Abstract
We studied shoaling behaviour in a species of fish (Garra barreimiae) from Oman. We compared two populations (a surface-dwelling and a cave-dwelling population) with different theoretical costs and benefits of shoaling. We measured the tendency to associate with a shoal of conspecifics. The stimulus shoal was confined to (1) clear Plexiglas cylinders in light, (2) wire-mesh cylinders in light, or (3) wire-mesh cylinders in darkness. The surface form exhibited a strong preference for the stimulus shoal during the experiments in light, but also in darkness, when only non-visual cues from the shoal could be perceived. The cave form did not show a preference when solely visual cues were available (Plexiglas cylinder). When non-visual cues from the shoal could be perceived (wire-mesh), the cave form did show a preference to associate with the shoal, but the shoaling tendency was considerably weaker than in the surface form. The shoaling tendency has probably been genetically reduced in the cave form.
Similar content being viewed by others
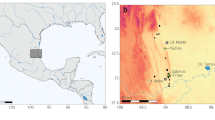
Avoid common mistakes on your manuscript.
Introduction
Shoaling behaviour provides benefits to the individual shoal members: in surface-dwelling fish, shoaling often serves to avoid predation (Brock and Riffenburgh 1960; Neill and Cullen 1973; Seghers 1974; Magurran et al. 1985; Godin 1986; Magurran and Pitcher 1987; Magurran 1990; Pitcher and Parrish 1993; Krause and Ruxton 2002). For example, in the guppy (Poecilia reticulata) the tendency to shoal is less pronounced in habitats with low predation risk (Seghers 1974; Magurran and Seghers 1991; Magurran et al. 1992, 1993). Furthermore, the ability to find food (Keenleyside 1979; Pitcher et al. 1982; Pitcher and Parrish 1993) or the ability to occupy a food source guarded by a physically stronger competitor (Foster 1985; Chapman and Kramer 1996) may increase with increasing shoal size. Additionally, shoaling fish can learn about both predators and prey from conspecifics (e.g., Brown and Laland 2003 for a review).
However, costs of group living arise from competition for food between the individual members of a shoal (see Pulliam and Caraco 1984; Ranta et al. 1993; Krause and Ruxton 2002). One would predict that shoaling behaviour should be reduced if the costs of increased food competition overrun the benefits of lower predation risk and increased food-finding ability. In fact, starved fish tend to shoal less than fish in a good nutritional state, probably because they are more affected by competition from shoal members (van Havre and FitzGerald 1988; Krause 1993; Reebs and Saulnier 1997; Krause et al. 1999).
The study system: habitat differences
Cave habitats differ from many surface habitats in the lack of photoautotrophic primary production due to the absence of light. In most cave ecosystems organic matter washed in from the surface (like leaves) and bat guano are the trophic basis of the subterranean food web (Poulson and White 1969; Parzefall 1993a; Poulson and Lavoie 2000). As a consequence, food is scarce in most caves (Barr and Holsinger 1985). Cave fishes may evolve physiological strategies to cope with this problem, such as reduced metabolic rate (Hüppop 1986) or the development of additional fat-storing tissues (Hüppop 1988, 2000). Most importantly, small fish may suffer intense predation pressure in surface habitats (e.g. by predatory fishes and birds), but predation on cave fishes is mostly absent (Parzefall 1993a; but see Plath et al. 2003a for predation on cave-dwelling Poecilia mexicana). Consequently, benefits of shoaling behaviour in terms of reduced predation risk are lacking in most cave fishes. Furthermore, the costs of food competition can increase in cave habitats due to the low food availability. Altogether, shoaling behaviour has no obvious adaptive significance in cave fishes and should be reduced. If costs of group living are high, cave fishes might even actively avoid conspecifics. In the present article, we tested this prediction in a species of barb (Garra barreimiae Fowler and Steinitz 1956) that has evolved a cave-dwelling population. We compared the shoaling tendency between the surface-dwelling and cave-dwelling forms.
