Abstract
In this study, a full-length cytosolic heat shock protein 70 complementary DNA (cDNA) of Laminaria japonica (designated as LJHsp70) was obtained by reverse transcriptase-polymerase chain reaction (RT-PCR) coupled with rapid amplification of cDNA ends. The full length of LJHsp70 cDNA was 2,918 bp, with a 5′ untranslated region of 248 bp, a 3′ untranslated region of 696 bp, and an open reading frame of 1,974 bp encoding a polypeptide of 657 amino acids with an estimated molecular mass of 72.03 kDa and an estimated isoelectric point of 4.97. There was highly repeated sequence of CAA in 5′ untranslated region of LJHsp70. The result of phylogenetic tree of Hsp70s, the BLAST program, analysis and cytosolic Hsp70-specific motif of LJHsp70 verified that the cloned LJHsp70 belonged to cytosolic Hsp70 family. Three typical Hsp70 signature motifs were detected in LJHsp70 by InterPro analysis. Under different stress conditions, messenger RNA (mRNA) expression levels of LJHsp70 were quantified by quantitative RT-PCR. To L. japonica sporophytes kept in different temperatures for 1 h, the expression level of LJHsp70 at 30°C was highest and twofold higher than that at 10°C. To L. japonica sporophytes kept at 25°C for different times, the mRNA expression level of LJHsp70 reached a maximum level after 7 h and then dropped progressively. The expression level of LJHsp70 at 0 or 5‰ salt concentration for 2 h was twofold higher than that at 30‰ salt concentration for 2 h. The results showed that LJHsp70 may be a kind of potential biomarker used to monitor environment conditions.
Similar content being viewed by others
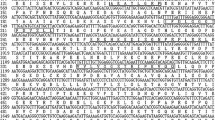
Avoid common mistakes on your manuscript.
Introduction
Stress factors, including heat shock and also a number of chemical, physical, and biological stressors, are able to affect the tertiary structure of proteins, disrupt normal cellular processes, and cause rapidly increased synthesis of a group of proteins belonging to heat shock protein families (Hsps; e.g., Goodman and Blank 1998; Wagner et al. 1999; Mager et al. 2000; Lewis et al. 2001; Li and Guy 2001; Snyder et al. 2001; Sørensen and Loeschcke 2001; Rizhsky et al. 2002; Barua and Heckathorn 2006).
Of all Hsp families, heat shock protein 70s (Hsp70s) are a highly conserved and ubiquitous protein family that is highly conserved across all domains of life (Gupta and Golding 1993; Boorstein et al. 1994; Karlin and Brocchieri 1998; Feder and Hofmann 1999). They play essential roles in transporting nascent proteins across membranes into organelles, folding newly translated proteins, repairing misfolded proteins, and helping target damaged proteins for degradation (Gething and Sambrook 1992; Nelson et al. 1992; Hartl 1996; Morimoto et al. 1997; Fink 1999). Under stress conditions, Hsp70s are upregulated, participate in the refolding of denatured proteins, maintain cell homeostasis, and protect organisms from damage (Jolly and Morimoto 1999; Hartl and Hayer-Hartl 2002; Mayer and Bukau 2005; Tanaka et al. 2007; Mycko et al. 2008). Major inducing factors for Hsp70s upregulation are the occurrence of damaged cellular proteins (Feder and Hofmann 1999). Regulation of Hsp70 expression occurs mainly at transcriptional level (Ananthan et al. 1986). Apart from this cytosolic function, they have been shown to migrate into the cell nucleus where they act to repair or protect the nuclear proteins and to minimize protein aggregation (Ohtsuka and Hata 2000). Hsp70s are also known to play important roles in transporting damaged proteins to lysosomes for degradation, suppressing apoptosis pathways, and providing cells with increased time to repair damage (Wickner et al. 1999; Verbeke et al. 2001).
