Abstract
The geochemistry, mineralogy, and grain size distribution of several estuarine cohesive sediment samples from potentially human-influenced areas without such an influence were analyzed to determine the natural heavy metal content and evaluate its impact on the Bahía Blanca estuarine environment. The data were compared with different ranges of concentrations for heavy metals in marine sediments established by the NOAA Screening Quick Reference Tables in which values range from background levels to those considered toxic to the marine environment. Our total heavy metal contents were below the established hazardous levels in all the analyzed samples, even though the potentially human-influenced areas (harbors, industry, urban spread) showed the highest total concentration values as well as greater percentages of bioavailable compounds. This would imply a low and not extensive anthropogenic input into the environment. The relatively high proportions in which Cd, Pb, and Cr appear as bioavailable compounds at some sites not influenced by human activity suggest the presence of a natural source for these elements. This could be attributed to the weathering of naturally occurring volcanic minerals, indicating that special care must be taken when monitoring of sediment for anthropogenic activity is carried out within this environment. According to the results obtained, and in order to minimize the environmental impact caused by periodic water injection dredging, relocation of sewage outfalls from vessel mooring areas into open waters is strongly recommended.
Similar content being viewed by others
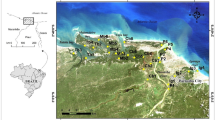
Explore related subjects
Discover the latest articles, news and stories from top researchers in related subjects.Avoid common mistakes on your manuscript.
1 Introduction
Marine sediments represent the final repository of most contaminants and elements carried to the oceans by rivers and at the same time also play a very important role in estuarine systems as potential sources and sinks. Estuaries usually serve as geochemical traps for dissolved materials, firstly increasing their concentration and eventually transferring them to cohesive sediments through different processes. Many anthropogenic pollutants are associated with clay minerals, or at least have a similar dynamical behavior. As a consequence, potentially dangerous substances including heavy metals tend to spread out and become concentrated in those areas where low flow energy allows fine sediment to settle and remain deposited, as over tidal flats and in artificial harbors.
The Bahía Blanca estuary is located in the southern region of the Buenos Aires province of Argentina (Fig. 1). It has a mesotidal semi-diurnal regime, with a mean tidal range from 2.5 m at the mouth to more than 4 m at the head. The inner estuarine zone has a restricted exchange with water from the open Atlantic Ocean, with almost negligible continental runoff. Maximum depths in the estuarine channels are around 20 m, and the intertidal zone, composed mainly by mud flats, represents more than 70% of the total estuarine basin area (Gómez et al. 2008). The strong tidal currents, produced by the high tidal range, lead to a water body of vertically practically homogeneous characteristics. Because of the high suspended sediment content that tidal currents produce, sunlight does not penetrate much into the water column. As a result, major primary production within the estuary takes place on the tidal flats during low tide.
The most important deep-harbor complex in Argentina is located on the northern bank of the estuary, connected to the open ocean through a 100-km-long access channel (Fig. 1). However, several sections of this channel need to be frequently dredged, as well as the mooring sites, in order to maintain navigational depths.
Besides including the most important deep-harbor complex of the country, on the northern coast of the estuary, there are also important industrial nucleuses and large urban populations that provide conditions that are also conducive to the introduction of pollutants into the water environment.
Once polluting substances are deposited, no significantly negative consequences on the environment may be generated due to immobilization. However, the Bahía Blanca estuary mooring sites in the harbor complex are dredged by water injection twice a year (approx. 400,000 m3 year−1). By injection on the bottom of pressurized water, this type of dredging generates a high-density suspension of cohesive sediments that migrate to the deeper areas of the main channel. Injection dredging is an economical way to maintain navigational depths; however, the rapid resuspension of great volumes of cohesive sediment promotes abrupt transfer of immobilized substances as bioavailable compounds, which can thus be disseminated throughout the estuarine environment. Unfortunately, municipalities a well as industries located in the area do not provide the quality and rates of discharges into the estuary. Thus, in order to evaluate the actual degree of the chemical pollution by heavy metals in cohesive sediments that are being dredged, it is first necessary to determine the concentration of hazardous substances that naturally occur in the sediment and compare them with those from the potentially impacted areas.
2 Materials and methods
In this study, 32 sediment samples (Fig. 2) were analyzed: (1) from a functional tidal flat at a southern island, (2) from relict tidal flats deposited between 3500 and 3300 years ago (Borel and Gómez 2006), and (3) from or close to the sites of potential pollutant inputs (mooring areas, sewage, and industrial outfalls). At submerged locations, surface sediment samples were obtained with a dredge made of a 7,800-cm3 capacity plastic canvas bag with a 195-cm2 stainless steel mouth. On board the vessel, samples of about 5,000 cm3 were kept in plastic bags and then stored in a freezer at −20°C until treated in the laboratory.
