Abstract
The influence of substrate composition on the production of enterocin EJ97 and the conditions for semi-preparative bacteriocin recovery have been studied. Final bacteriocin concentrations of 12.5 or 15.6 mg/l were obtained in the commercial media brain heart infusion broth (BHI) and tryptic soya broth, respectively. The bacteriocin was also produced in the complex medium CM (8.75 mg/l), in which the vitamin supplement was essential for production. Some combinations of meat peptone and yeast extract plus either soy peptone or BHI also supported bacteriocin production, at concentrations of 6.25–7.5 mg/l. In cow milk (whole, half-skimmed, and skimmed), the final bacteriocin concentrations obtained ranged from 7.5 to 11.25 mg/l. Highest bacteriocin activity was obtained by using pasteurised milk whey as growth substrate (up to 25 mg/l), suggesting that this bacteriocin can be obtained on a large scale by using this cheap food-grade industrial by-product. Highest bacteriocin titres were always obtained after 8 h of incubation at 37 °C. Semi-preparative concentration and purification of enterocin EJ97 produced in a complex medium was achieved by bulk cation exchange chromatography without previous cell separation, followed by reversed-phase chromatography. This two-step procedure allowed preparation of milligram quantities of purified bacteriocin, which is an improvement compared to purification procedures established for most other bacteriocins (35). The availability of purified enterocin EJ97 will facilitate other studies such as the elucidation of its molecular structure and its interaction with target bacteria.
Similar content being viewed by others
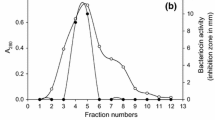
Avoid common mistakes on your manuscript.
Introduction
Bacteriocins produced by lactic acid bacteria (LAB) are antimicrobial substances that may inhibit a wide range of spoilage and pathogenic foodborne bacteria. Therefore, LAB bacteriocins are of increasing interest for preservation of foods either alone or in combination with additional hurdles such as refrigeration, mild heat treatments and chemical preservatives [8, 10]. Bacteriocins can be produced directly in foods by inoculation with bacteriocinogenic starter, adjunct or protective cultures. Alternatively, commercial bacteriocin preparations can be added onto the foods. In order to meet the complex nutritional requirements of LAB and lower the production costs, cheap substrates such as whey, potato waste as well as mussel or fish-processing wastes has been tested for production [2, 12, 20, 21, 23, 25, 31, 33, 36, 37]. Standardization of the optimal conditions for bacteriocin production and recovery are therefore key factors to be determined before doing field studies for bacteriocin application, and several studies to optimise production of the bacteriocins nisin, pediocin PA1/AcH, AS-48 and many others have been carried out [1, 4, 5, 37]. Food-grade lyophilized preparations of crude or partially-purified bacteriocins such as nisin, pediocin PA1/AcH, lacticin 3147 and variacin are available [12, 25, 26, 31, 33]. However, low production yields and difficulties in obtaining significant amounts of pure bacteriocin have limited, in part, the biochemical and physical characterization of the active compounds in many cases [3].
Enterocin EJ97 is a bacteriocin produced by Enterococcus faecalis EJ97 [16] belonging to class II.2 enterocins [13]. The bacteriocin peptide has been purified and sequenced, and the genetic determinants have been identified and studied in detail [34]. This bacteriocin is active against foodborne pathogenic and/or spoilage bacteria (Listeria monocytogenes, Bacillus coagulans, B. macroides/B. maroccanus, Staphylococcus aureus), a reason why it is of great interest for biopreservation [17–19]. One of the most important drawbacks for assay of many bacteriocins in foods is the difficulty to obtain sufficient amounts of partially purified concentrates of high inhibitory activity. In addition, the in-depth characterisation of bacteriocin molecules is often limited by the difficulties to obtain sufficient amounts of high-purity preparations. In the present work, the conditions influencing bacteriocin production, and the semi-preparative bacteriocin purification of enterocin EJ97 are described.