The study species
Surface-dwelling G. barreimiae are endemic to the Arabian Emirates and to the Oman (Fowler and Steinitz 1956; Feulner 1998). G. barreimiae is one of the most common species of fish in the “Widyan”, that is, river systems that periodically fall dry (Krupp and Schneider 1988). This fish has the remarkable ability to move between pools across land in search of other pools (Feulner 1998). G. barreimiae mainly feeds on algae and other epibionts (Büttiker and Krupp 1989). Algae grow in large quantities in the shallow parts of the Widyan, which are intensely penetrated by sunlight (Büttiker and Krupp 1989).
Cave fishes evolve when a previously surface-dwelling species starts to colonise the groundwater of a cave. In our study system, this means that surface-dwelling barbs probably represent the most recent ancestor of the cave form, and cave-dwelling barbs represent the derived form. However, molecular phylogenetic studies on G. barreimiae have so far not been carried out. The cave form of G. barreimiae was discovered by A. Dunsire and M. Gallagher in 1980 in a cave in the Jabal-Akhdar mountain system near Al-Hamra, Oman (Banister 1984, 1987). G. barreimiae is the only known species of fish from this cave. Predation on cave-dwelling G. barreimiae is unknown. Food availability is low in the cave habitat of G. barreimiae (Weissenbacher et al. 2002). We assume that cave-dwelling G. barreimiae feed on bat guano, organic matter washed into the cave, and small invertebrates. However, thorough studies on the ecology of the cave population are lacking.
Sensory basis for shoaling behaviour
During the colonisation of subterranean habitats, formerly surface-dwelling animals have to cope with one predominant problem: due to the absence of light all behaviour patterns that rely on vision become obsolete (Parzefall 1993a). A similar, but less dramatic scenario might occur in shoaling species that are active at night, or in species living in murky water. To compensate for the lack of visual information, other sensory systems (e.g., sense of touch, sense of smell) are often elaborated: for example, sensitive body appendices (i.e. barbells or fins in fishes) may be enlarged in cave dwellers (Weber et al. 1998; Weber 2000). The visual system becomes useless in the dark and is often reduced (e.g. Wilkens 1988; Langecker 2000). If cave fishes exhibited shoaling, they would need to use non-visual senses to detect shoal members. In aquatic cave animals, chemical or mechanical (water displacement) signals appear suitable for this purpose, but non-visual communication has not yet been examined in surface- and cave-dwelling G. barreimiae. Eyes are reduced in the cave form (Banister 1984). However, we detected small externally visible remnants of eyes in the fish reared in our laboratory. It is yet unknown whether these cave fish still perceive visual cues when raised in light. In the present article, we address the following questions on shoaling behaviour in G. barreimiae:
-
(1)
Does the surface form associate with a shoal of conspecifics and is this behaviour reduced in the cave form?
-
(2)
What types of cues (visual and non-visual) are used by the two forms?
To answer these questions, we carried out simultaneous choice tests, giving focal fish a choice of a stimulus shoal and no shoal. We generally expected reduced shoaling or even avoidance of conspecifics in the cave form. Three different treatments were performed:
-
(1)
Only visual cues from the stimulus shoal could be perceived.
-
(2)
Both visual and non-visual cues from the stimulus shoal could be perceived. Here, we wanted to assess the strength of the shoaling tendency in the surface and the cave form when multiple cues were available.
-
(3)
Only non-visual cues from the stimulus shoal could be perceived. These tests were carried out in darkness but allowed for the exchange of chemical and tactile information.