Sample grain size distributions were obtained following the conventional sieving and pipette procedures (Krumbein and Pettijohn 1956). As it was not feasible to identify optically the minerals below 63 μm, optical observations were carried out using a petrographic microscope on only the very fine sand size fraction that was closest to the chemically analyzed silt–clay fraction. X-ray diffraction patterns from total sample (sand, silt, and clay fractions) and from the clay fraction (grain size <2 μm) were also obtained.
For geochemical analysis, grain size fractions smaller than 63 μm from the collected sediment samples were obtained. The total metal content was determined following the method described by Marcovecchio et al. (1998), which includes wet mineralization with an acid mixture. This technique includes sediment mineralization in a strong acid mixture (HClO4/HNO3, 1:3) under controlled temperature (110 ± 5°C). The residue was diluted in 0.7% (v/v) HNO3 up to 10 ml. Metal concentrations in these solutions were measured using a Perkin Elmer AA-2380 atomic absorption spectrophotometer (AAS) with an air–acetylene flame.
In order to determine the geochemical partitioning of eight heavy metals (Cd, Cu, Pb, Zn, Cr, Ni, Mn, and Fe), a sequential extraction procedure with different solvents was performed. This methodology allows obtaining five geochemical fractions designated as FI of exchangeable adsorbed metals, FII of metals in oxidizable complexes, FIII of metals in carbonates, FIV of metals in reducible compounds, and FV of residual metals. FI added to FII is considered to be the potentially bioavailable fraction of a given heavy metal that could disperse into the estuarine environment as a contaminant. The geochemical partitioning method used is described by Marcovecchio and Ferrer (2005), by Megalatti et al. (1983) modified by Lacerda et al. (1988), and by Maddock and Lopes (1988).
The AAS was used to determine heavy metal concentrations. Analytical grade reagents were used for the blanks and calibration curves, and the analytical quality was checked against reference materials from the National Institute of Environmental Studies in Tsukuba, Japan. All determinations were carried out by duplicates, accepting an error of up to 10%. The results are expressed as micrograms per gram of dry weight, except for Fe which is expressed in milligrams per gram of dry sediment.
3 Results and discussion
3.1 Grain size and mineralogy
Results of the samples’ grain size analysis are shown in Fig. 3 (percentages by weight). All samples show a high proportion of silt and clay (grain size <63 μm), but the majority of the samples from the potentially impacted areas (harbors and tidal flats close to the petrochemical complex located in the area) exhibit silt and clay >80%. Samples from both the ancient tidal flats and those functional tidal flats away from human influence exhibited high proportions of sand (from 40% to more than 60%). The relatively high proportion of clay in samples from the mooring areas is the consequence of the low flow energy in these zones. This fact, as well as the high siltation rates in these areas, suggests that pollutants circulating within the environment are likely to concentrate there.
Optical observations carried out with a petrographic microscope determined that the samples are dominated by plagioclase (10%), quartz (20%), and volcanic glass (around 50%). The heavy mineral content ranged between 2% and 5%, composed mainly of iron ore, amphiboles, and pyroxenes. Some other constituents such as calcite, micas, microcline, and fragments of shells were occasionally found. Volcanic glass was always present as angular shards of different sizes and shapes, colorless to light brown (acid to lightly basic), showing signs of weathering. Through qualitative and quantitative X-ray diffraction patterns from the total sample, the main identified minerals were plagioclase (10–45%), quartz (7–20%), volcanic glass (∼50%), and clay minerals (4–16%); these mainly include smectites as beidellite (15–20%), illite (3–12%), interstratified minerals (3–38%), and chlorite and kaolinite (1–4%).
3.2 Geochemistry
Total metal concentrations as well as their ranges, partial mean values (separating impacted and unimpacted sites), and total mean values of the eight heavy metals in the fine fraction (grain size <63 μm) of the sediment are presented in Table 1. As mentioned, geochemical fractioning of these elements were performed through a sequential extraction procedure using the AAS.
In order to evaluate whether the levels of total heavy metals are dangerous, international screening quick reference tables, National Oceanographic and Atmospheric Administration (NOAA) Screening Quick Reference Tables (SQUIRTs; Buchman 2008), were consulted. These tables present concentrations for inorganic and organic pollutants in various environmental media at different levels, from “background” values (ppm) to those that represent potential risks of contamination in marine sediment, water, and soil. SQUIRTs were developed by the U.S. NOAA for screening purposes. The guideline values are divided into increasing categories of observable effects (Table 2) derived through the use of several approaches (Buchman 2008).