Materials and methods
Bacterial strains and growth media
Enterococcus faecalis EJ97 [16] was used for EJ97 production, and E. faecalis S-47 as indicator for enterocin assay. Enterococci were cultivated on brain heart infusion broth (BHI; Scharlab, Barcelona, Spain) at 37 °C, and maintained routinely at 4 °C on BHI agar slants. Media tested for bacteriocin production are listed in Table 1. Complex medium (CM) was described elsewhere [14]. Tryptic soya broth (TSB), meat peptone, soy peptone and yeast extract were purchased from Scharlab. Casamino acids and yeast nitrogen base (YNB) were from Difco. Commercial milk was from RAM (Lactimilk S.A., Arteixo, A Coruña, Spain). Whey from cow milk fresh cheese was obtained from local factories. Media used for bacteriocin production were buffered with 0.15 M sodium phosphate buffer, except milk. Solid media were prepared by adding 1.5% agar (Scharlab). Media were sterilised by autoclaving at 117 °C for 15 min. YNB was sterilised by filtration through 0.22 μm filters under sterile conditions. Whey was either autoclaved or pasteurised at 70 °C for 15 min.
Bacteriocin production
Batches (100 ml) of the different media were inoculated (4%, v/v) with an overnight culture of the bacteriocin producer strain EJ97, in duplicate, and the cultures were incubated statically under microaerophilic conditions at 37 °C. At desired intervals of incubation (8 and 24 h, or every 2 h), samples were withdrawn under sterile conditions, and centrifuged in a microcentrifuge (12,000g for 5 min). Bacteriocin activity in supernatants was determined by the agar-well diffusion assay as described previously [14]. Briefly, 8 mm-diameter stainless steel cylinders (“Oxford towers”, Scharlab) were laid on 90 mm diameter Petri dishes containing Mueller Hinton agar buffered with 0.15 M sodium phosphate buffer, pH 7.2, and the dishes were overlaid with 6 ml of molten agar (1.5% brain heart infusion plus 0.75% agar in phosphate buffer) inoculated with 50 μl of an overnight growth of the indicator bacterial strain to be tested. After solidification, the wells were removed and filled with 100 μl of the bacteriocin sample to be tested. Samples were incubated at 37 °C for 24 h and the diameter of the halos of inhibited growth around the wells was measured. Samples were tested in duplicate. Bacteriocin activity was calculated as arbitrary units (AU) and converted to mg/l by using the specific activity value of 1.6 AU/μg calculated previously [16].
Bacteriocin purification
The producer strain was cultivated in 10 l of CM broth for 8 h, and the produced bacteriocin was recovered by cation exchange chromatography. Cultured broths were adjusted to pH 6.5 and mixed with Carboxymethyl-Sephadex CM25 (Pharmacia) gel slurry (1:40, v/v) under stirring for 30 min. The mixture was decanted for 30 min and the sedimented gel slurry containing adsorbed bacteriocin was loaded on a 10 × 80 cm glass column. The gel was washed with five bed volumes of distilled water and the retained bacteriocin was eluted with two volumes of NaCl (1.5 M in distilled water). Eluted fractions (30 ml each) were sterilized by filtration (0.45 μm, Millipore) and tested for bacteriocin activity. Active fractions were loaded on a reversed-phase high-performance liquid chromatography (RP-HPLC) Vydac 218TP510 column (10 × 250 mm; The Separation Group, Hesperia, California) previously equilibrated in solvent A (10 mM trifluoroacetic acid, TFA; Fluka), at a flow rate of 5 ml/min. Non-absorbed material was eliminated by washing the column with solvent A until the UV absorbance of the effluent at 280 nm (A280 nm) reached baseline. The material retained in the column was eluted with a gradient of 0–100% of solvent B (Isopropyl-alcohol:acetonitrile (2:1, v/v) in 40 mM TFA) over 30 min at a flow rate of 2.5 ml/min. Fractions of the column effluent were collected according to their UV light absorbance, lyophilized and re-dissolved in distilled water before being tested for bacteriocin activity. The protein concentration of samples was determined according to Bradford [6]. The purity of the bacteriocin after reversed-phase chromatography was tested by SDS-PAGE on 15% acrylamide slab gels according to Laemmli [22], using low range molecular weight markers (SigmaMarker™, Sigma) as standards. Gels were stained with Comassie Brilliant blue R-250.