Methods
Study organisms and general housing conditions
We used two populations of G. barreimiae from Oman: the surface-dwelling fish were collected in 1956 by H. W. Fowler and H. Steinitz. The cave form was caught in 1980 in the Hoti cave system (23°05′N, 57°21′E), Jabal-Akhdar mountains, approximately 130 km south-southwest of Maskat, Oman by A. Dunsire and M. Gallagher. Some specimens were transferred to the aquarium of the Zoological Institute in Hamburg for H. Wilkens in 1990. The fish used in our studies came from the third to fourth laboratory generation. Test fish from both populations (surface: 6.78±0.55 cm; cave: 5.68±0.41 cm) were mature and were approximately the same age (7 years) when they were used in the tests. Stocks were maintained in 50- to 200-l aquaria containing hiding places and plants at 25–30°C. Surface and cave fish were kept in different tanks. The bottom was covered with bright gravel. Fish were raised under an artificial 12:12 h light:dark cycle, ensuring that even the cave fish were familiar with light during our tests. Fish were fed twice a day ad libitum with flake food and Artemia naupliae, occasionally supplemented by live cladocerans and Tubifex worms. We were unable to distinguish between the sexes. During the shoaling tests, sexual behaviour was not observed. However, some fish of both populations spawned some days after the experiments. This was probably a response to the previous frequent water exchange, which stimulates spawning (H. Wilkens, personal communication).
Shoaling experiments
The test tank (120×50×40 cm, length × height × width) was filled with aged tap water of 27°C. The bottom was covered with a thin layer of bright gravel. Two vertical lines, marked on the front of the tank, divided the tank into three equal compartments: two lateral preference zones and a central neutral zone. We placed a cylinder (15 cm diameter, 30 cm height) in each of the preference zones to hold the stimulus shoal. Hence, the focal fish could swim around the shoal. We used three different combinations of cylinder materials and illumination conditions (Plath et al. 2003b).
In treatment 1, we used clear Plexiglas cylinders and the tests were carried out in light. The upper part of the cylinders was above water level. The cylinders were not closed at the bottom. However, the bottom was embedded in gravel during the tests, and chemical cues were unlikely to reach the focal fish. Hence, in this treatment, the test fish could perceive only visual cues from the stimulus shoal.
In treatment 2, we used wire-mesh cylinders instead (1 mm wire diameter, 5 mm mesh width) and the tests were also performed in light. In this experiment, the focal fish could perceive both visual and non-visual cues from the stimulus shoal.
In treatment 3, the same type of mesh cylinder was used but the tests were carried out in darkness. In this experimental situation we assumed that the test fish could perceive solely non-visual cues from the shoal.
During the tests in light, the test tank was illuminated from above by a centrally located 36 W incandescent bulb, providing approximately 90 lx on the water surface. The observer sat quietly approximately 2 m from the tank. During the tests in the absence of visible light, a 500 W infrared bulb (wavelength >800 nm) was used instead. In this case, trials were recorded by an infrared-sensitive camera, transferred to a monitor in a neighbouring room, and scored directly.
Before a test, three stimulus fish were randomly taken from the stock tanks and introduced into one cylinder. Stimulus fish came from the same population as the focal fish population. The other cylinder remained empty. We waited until the stimulus fish were swimming calmly. Then, a focal fish was gently introduced into the neutral zone. When the focal fish began to swim, we started measuring the time it spent in both preference zones for 5 min. Then, the position of the two cylinders was carefully exchanged and measurement was repeated. The focal fish remained in the test tank while we exchanged the cylinders. This procedure was performed to detect side biases. Times from both parts of a trial were added. We a priori decided to exclude trials in which the fish spent more than 80% of the choice time in only one compartment during both parts of a trial due to side biases. No trials had to be excluded due to this criterion.
All fish were tested in all three treatments. Between the tests, test fish were kept in separate tanks for 1–2 days. The order of the treatments was random. Focal fish may have served as a stimulus fish in a subsequent trial. Due to the limited number of fish available for the tests (surface form: n=16, cave form: n=11), we could not use completely different stimulus fish for each focal fish tested. However, the combination of individual fish used for the stimulus shoal was random.
Statistical analysis
To test for differences between the two populations and between the three treatments, we calculated the shoaling tendency (time in the compartment with the shoal − time in the opposite compartment). We compared shoaling tendencies using population (surface or cave) as between factor and treatment (treatments 1–3) as repeated measure for a repeated measures analysis of variance (ANOVA) using StatView 5.0.