So far, all Hsp70s possess three distinct domains: an N-terminal adenosine triphosphatase (ATPase) domain, a substrate-binding domain, and a highly variable C-terminal domain. Plant Hsp70s possess at least four types of Hsp70s, each of which localizes to a different cellular compartment. The C terminus of each subgroup is unique and highly conserved. The motif for cytosolic group is EEVD or EEID, for endoplasmic reticulum (ER) group is HDEL, for mitochondrion (MT) group is PEAEYEEAKK, and for chloroplast (CP) group is PEGDVIDADFTDSK (Guy and Li 1998; Sung et al. 2001). A recent comparative analysis of Algal Hsp70s found that red algae, green algae, and diatoms also have multiple Hsp70s, which localize to cytoplasm, mitochondria, chloroplast, or endoplasmic reticulum (Renner and Waters 2007).
Hsp70s are a kind of potential biomarker of environmental stresses and can be applied to monitor environmental conditions (Tomanek and Sanford 2003; Dahlhoff 2004; Ireland et al. 2004). Stress response studies have been carried out with intertidal invertebrates (e.g., Hofmann and Somero 1995; Halpin et al. 2002; Tomanek and Sanford 2003; Hamdoun et al. 2003; Sagarin and Somero 2006) and terrestrial plants (e.g., Howarth 1991; Downs et al. 1998; Hong and Vierling 2000; Manitašević et al. 2007). The intertidal and subtidal zones are a dynamic environment that undergoes fierce and rapid changes in physical parameters with the turning tides and alterations caused by diurnal, seasonal, and meteorological variations (Collén et al. 2007). Therefore, investigations of stress response in non-mobile organisms such as seaweeds are especially interesting because they are unable to escape from unfavorable conditions. Thus, they must make appropriately physiological and biochemical changes in order to deal with environmental stresses. Stress response has been studied in few species of macroalgae to date (Vayda and Yuan 1994; Lewis et al. 1998; Ireland et al. 2004; Li and Brawley 2004; Roeder et al. 2005; Collén et al. 2007; Henkel and Hofmann 2008a,b). Laminaria japonica is a representative and important species of Phaeophyta because it forms seaweed ecosystems along Asian coasts, and it is used commercially as a foodstuff and a raw material for iodine, mannitol, and alginate production in eastern Asian countries (Suzuki et al. 2006). For better understanding, the mechanism of seaweeds response to the ecological and environmental stimulation, a cytosolic Hsp70 complementary DNA (cDNA) of L. japonica was obtained by reverse transcriptase-polymerase chain reaction (RT-PCR) and rapid amplification of cDNA ends (RACE). Under different stress conditions, messenger RNA (mRNA) expression levels of LJHsp70 were investigated using real-time quantitative RT-PCR (qRT-PCR).
Materials and Methods
Laminaria japonica Sample
L. japonica sporophytes (10–15 cm in length) were collected from Qingdao, China on 28th November 2007 and cultured in aerated seawater at 10°C, 10 μmol m−2 s−1 in 12:12 h (L/D) for 7 days before experimentation. About 5 g of L. japonica sporophytes was used in each stress treatment group.
Stress Treatments
L. japonica sporophytes were kept in 30°C for 1 h for extracting total RNA and cloning full-length cDNA of LJHsp70 gene. In heat shock temperature treatment, L. japonica sporophytes were kept in different temperatures (5°C, 10°C, 15°C, 20°C, 25°C, 30°C, 35°C, and 40°C, respectively) for 1 h to investigate the effects of temperatures on expression level of LJHsp70 mRNA. In heat shock time treatment, L. japonica sporophytes were kept at 25°C for different times (0, 1, 2, 3, 4, 5, 6, 7, 8, 9, 10, 11, and 12 h, respectively) to investigate the effects of heat shock times on expression level of LJHsp70 mRNA. In salt concentration challenge treatments, L. japonica sporophytes were kept at 10°C in different salt concentrations (0‰, 5‰, 10%, 15‰, 20‰, 25‰, 30‰, 35‰, 40‰, and 45‰, respectively) for 2 h to investigate the effects of salt concentration challenges on expression level of LJHsp70 mRNA.