Results
Influence of medium composition on bacteriocin production
Commercial growth media as well as different combinations of commercial substrates used at low concentration were tested for bacteriocin production (Table 1). In the commercial media BHI and TSB, final bacteriocin concentrations of 20–25 AU/ml (12.5–15.6 mg/l) were obtained after 8 h of incubation. Prolonged incubation of cultures resulted in lower bacteriocin titres.
Enterocin EJ97 (14 AU/ml, or 8.75 mg/l) was produced in a complex medium (CM) composed of low concentrations of BHI, Casamino acids, YNB, and glucose. However, no activity was detected in similar combinations lacking YNB (CM1 and CM2). Among the 14 different combinations of meat peptone tested, only some bacteriocin production (10–12 AU/ml, or 6.25–7.5 mg/l) was detected for media 9, 10, 13 and 14 (Table 1).
Enterocin EJ97 was also produced in commercial whole milk, half-skimmed milk and skimmed milk. Highest titres (18 AU/ml, or 11.25 mg/l) were obtained after 8 h of incubation in half-skimmed milk. As in previous cases, prolonged incubation of cultures for 24 h resulted in lower final bacteriocin concentrations. Controls of milk samples had no bacteriocin activity.
Cow milk whey from two local cheese factories (milk whey 1 and 2 in Table 1) were also tested as substrates for bacteriocin production. Whey was either pasteurised at 70 °C for 15 min or heat-sterilised at 117 °C for 15 min. Highest titres were (40 AU/ml, or 25 mg/l) obtained with pasteurised milk whey 2 after 8 h of incubation (Table 1). However, no bacteriocin activity was obtained when heat-sterilised whey was used as growth substrate. Negative controls consisting of pasteurised or heat-sterilised whey without previous inoculation with the bacteriocinogenic strain did not exhibit any antimicrobial activity.
Time-course of bacteriocin production on whey as substrate
The time course of bacteriocin production was studied on whey (milk whey 2 in Table 1) without added buffer and also on whey buffered with 0.15 M sodium phosphate buffer, pH 7.0 (Fig. 1). In the non-buffered whey, bacteriocin activity was detected after 4 h of incubation, and reached highest concentrations at 8 h. The final pH of the cultured whey was 4.3. In buffered whey, bacteriocin activity was also detected after 4 h of incubation, but highest titres were detected at 6 h, and final activity was higher (40 versus 30 AU/ml, or 25 versus 18.75 mg/l). The final pH of the cultured whey was also higher (5.6).
Growth of E. faecalis EJ97 (light symbols) and bacteriocin production (dark symbols) on milk whey 2 buffered with 0.15 M sodium phosphate buffer, pH 7.0 (filled circle) and on the same non-buffered whey (filled triangle). Cultures were incubated at 37 °C without stirring under microaerophilic conditions. Samples were taken every 2 h and centrifuged, and the supernatants were tested for bacteriocin activity (in arbitrary units, AU) by the agar-well diffusion assay against the indicator strain E. faecalis S-47. Data are the average of duplicates ± SD (error bars)
Bacteriocin purification
Enterocin EJ97 was purified by adaptation of the previously described analytical two-step procedure [16] to a semi-preparative scale. The results obtained for a 10 l batch are shown in Table 2. Bacteriocin was recovered from cultured broths by direct mixing with the cation exchanger Carboxymethyl Sephadex CM-25 without previous separation of cells by centrifugation. The cells and most other components from the growth medium could be washed away with relative ease by passing five bed volumes through the column packed with the gel slurry, and partially-purified and concentrated bacteriocin was recovered from the gel by elution with 1.5 M NaCl (Fig. 2a). The yield of this purification step was 59.46% (Table 2).