We post hoc asked whether the focal fish generally responded to the stimulus fish under the three different experimental conditions. Therefore, we tested for differences between the time spent near the shoal and the time spent in the empty compartment during each treatment using paired t tests (SigmaStat 2.0). The latter test allowed us to examine whether the measured association times were due to random distribution of the focal fish in the test tank. Random distribution would be predicted if the focal fish did not perceive any information from the stimulus shoal. Since all data sets were analysed twice, we applied a Bonferroni adjustment as follows: α′=0.05/2=0.025.
Results
Comparison of the shoaling tendency between the populations
Population had a significant influence on shoaling tendency (repeated measures ANOVA: F1,25=128.07, P<0.0001, power=1.0). A post hoc test revealed that the surface fish spent more time near the stimulus fish than did the cave fish (Tukey test: P<0.05; Fig. 1). Treatment also significantly influenced shoaling tendency (repeated measures ANOVA: F2,50=6.11, P=0.0042, power=0.88). A post hoc test revealed that treatment 1 differed from treatments 2 and 3 (Tukey test, P<0.05 in both cases), whereas treatments 2 and 3 did not differ (P>0.05). The interaction term Population × Treatment also had a significant influence on the time spent shoaling (repeated measures ANOVA: F2,50=19.24, P<0.0001, power=1.0). The surface form showed the strongest shoaling tendency during the treatment in which the shoal was presented in Plexiglas cylinders in light (treatment 1), whereas the cave form showed no shoaling in this treatment (Fig. 1).
Shoaling tendency in surface-dwelling (open bars) and cave-dwelling (black bars) Garra barreimiae (time spent near a stimulus shoal − time in an empty control compartment; mean ± SD). A stimulus shoal comprising three conspecifics was confined to (1) clear Plexiglas cylinders in light, (2) wire-mesh cylinders in light, and (3) wire-mesh cylinders in darkness.
General response to the stimulus shoal
In treatment 1, surface-dwelling G. barreimiae spent significantly more time in the compartment with the stimulus shoal (mean ± SD: 386.88±41.94 s) than in the compartment containing the empty Plexiglas cylinder (94.94±44.53 s; paired t test: t15=18.06, P<0.001, α′=0.025, power=1.0; Fig. 1). In treatment 2, the surface fish also spent significantly more time near the stimulus shoal (337.13±63.01 s; empty cylinder: 167.50±40.37 s; t15=7.53, P<0.001, α′=0.025, power=1.0). Also in treatment 3, the test fish significantly preferred the stimulus shoal (paired t test: t15=8.33, P<0.001, α′=0.025, power=1.0). They spent 295.44±30.51 s near the stimulus shoal and 158.88±40.46 s in the empty compartment.
Cave-dwelling G. barreimiae did not prefer either compartment in treatment 1 (near shoal: 237.82±18.54 s; near empty cylinder: 234.55±17.22 s; t10=0.37, P=0.72, α′=0.025, power=0.05). In treatment 2, the cave fish spent significantly more time near the shoal (258.27±31.72 s) than near the empty cylinder (211.64±23.66 s; t10=3.00, P=0.013, α′=0.025, power=0.73). In treatment 3, the cave fish also spent significantly more time in the compartment with the shoal (244.36±18.38 s; empty: 201.64±17.59 s; t10=4.26, P=0.002, α′=0.025, power=0.97).
Discussion
Surface population
We measured the time surface-dwelling and cave-dwelling G. barreimiae spent near a stimulus shoal or in an empty compartment in a simultaneous choice situation. In surface-dwelling G. barreimiae shoaling behaviour appears to be pronounced as they strongly preferred to associate with the stimulus shoal in all three treatments. Shoaling behaviour has also been reported for a variety of other cyprinid fishes (e.g., Magurran et al. 1985; Hager and Helfman 1991; Ashley et al. 1993; Krause 1994; Chivers et al. 1995; Krause et al. 1999; Reebs and Saulnier 1997; Barber and Wright 2001).
