciences), Yoshio Awaya (Gifu University) and Masahiro Amano (Waseda University) collaborated with us on the national forest project. We also thank the anonymous reviewers for their careful reading of this article and insightful comments. Part of this study was supported by the Global Environment Research Coordination System of the Ministry of Environment of Japan as a research project on the Influence of Environmental Changes and Forest Management Practices on CO2 Sequestration of Conifer Plantations (FY2004–2008).
Author information
Authors and Affiliations
Corresponding author
About this article
Cite this article
Hosoda, K., Iehara, T. Aboveground biomass equations for individual trees of Cryptomeria japonica, Chamaecyparis obtusa and Larix kaempferi in Japan. J For Res 15, 299–306 (2010). https://doi.org/10.1007/s10310-010-0192-y
Received:
Accepted:
Published:
Issue Date:
DOI: https://doi.org/10.1007/s10310-010-0192-y