Comparing total metal concentrations in the marine sediments of Bahía Blanca estuary with the respective probable effects levels (background and hazardous levels; Table 3), we can conclude that some concentrations of heavy metals as Cd, Cr, Pb, Ni, and Zn lie over the “background” levels but below the limits of concentration at which adverse effects become persistent. Only Cu has ranges of concentration close to the SQUIRT background. Concentrations of Mn and Fe lie below the background levels of SQUIRTs and are not discussed here because these metals are mainly derived from weathering of heavy minerals present everywhere in the region as iron ores (magnetite, amphiboles, pyroxenes, and biotites; Teruggi 1957; Grecco et al. 2006).
All metal partial mean values are lower in unimpacted areas; some concentration values (Pb, Cu, and Zn) are 50% below those related to anthropogenic input (harbors). For Cd, Cr, and Ni, the concentrations are rather similar in the harbors and the external (non-harbor) areas within the estuary (TRIN, BM1, BM2, and BM3), although they are slightly higher in areas of sewage outfalls (WE, WW, PRN, and PRS). As a whole, only total chromium behaves differently and is preferentially concentrated in harbors with a partial mean value of 9.32 ppm, while in tidal flats close to the petrochemical complex, the partial mean value is 4.59 ppm, which is similar to chromium concentrations from the unimpacted areas.
In harbors, the total concentration of heavy metals (μg/g dry weight) ranges as follows: Cd (0.6–1.6), Pb (5–23), Cu (5–23), Zn (23–87), Cr (4–13), and Ni (8–16.5). In tidal flats close to the petrochemical complex, these values are greater (except for chromium): Cd (0.6–2.9), Pb (8.5–26), Cu(13–21), Zn (59–90.5), Cr (3–6), and Ni (14–25.3); (Fig. 4).
3.3 Bioavailability
As mentioned, fraction FI (exchangeable adsorbed metals) added to FII (metals in oxidizable complexes), i.e., (FI+FII), is considered to be the potentially bioavailable fraction of a given heavy metal. Heavy metal concentrations in bioavailable fractions for each sampling site are given in micrograms per gram, dry weight, in Table 4. Those heavy metals as bioavailable compounds (FI+FII) range as follows: Cd (1.4–0.07), Pb (22–1), Cu (10.5–0.5), Zn (45–1.6), Cr (4.2–0.4), and Ni (12–0.25); (Fig. 5). Fe and Mn are mainly found in the residual fraction (FV), probably associated with the silicate phases of sediments. So they are not represented in the bioavailable fractions (FI+FII) and are not considered in Table 4.
The maximum concentrations of bioavailable compounds appear to be concentrated close to where the industrial and sewage outfalls occur, being notably larger in the intertidal areas where petrochemical outfalls are present (PBB samples). Although this is an important anthropogenic input, the relatively low values at some neighboring sites as Galván Harbor (GWS, GEN, GES), similar to those from the unimpacted areas (BM1, BM2, BM3, TRIN), indicate a generally low and not extensive human impact to the estuarine environment. Exceptions seem to be the harbor areas where sewage outfalls occur, including the inner estuary region (WW, MEGA, PROF) and at the most external harbor Rosales (PRN, PRS). Because in these ports potentially hazardous substances in the sediment deposits are at first immobilized, the impact is initially limited to a small area. However, water injection dredging at the harbor mooring sites expands the affected area as it promotes the transfer of large quantities of bioavailable compounds into the water column. Thus, this abrupt spread of pollutants into larger areas and its subsequent settling over the extensive tidal flats, which affects major primary production that takes place there, could eventually alter the trophic chain of the estuarine ecosystem.
For several years and up until now, Rosales Harbor has been the disposal site for the almost untreated Punta Alta city sewage. A cleanup project that includes elimination of sludge discharge, secondary treatment of sewage, and containment of combined sewer overflows must quickly be carried out. One aspect of the project must be the relocation of the sewage outfall, from the head of Rosales Harbor to a new open site where tidal currents can prevent the local buildup of pollutant concentrations, allowing also complete oxidation of the organic matter. In this way, given the relatively low heavy metal content of the sediments and without the abrupt transfer of pollutants into the water column due to injection dredging, environmental impact would be reduced.
A case similar to ours occurred in Boston Harbor in 1998 (Butman and Bothner 1998; Signell 1997). Sewage outfalls were relocated in the Massachusetts Bay such that the local marine species were put out of danger. The new outfall site in the western portion of the bay has the important advantage of being in deeper water. This new location allows greater dilution of the effluent compared with that in the shallow, confined waters of Boston Harbor. As a result, the areal extent of relatively high effluent levels and its environmental impact is noticeably reduced.