The preference to associate with conspecifics was most pronounced in treatment 1, where the shoal was separated from the focal fish by transparent Plexiglas; it was weaker when the shoal was isolated by a mesh grid in light (treatment 2). The visibility of the stimulus shoal was to some extent better in the Plexiglas treatment than in the wire-mesh treatment at least to the observer’s eye. The surface form readily responded to solely non-visual cues (treatment 3). These fish might also shoal at night or in dim light, when non-visual cues are more reliable to locate conspecifics. Furthermore, fish from the surface form mostly swim near the bottom in their natural habitat and often hide under stones or in crevices (Feulner 1998). Here, vision may be obscured, so that surface-dwelling fish occasionally have to rely on non-visual communication. However, previous studies have demonstrated that even sea fish dwelling in open water may school when blinded (Partridge and Pitcher 1980). In summary, surface fish use both visual and non-visual cues to shoal, but shoaling is more pronounced when visual cues are available.
Cave population
In the cave form, no response to solely visual cues was found. The visual system appears to be considerably reduced, thereby making the perception of visual signals impossible even in light-reared animals (see Banister 1984). Alternatively, the perception of visual cues might still be possible but the cave fish do not respond to these cues. Qualitative observations suggest that the cave form does not perceive visual cues such as hand movements outside the tank.
When the focal fish could perceive non-visual cues (treatments 2 and 3), fish from the cave form did shoal, but their shoaling tendency was considerably weaker than that of the surface form. Like the surface-dwelling fish in darkness (treatment 2), fish from the cave form probably use pressure-wave signals to detect the stimulus shoal via their mechanosensory lateral-line system. The lateral-line system is involved in position keeping in schooling fish (Partridge and Pitcher 1980). The lateral line is modified/enhanced in many cave fishes (Poulson 1963; Walters and Walters 1965; Parzefall 1970; Thinès and Durand 1973; Weber 2000). Cave-dwelling Astyanax fasciatus use their lateral-line system for “hydrodynamic imaging” (Hassan 1989), for detecting moving objects (like other fish or prey), and for rheotaxis (Montgomery et al. 2001 for a review). However, little is known about the morphology and physiology of the mechanosensory lateral-line system in surface-dwelling and cave-dwelling G. barreimiae. Besides mechanical (water displacement) cues, chemical cues might be used to locate shoal members. For example, the Somalian cave barb Phreatichthys andruzzii (Cyprinidae) responds to chemical cues from conspecifics (Berti et al. 1989). Further studies will be needed to investigate the relative influence of mechanical and chemical cues on the detection of conspecifics in G. barreimiae.
Reduction of shoaling behaviour in the cave form
Our results confirm the prediction that the shoaling tendency would be reduced in the cave form. Even in darkness, the surface form still exhibited more pronounced shoaling behaviour than the cave form. Since the two populations used in our tests were reared under identical conditions, the differences in the shoaling tendency between surface-dwelling and cave-dwelling G. barreimiae appear to be genetically determined. A potential problem of our study was that fish had been raised in captivity for several years and generations. Hence, we discuss the genetic basis for the observed differences with caution. A reduction of the tendency to form social aggregations has also been reported for other cave fishes, such as the Mexican cave tetra Astyanax fasciatus (Characidae) and the Mexican cave molly Poecilia mexicana (Poeciliidae; Parzefall 1993b), and for the African cave fishes Caecobarbus geertsii, Barbopsis devecchii (Cyprinidae), and Uegitglanis zammaranoi (Clariidae; Jankowska and Thinès 1982). We assume that low predation risk and extremely low food availability (Weissenbacher et al. 2002) have led to the reduction of shoaling behaviour in the cave form of G. barreimiae. However, the cave form did not actively avoid conspecifics and still showed a weak preference for the stimulus shoal. There may be some as yet unknown advantage for this behaviour in the cave form, balancing the selection arising from costs of food competition. Future studies will need to investigate the social behaviour of both forms under natural conditions. In summary, we provide an example of a reduction of shoaling behaviour during the evolution of a cave form by a previously surface-dwelling species of fish.