Semi-preparative scale purification of enterocin EJ97. a Ten litres of cultured complex medium (CM) broths obtained after 8-h cultivation of the producer strain E. faecalis EJ97 without stirring under microaerophilic conditions were purified by direct cation exchange chromatography on Carboxymethyl-Sephadex CM25. Bacteriocin activity was recovered with 1.5 M NaCl. Bars represent bacteriocin concentration of eluted samples (30 ml each). b Samples from cation exchange chromatography were purified by semi-preparative reversed-phase chromatography on a Vydac 218TP510 column. The proteins retained in the column were eluted with a gradient of 0–100% of solvent B (Isopropyl-alcohol:acetonitrile (2:1, v/v) in 40 mM TFA) over 30 min at a flow rate of 2.5 ml/min. The solvent gradient (dotted line), the UV-absorbance of column effluent (solid line) and bacteriocin activity (arrow) are shown. The amount of bacteriocin purified in this run was 4.2 mg. Bacteriocin activity (in arbitrary units, AU) was determined by the agar-well diffusion assay against the indicator strain E. faecalis S-47. c Sodium dodecyl sulphate polyacrylamide gel electrophoresis (SDS-PAGE) of purified bacteriocin: 2.0 μg (lane 1). Molecular mass markers, in kDa (M)
Semi-preparative scale reversed-phase chromatography was used to obtain purified bacteriocin. Partially purified CM-25 fractions were loaded directly on a Vydac C18 column, and the bacteriocin was recovered as a single elution peak without apparent loss of column resolution (Fig. 2b). The yield of this step was very high, and the specific activity of the bacteriocin was similar to the reported specific activity of 1.60 AU/g of protein for purified enterocin EJ97. The amount of purified bacteriocin obtained for each HPLC run was in the range of 3–5 mg. After this step, the purified fractions yielded a single low-molecular weight band by SDS-PAGE (Fig. 2c).
Discussion
One of the main problems to study the efficacy of bacteriocins in foods is the preparation of concentrates with sufficient activity and on the use of cheap substrates for cultivation of producer strains in order to decrease the costs of bacteriocin production. These questions have been addressed in this work for enterocin EJ97, a bacteriocin with promising applications because of its antimicrobial activity against foodborne pathogenic or spoilage bacteria.
The results obtained in this work on the different growth substrates used for bacteriocin production indicate that the composition of the medium and the concentration of nutrients have a great influence on the production of enterocin EJ97. For example, addition of yeast nitrogen base is essential for bacteriocin production in complex medium (which has a very low concentration of Casamino acids and brain-heart infusion), suggesting a vitamin requirement. Furthermore, in media composed of meat peptone, soy peptone, and yeast extract, concentrations of at least 1% were required for each component in order to achieve bacteriocin production. The concentration of yeast extract could be reduced if 1% brain-heart infusion was added, indicating that this medium (even if used at low concentration) provides the nutrient(s) essential for bacteriocin production. Addition of glucose to growth media did not enhance production, indicating that the carbon source was not a limiting factor. Enterococci are heterotrophic bacteria with known nutritional requirements of vitamins [11, 24, 27, 29, 30] as well as amino acids for growth [29]. This may explain the complexity of media required for enterocin EJ97 production. Since the results from the present study indicate that nutrient limitation severely affects bacteriocin production, this has to be taken into consideration for the design of cheap growth substrates based on by-products from other food industries.
Milk is also a suitable substrate for bacteriocin production, but bacteriocin titres were much lower in whole milk than in half-skimmed or skimmed milk, suggesting that EJ97 may interact with milk fat. Therefore, the fat content of milk-based substrates should be considered if these are to be used for large-scale production. Highest bacteriocin titres were obtained on pasteurised whey, suggesting that this industrial by-product could be used for large-scale production of EJ97. However, no bacteriocin activity was obtained when heat-sterilised whey was used as growth substrate indicating the requirement of a heat-labile nutrient. Accordingly, pre-treatments applied to whey must be specifically determined when using this substrate for industrial production of EJ97. Altogether, the results from this study suggest that milk and dairy industry by-products are good candidates for commercial preparation of enterocin EJ97. Dairy substrates are currently used for production of commercial bacteriocin preparations (Nisaplin™, Alta™ 2341) as well as other bacteriocins under study such as lacticin 3147 and variacin [25, 26, 31].