In the present case, the relatively high proportions in which Pb, Cd, and Cr appear as bioavailable compounds at some sites unimpacted by human activity, as in BM samples (Fig. 6), suggest a natural source for these elements. This can be attributed to the weathering of local sediments originating from the Andean volcanic rocks, which are mainly basaltic to andesitic in composition (Teruggi 1957). The weathering of primary minerals of volcanic origin as plagioclases (labradorite and andesine), pyroxenes (augite), amphiboles (hornblende), volcanic glass, and iron ores is likely to increase the concentration of heavy metals as oxidizable complexes and exchangeable adsorbed metals.
4 Conclusions
Based on the results of the present study, the following conclusions can be drawn:
-
1.
There is a natural source of bioavailable compounds of heavy metals that is attributed to the weathering of volcanic glass. Thus, special care should be taken when monitoring estuarine sediments relative to any anthropogenic activity in order to prevent erroneous interpretation of data on heavy metals.
-
2.
There are sites that are clearly impacted, such as locations where petrochemical discharges take place. However, the polluted materials are not dispersed beyond the affected area and are confined to relatively small regions of the estuary. In contrast, periodic dredging by water injection at harbor sites which receive sewage outfalls is believed to increase the impacted area considerably.
-
3.
It is suggested that sewage outfalls located at mooring sites be subjected to appropriate geochemical treatments and relocated to prevent the spreading of potentially dangerous bioavailable compounds in the estuarine environment.
References
Borel CM, Gómez EA (2006) Palinología del Holoceno del Canal del Medio, estuario de Bahía Blanca (Buenos Aires, Argentina). Ameghiniana 43(2):399–412
Buchman MF (2008) NOAA Screening Quick Reference Tables, NOAA OR&R Report 08-1. Office of Response and Restoration Division, National Oceanic and Atmospheric Administration, Seattle, 34 pp
Butman B, Bothner MH (1998) Predicting the long-term fate of sediments and contaminants in Massachusetts Bay. U.S. Geological Survey Sheet FS, pp 172–97
Gómez EA, Borel CM, Aguirre ML, Martínez DE (2008) Radiocarbon reservoir ages and hard-water effect for the northeastern coastal waters of Argentina. Radiocarbon 50(1):119–129
Grecco LE, Marcos AO, Gomez EA, Botté S, Marcovecchio JE (2006) Natural content and anthropogenic input of heavy metals in sediments from Bahia Blanca estuary (Argentina). J Coast Res S39:1021–1025
Krumbein W, Pettijohn F (1956) Manual of sedimentary petrography. Appleton-Century-Crofts, New York, p 549
Lacerda LD, Souza CM, Pestana MHD (1988) Geochemical distribution of Cd, Cu, Cr, and Pb in sediments of estuarine areas along the Southeastern Brazilian coast. In: Seelinger U, de Lacerda LD, Patchineelam SR (eds) Metals in coastal environments of Latin America. Springer, Heidelberg, pp 86–99
Maddock JEL, Lopes CEA (1988) Behaviour of pollutant metals in Aquatic sediments. In: Seeliger U, Lacerda LD, Patchineelam SR (eds) Metals in coastal environments of Latin America. Springer, Heindelberg, pp 100–105
Marcovecchio JE, Ferrer LD (2005) Distribution and geochemical partitioning of heavy metals in sediments of the Bahía Blanca estuary, Argentina. J Coast Res 21(4):826–834
Marcovecchio JE, L Ferrer, A Barral, M Scagliola, A Pucci, 1998. A model for geochemical partitioning of heavy metals in the Mar del Plata coastal ecosystem, Argentina. In: Environmental Geochemistry in the Tropics, JC Wasserman, E Vieira da Silva-Filho, R Villas-Boas (eds), Springer-Verlag , Heidelberg, Ch. X: 131-141.
Megalatti N, Robbe O, Marchandise P, Astruk M (1983) A new chemical extraction procedure in the fractionation of heavy metals in sediments. In: Förstner U (ed) Heavy metals in the environment, vol 4. CEP Consult Ltd., Edinburgh, pp 1090–1093
Signell R (1997) Predicting the impact of relocation Boston’s sewage outfall. Effluent dilution simulation in Massachusetts Bay. U.S. Geological Survey Sheet, Fact Sheet FS 185-97, p 2
Teruggi ME (1957) The nature and origin of Argentine loess. J Sed Petrol 27(3):322–332
Author information
Authors and Affiliations
Corresponding author
Additional information
Responsible Editor: Ashish Mehta
Rights and permissions
About this article
Cite this article
Grecco, L.E., Gómez, E.A., Botté, S.E. et al. Natural and anthropogenic heavy metals in estuarine cohesive sediments: geochemistry and bioavailability. Ocean Dynamics 61, 285–293 (2011). https://doi.org/10.1007/s10236-010-0354-7
Received:
Accepted:
Published:
Issue Date:
DOI: https://doi.org/10.1007/s10236-010-0354-7