References
Ashley AJ, Kats LB, Wolfe JW (1993) Balancing trade-offs between risk and changing shoal size in northern red-belly dace (Phoxinus eos). Copeia 1993:540–542
Banister KE (1984) A subterranean population of Garra barreimiae (Teleostei: Cyprinidae) from Oman, with comments on the concept of regressive evolution. J Nat Hist 18:927–938
Banister KE (1987) Two new species of Garra (Teleostei–Cyprinidae) from the Arabian peninsula. Bull Br Mus Nat Hist Zool 52:59–70
Barber I, Wright HA (2001) How strong are familiarity preferences in shoaling fish? Anim Behav 61:975–979
Barr TC, Holsinger JR (1985) Speciation in cave faunas. Annu Rev Ecol Syst 16:313–337
Berti R, Vezzosi R, Ercolini A (1989) Locomotory response of Phreatichthys andruzzii Vinciguerra (Pisces, Cyprinidae) to chemical signals of conspecifics and of closely related species. Experientia 45:205–207
Brock VE, Riffenburgh RH (1960) Fish schooling: a possible factor in reducing predation. J Conseil 25:307–317
Brown C, Laland KN (2003) Social learning in fishes: a review. Fish Fisheries 4:280–288
Büttiker W, Krupp F (1989) Fauna eines Sandmeeres—Zoologische Untersuchung in der Wahiba—Wüste, Oman. Nat Mus 119:241–261
Chapman MR, Kramer DL (1996) Guarded resources: the effect of intruder number on the tactics and success of defenders and intruders. Anim Behav 52:83–94
Chivers DP, Brown GE, Smith RJF (1995) Familiarity and shoal composition in fathead minnows (Pimephales promelas): implications for antipredator behaviour. Can J Zool 73:955–960
Feulner G (1998) Wadi fish of the UAE. Tribulus 8(2):16–22
Foster SA (1985) Group foraging by a coral reef fish: a mechanism for gaining access to defended resources. Anim Behav 33:779–782
Fowler HW, Steinitz H (1956) Fishes from Cyprus, Iran, Iraq, Israel and Oman. Bull Res Counc Isr 5B:260–292
Godin JGJ (1986) Antipredator function of shoaling in teleost fishes: a selective review. Naturaliste Can 113:241–250
Hager MC, Helfman GS (1991) Safety in numbers—shoal choice by minnows under predatory threat. Behav Ecol Sociobiol 29:271–276
Hassan ES (1989) Hydrodynamic imaging of the surroundings by the lateral line of the blind cave fish Anoptichthys jordani. In: Coombs S, Gorner P, Munz H (eds) The mechanosensory lateral line neurobiology and evolution. Springer, Berlin Heidelberg New York, pp 217–228
van Havre N, FitzGerald GJ (1988) Shoaling and kin recognition in the threespine stickleback (Gasterosteus aculeatus L.). Biol Behav 13:190–201
Hüppop K (1986) Oxygen consumption of Astyanax fasciatus (Characidae, Pisces): a comparison of epigean and hypogean populations. Environ Biol Fish 17:299–308
Hüppop K (1988) Phänomene und Bedeutung der Energieersparnis beim Höhlensalmler Astyanax fasciatus. PhD thesis, University of Hamburg
Hüppop K (2000) How do cave animals cope with the food scarcity in caves? In: Wilkens H, Culver DC, Humphries WF (eds) Ecosystems of the world 30: subterranean ecosystems. Elsevier, Amsterdam, pp 159–188
Jankowska M, Thinès G (1982) Étude comparative de la densité de groupes de poissons cavernicoles et épigés (Characidae, Cyprinidae, Clariidae). Behav Process 7:289–294
Keenleyside MHA (1979) Diversity and adaptation in fish behaviour. Springer, Berlin Heidelberg New York