Production of enterocin EJ97 was also enhanced in buffered whey, suggesting that excess acidification may inhibit production. It has been reported that bacteriocins may differ greatly in their optimum pH for production, with reported values of 5.5 for enterocin 1146 [32], 3.7 for pediocin AcH, 4.5 for sakacin A, 5.0 for leuconocin Lcm1 or 5.8 for nisin [40]. Furthermore, bacteriocin production has also been reported to be enhanced in controlled pH fermentations [40]. Accordingly, pH-controlled fermentations should be used to enhance production of enterocin EJ97, and the pH optimum should be established.
This is the first report on recovery of enterocin EJ97 on a semi-preparative scale without previous separation of cells by centrifugation. The procedure is time-saving and allows easier processing of larger culture volumes. Recovery of bacteriocins from cultured broths is often a limiting step, especially to study their physico-chemical properties. Since bacteriocins often differ substantially in their amino acid sequence and other properties such as net charge or hydrophobicity, there is no universal method for their purification. In addition, because of their small molecular sizes they are often difficult to separate from the components of protein digests used in culture media. Many different methods have been applied for purification of bacteriocins, such as salt precipitation, gel filtration, ion exchange chromatography, hydrophobic interaction chromatography, and reversed-phase high-performance liquid chromatography [7, 28]. Most of these methods produce satisfactory results only on a small scale, but are usually of low yield, very expensive, difficult to handle, and unsuitable when working with the large volumes that are needed for large-scale industrial production and for production of peptides for structural and functional studies [35]. Industrial approaches to recovery of nisin have been reviewed [12]. Methods based on cell adsorption have been described for pediocin [39] and also for nisin in combination with microfiltration [38]. Adsorption of bacteriocins to food-grade diatomite calcium silicate has also been suggested as an alternative large-scale procedure [9], although no particular application has been described yet.
In the present study, we used a simple two-step procedure for bacteriocin recovery based on physico-chemical properties common to many bacteriocins: a net positive charge, and resistance to organic solvents and acidic pH. This procedure was used previously on an analytical scale for purification of enterocin AS-48 [15] and EJ97 [16], and further adapted for large-scale production of AS-48 [1]. The recovery obtained in the present study (48.85%) was somewhat lower compared to the previously-reported values of ca. 54% for EJ97 [16] and of 65–75% for AS-48 [1], but in the case of EJ97 the elimination of the cell separation step notably simplifies the process and compensates for the slightly lower yield obtained. A similar two-step procedure without previous cell separation was described to purify microgram quantities of pediocin-like bacteriocins, with yields of 80–90% or more [35], although the process was not tested on a larger scale. It seems, therefore, that the presence of the bacterial cells during the cation exchange step may have a variable influence on bacteriocin binding depending on the bacteriocin and the producer strain. In the case of enterocin EJ97, this could be due to partial adsorption of bacteriocin molecules to the bacterial cells. Recently, a two-step scalable method based on hydrophobic interaction chromatography step using an octyl-Sepharose column as the second step has been proposed for pediocin PA-1 final purification and polishing with a recovery as high as 73%, which is at least two to three times more than that of other methods reported so far [3]. Scale up recovery of enterocin EJ97 (be it as a partially-purified concentrate or as an homogeneous preparation) is an important step forward in order to study its antimicrobial activity in model food systems and its interaction with target bacteria as well as with food components. The easy recovery of milligram quantities of the purified peptide will also facilitate other studies on the molecular structure and the physico-chemical properties of this bacteriocin.