Krause J (1993) The influence of hunger on shoal size choice by three-spined sticklebacks, Gasterosteus aculeatus. J Fish Biol 43:775–780
Krause J (1994) The influence of food competition and predation risk on size-assortative shoaling in juvenile chub (Leuciscus cephalus). Ethology 96:105–116
Krause J, Ruxton GD (2002) Living in groups. Oxford University Press, Oxford
Krause J, Hartmann N, Pritchard VL (1999) The influence of nutritional state on shoal choice in zebrafish, Danio rerio. Anim Behav 57:771–775
Krupp F, Schneider W (1988) Die Süβwasserfauna des Vorderen Orients. Anpassungsstrategien und Besiedlungsgeschichte einer zoogeographischen Übergangszone. Nat Mus 118(7):193–212
Langecker TG (2000) The effects of continuous darkness on cave ecology and cavernicolous evolution. In: Wilkens H, Culver DC, Humphries WF (eds) Ecosystems of the world 30: subterranean ecosystems. Elsevier, Amsterdam, pp 135–157
Magurran AE (1990) The adaptive significance of schooling as an antipredator defence in fish. Ann Zool Fenn 27:51–66
Magurran AE, Pitcher TJ (1987) Provenance, shoal size and the sociobiology of predator evasion behaviour in minnow shoals. Proc R Soc Lond B 229:439–445
Magurran AE, Seghers BH (1991) Variation in schooling and aggression amongst guppy (Poecilia reticulata) populations in Trinidad. Behaviour 118:214–234
Magurran AE, Oulton W, Pitcher TJ (1985) Vigilant behaviour and shoal size in minnows. Z Tierpsychol 67:167–178
Magurran AE, Seghers BH, Carvalho GR, Shaw PW (1992) Behavioural consequences of an artificial introduction of guppies (Poecilia reticulata) in N. Trinidad: evidence for the evolution of antipredator behaviour in the wild. Proc R Soc Lond B 248:260–277
Magurran AE, Seghers BH, Carvalho GR, Shaw PW (1993) Evolution of adaptive variation in antipredator behaviour. In: Huntingford FA, Torricelli P (eds) Behavioural ecology of fishes. Harwood Academic, Amsterdam, pp 29–44
Montgomery JC, Coombs S, Baker CF (2001) The mechanosensory lateral line system of the hypogean form of Astyanax fasciatus. Environ Biol Fish 62:87–96
Neill SR, Cullen JM (1973) Experiments on whether schooling by their prey affects the hunting behaviour of cephalopods and fish predators. J Zool Lond 172:549–569
Partridge BL, Pitcher TJ (1980) The sensory basis for fish schools: relative roles of lateral line and vision. J Comp Physiol A 135:315–325
Parzefall J (1970) Morphologische Untersuchungen an einer Höhlenform von Mollienesia sphenops (Pisces, Poeciliidae). Z Morph Tiere 68:323–342
Parzefall J (1993a) Behavioural ecology of cave-dwelling fishes. In: Pitcher TJ (ed) Behaviour of teleost fishes, 2nd edn. Chapman and Hall, London, pp 573–608
Parzefall J (1993b) Schooling behaviour in population-hybrids of Astyanax fasciatus and Poecilia mexicana (Pisces, Characidae and Poeciliidae). In: Schröder H, Bauer J, Schartl M (eds) Trends in ichthyology. Blackwell Science, Oxford, pp 297–303
Pitcher TJ, Parrish JK (1993) Functions of shoaling behaviour in teleosts. In: Pitcher TJ (ed) Behaviour of teleost fishes, 2nd edn. Chapman and Hall, London, pp 363–437
Pitcher TJ, Maggurran AE, Winfield I (1982) Fish in larger shoals find food faster. Behav Ecol Sociobiol 10:149–151
Plath M, Parzefall J, Schlupp I (2003a) The role of sexual harassment in cave and surface-dwelling populations of the Atlantic molly, Poecilia mexicana (Poeciliidae, Teleostei). Behav Ecol Sociobiol 54:303–309
Plath M, Wiedemann K, Parzefall J, Schlupp I (2003b) Sex recognition in surface and cave-dwelling male Atlantic mollies Poecilia mexicana (Poeciliidae, Teleostei). Behaviour 140:765–782
Poulson TL (1963) Cave adaptation in amblyopsid fishes. Am Midl Nat 70:257–290
Poulson TL, Lavoie KH (2000) The trophic basis of subterranean ecosystems. In: Wilkens H, Culver DC, Humphries WF (eds) Ecosystems of the world 30: subterranean ecosystems. Elsevier, Amsterdam, pp 231–249
Poulson TL, White WB (1969) The cave environment. Science 165:971–981
Pulliam HR, Caraco T (1984) Living in groups: is there an optimal group size?. In: Krebs JR, Davies NB (eds) Behavioural ecology: an evolutionary approach. Blackwell Scientific, Oxford, pp 122–147
Ranta E, Rita H, Lindström K (1993) Competition versus cooperation: success of individuals foraging alone and in groups. Am Nat 142:42–58
Reebs SG, Saulnier N (1997) The effect of hunger on shoal choice in golden shiners (Pisces: Cyprinidae, Notemigonus crysoleucas). Ethology 103:642–652
Seghers BH (1974) Schooling behaviour in the guppy Poecilia reticulata: an evolutionary response to predation. Evolution 28:486–489
Thinès G, Durand J-P (1973) Connaissances actuelles sur l’appareil sensoriel de la ligne latérale chez des vertébrés cavernicoles aquatiques. Ann Spéléol 28:271–282
Walters LH, Walters VW (1965) Laboratory observations on a cavernicolous poeciliid from Tabasco, Mexico. Copeia 1965:214–233
Weber A (2000) Fish and amphibia. In: Wilkens H, Culver DC, Humphries WF (eds) Ecosystems of the world 30: subterranean ecosystems. Elsevier, Amsterdam, pp 109–132
Weber A, Proudlove GS, Parzefall J, Wilkens H, Nalbant TT (1998) Morphology, systematic diversity, distribution and ecology of stygobitic fishes. In: Juberthie C, Decu V (eds) Encyclopaedia Biospeologica, Tome II. Société de Biospéologie, Moulis-Bucarest, pp 835–1373
Weissenbacher A, Sattmann H, Christ M, Scattolin G, Ahnelt H (2002) Auf der Spur der blinden Höhlenfische. Aquaristik-Fachmagazin 34:66–72
Wilkens H (1988) Evolution and genetics of epigean and cave Astyanax fasciatus (Characidae, Pisces). Support for the neutral mutation theory. Evol Biol 23:271–367
Acknowledgements
The authors would like to thank H. Wilkens for access to his fish stocks. A. Weber and J. Parzefall kindly read an earlier version of this article. Two anonymous reviewers provided valuable comments. We thank T.H. Dirks, I.D. Schmidt, A. Taebel-Hellwig, and the aquarium team in Hamburg for technical assistance and animal care. The experiments presented in this article comply with the current laws in Germany.
Author information
Authors and Affiliations
Corresponding author
Additional information
Communicated by R.F. Oliveira
Rights and permissions
About this article
Cite this article
Timmermann, M., Schlupp, I. & Plath, M. Shoaling behaviour in a surface-dwelling and a cave-dwelling population of a barb Garra barreimiae (Cyprinidae, Teleostei). acta ethol 7, 59–64 (2004). https://doi.org/10.1007/s10211-004-0099-8
Received:
Revised:
Accepted:
Published:
Issue Date:
DOI: https://doi.org/10.1007/s10211-004-0099-8