References
Abriouel H, Valdivia E, Martínez-Bueno M, Maqueda M, Gálvez A (2003) Semipreparative-scale production and recovery of enterocin AS-48 derived from Enterococcus faecalis subsp. liquefaciens A-48-32. J Microbiol Methods 55:599–605
Amiali MN, Lacroix C, Simard RE (1998) High nisin Z production by Lactococcus lactis UL719 in whey permeate with aeration. World J Microbiol Biotechnol 14:887–894
Beaulieu L, Aomari H, Groleau D, Subirade M (2006) An improved and simplified method for the large-scale purification of pediocin PA-1 produced by Pediococcus acidilactici. Biotechnol Appl Biochem 43:77–84
Biswas SR, Ray P, Johnson MC, Ray B (1991) Influence of growth conditions on the production of a bacteriocin, pediocin AcH, by Pediococcus acidilactici H. Appl Environ Microbiol 57:1265–1267
Bhugaloo-Vial P, Grajek W, Dousset X, Boyaval P (1997) Continuous bacteriocin production with high cell density bioreactors. Enzyme Microb Technol 21:450–457
Bradford M (1976) A rapid and sensitive method for the quantitation of microgram quantities of protein utilizing the principle of protein-dye binding. Anal Biochem 72:248–254
Carolissen-Mackay V, Arendse G, Hastings JW (1997) Purification of bacteriocins of lactic acid bacteria: problems and pointers. Int J Food Microbiol 34:1–16
Cleveland J, Montville TJ, Nes IF, Chikindas ML (2001) Bacteriocins: safe, natural antimicrobials for food preservation. Int J Food Microbiol 71:1–20
Coventry MJ, Gordon JB, Alexander M, Hickey MW, Wan J (1996) A food-grade process for isolation and partial purification of bacteriocins of lactic acid bacteria that uses diatomite calcium silicate. Appl Environ Microbiol 62:1764–1769
Deegan LH, Cotter PD, Hill C, Ross P (2006) Bacteriocins: biological tools for bio-preservation and shelf-life extension. Int Dairy J 16:1058–1071
Deibel RH (1964) The group D streptococci. Bacteriol Rev 28:330–366
De Vuyst L, Vandamme EJ (1994) Nisin, a lantibiotic produced by Lactococcus lactis subsp. lactis: properties, biosynthesis, fermentation and applications. In: De Vuyst L, Vandamme EJ (eds) Bacteriocins of lactic acid bacteria: microbiology, genetics and applications. Blackie Academic & Professional, London, pp 152–221
Franz CMAP, van Belkum MJ, Holzapfel WH, Abriouel H, Gálvez A (2007) Diversity of enterococcal bacteriocins and their grouping into a new classification scheme. FEMS Microbiol Rev 31:293–310
Gálvez A, Maqueda M, Valdivia E, Quesada A, Montoya E (1986) Characterization and partial purification of a broad spectrum antibiotic AS-48 produced by Streptococcus faecalis. Can J Microbiol 32:765–771
Gálvez A, Valdivia E, Martínez-Bueno M, Maqueda M (1989) Purification and amino acid composition of peptide antibiotic AS-48 produced by Streptococcus (Enterococcus) faecalis subsp. liquefaciens S-48. Antimicrob Agents Chemother 33:437–441
Gálvez A, Valdivia E, Abriouel H, Camafeita E, Méndez E, Martínez-Bueno M, Maqueda M (1998) Isolation and characterization of enterocin EJ97, a bacteriocin produced by Enterococcus faecalis EJ97. Arch Microbiol 171:59–65
García MaT, Ben Omar N, Lucas R, Pérez-Pulido R, Castro A, Grande MaJ, Martínez-Cañamero M, Gálvez A (2003) Antimicrobial activity of enterocin EJ97 on Bacillus coagulans CECT 12. Food Microbiol 20:533–536
García MaT, Martínez Cañamero M, Lucas R, Ben Omar N, Pérez Pulido R, Gálvez A (2004a) Inhibition of Listeria monocytogenes by enterocin EJ97 produced by Enterococcus faecalis EJ97. Int J Food Microbiol 90:161–170
García MaT, Lucas R, Abriouel H, Ben Omar N, Pérez R, Grande MaJ, Martínez-Cañamero M, Gálvez A (2004b). Antimicrobial activity of enterocin EJ97 against Bacillus macroides/B. maroccanus isolated from zucchini purée. J Appl Microbiol 97:731–737
Guerra NP, Pastrana L (2001) Enhanced nisin and pediocin production on whey supplemented with different nitrogen sources. Biotechnol Lett 23:609–612
Guerra NP, Pastrana L (2002) Production of bacteriocins from Lactococcus lactis subsp. lactis CECT 539 and Pediococcus acidilactici NRRL B-5627 using mussel-processing wastes. Biotechnol Appl Biochem 36:119–125
Laemmli UK (1970) Cleavage of structural proteins during the assembly of the head of bacteriophage T4. Nature 227:680–85
Liu C, Liu Y, Chen S (2005) Effects of nutrient supplements on simultaneous fermentation of nisin and lactic acid from cull potatoes. Appl Biochem Biotechnol 121–124:475–483
McCoy TA, Wender AH (1953) Some factors affecting the nutritional requirements of Streptococcus faecalis. J Bacteriol 65:660–665
Morgan SM, Galvin M, Kelly J, Ross RP, Hill C (1999) Development of lacticin 3147-enriched whey powder with inhibitory activity against foodborne pathogens. J Food Prot 62:1011–1016
Morgan SM, Galvin M, Ross RP, Hill C (2001) Evaluation of spray-dried lacticin 3147 powder for the control of Listeria monocytogenes and Bacillus cereus in a range of food systems. Lett Appl Microbiol 33:387–391
Mulligan JH, Snell EE (1977) Transport and metabolism of vitamin B6 in lactic acid bacteria. J Biol Chem 252:835–839
Muriana PM, Luchansky JB (1993) Biochemical methods for the purification of bacteriocins. In: Hoover DF, Steenson LR (eds) Bacteriocins from lactic acid bacteria. Academic, San Diego, pp 41–57
Murray BE, Singh KV, Ross RP, Heath JD, Dunny GM, Weinstock GM (1993) Generation of restriction map of Enterococcus faecalis OG1 and investigation of growth requirements and regions encoding biosynthetic function. J Bacteriol 175:5216–5223
Niven CF Jr, Sherman JM (1944) Nutrition of the enterococci. J Bacteriol 47:335–342
O’Mahony T, Rekhif N, Cavadini C, Fitzgerald GF (2001) The application of a fermented food ingredient containing ‘variacin’, a novel antimicrobial produced by Kocuria varians, to control the growth of Bacillus cereus in chilled dairy products. J Appl Microbiol 90:106–114
Parente E, Ricciardi A (1994) Influence of pH on the production of enterocin 1146 during batch fermentation. Lett Appl Microbiol 19:12–15
Rodriguez JM, Martinez MI, Kok J (2002) Pediocin PA-1, a wide-spectrum bacteriocin from lactic acid bacteria. Crit Rev Food Sci Nutr 42:91–121
Sánchez-Hidalgo M, Maqueda M, Gálvez A, Abriouel H, Valdivia E, Martínez-Bueno M (2003) The genes coding for enterocin EJ97 production by Enterococcus faecalis EJ97 are located on a conjugative plasmid. Appl Environ Microbiol 69:1633–1641
Uteng M, Hauge HH, Brondz I, Nissen-Meyer J, Fimland G (2002) Rapid two-step procedure for large-scale purification of pediocin-like bacteriocins and other cationic antimicrobial peptides from complex culture medium. Appl Environ Microbiol 68:952–956
Vázquez JA, González MP, Murado MA (2004) Peptones from autohydrolysed fish viscera for nisin and pediocin production. J Biotechnol 112:299–311
Vázquez JA, González MP, Murado MA (2006) Preliminary tests on nisin and pediocin production using waste protein sources. Factorial and kinetic studies. Bioresour Technol 97:605–613
Van´t Hul JS, Gibbons WR (1996) Concentration and recovery of the bacteriocin nisin from Lactococcus lactis subsp. lactis. Biotechnol Appl Biochem 24:251–256
Yang R, Johnson MC, Ray B (1992) Novel method to extract large amounts of bacteriocins from lactic acid bacteria. Appl Environ Microbiol 58:3355–3359
Yang R, Ray B (1994) Factors affecting production of bacteriocins by lactic acid bacteria. Food Microbiol 11:281–291
Acknowledgments
This work was supported by Plan Andaluz de Investigación (group AGR230) and Plan Propio of the University of Jaén.
Author information
Authors and Affiliations
Corresponding author
Rights and permissions
About this article
Cite this article
López, R.L., García, M.T., Abriouel, H. et al. Semi-preparative scale purification of enterococcal bacteriocin enterocin EJ97, and evaluation of substrates for its production. J Ind Microbiol Biotechnol 34, 779–785 (2007). https://doi.org/10.1007/s10295-007-0254-0
Received:
Accepted:
Published:
Issue Date:
DOI: https://doi.org/10.1007/s10295-007-0254-0