RNA Extraction
Because of high levels of polysaccharides, tannins, and phenolics present in L. japonica tissue, RNA extraction kits generally fail to recover high-quality RNA. In this work, total RNA of L. japonica sporophyte was extracted using the modified method of cetyl trimethylammonium bromide (CTAB; Yao et al. 2009). The concrete protocol was described as follows. About 0.1 g of L. japonica sample was grinded to powder in liquid nitrogen with mortar and pestle. Two milliliter of extraction buffer [100 mM Tris (pH 7.5), 50 mM EDTA, and 2 M NaCl] and 100 µl of 5 M dithiothreitol were added to the powdered tissue, transferred into an RNase-free EP tube and kept in room temperature for 15 min. Two milliliter of chloroform/isoamyl alcohol (24:1, V/V) was added to the mixture, mixed thoroughly, and centrifuged at 12,000×g for 20 min at 4°C. Supernatant was transferred to a clean RNase-free EP tube, added 0.5 ml of absolute ethanol and 2 ml of chloroform/isoamyl alcohol (24:1, V/V), mixed thoroughly, and centrifuged at 12,000×g for 20 min at 4°C. Supernatant was transferred to a clean RNase-free EP tube and added 0.5 ml of 12 M LiCl solution, mixed thoroughly, and stored in −80°C for 30 min. RNA precipitation was collected by centrifugation at 14, 000×g for 20 min at 4°C. The pellet was washed twice with 70% ethanol, dried under vacuum, and resuspended in RNase-free water. DNA was removed from total RNA using RQ1 RNase-Free DNase kit (Promega, Wisconsin, USA). Concentration of total RNA was determined by measuring ultraviolet (UV) absorbance at 260 nm. RNA purity was checked by determining the A 260/A 280 ratio. RNA integrity was checked by formaldehyde agarose gel electrophoresis.
LJHsp70 cDNA Cloning
Single-strand cDNA of LJHsp70 was synthesized using RNA PCR Kit (AMV) Ver. 3.0 (TaKaRa, Tokyo, Japan). PCR was carried out in a programmable Mastercycler personal thermocycler (Eppendorf, Hamburg, Germany) in a 10-µl reaction volume containing 1.0 µl of 10× RT buffer, 2.0 µl of MgCl2 (25 mM), 1.0 µl of each deoxyribonucleotide triphosphate (10 mM), 3.75 µl of RNase-free dH2O, 0.25 µl of RNase inhibitor, 0.5 µl of avian myeloblastosis virus reverse transcriptase, 0.5 µl of oligo dT-adaptor primer (2.5 μM), and 1.0 µl of total RNA (about 1 µg). Reaction was incubated at 30°C for 10 min, then incubated at 42°C for 30 min, terminated by heating at 99°C for 5 min, and subsequently stored at −80°C or used for PCR. One pair of degenerated primers, Hsp-F (5′-GASGGYATCGACTTYAACTC-3′) and Hsp-R (5′-TCCTTRCCCGTMGAYTTCTCCAC-3′), were designed on the basis of known Hsp70s of algae (accession number EU780017, AB023580, M27825, and EF537647) for amplifying a cytosolic LJHsp70 cDNA fragment of about 630 bp. PCR was performed in a 50 µl reaction volume containing 3 µl of cDNA as a template, 10 µl of 5× PCR buffer, 33.75 µl of dH2O, 0.25 µl of TaKaRa Ex Taq HS, and 1.5 µl of each primer (10 mM). PCR was carried out at 94°C for 3 min, followed by 35 cycles of 94°C for 30 s, 55°C for 30 s, 72°C for 1 min, and a final extension at 72 C for 10 min. The interest fragment of PCR product was excised, purified by agarose gel DNA fragment recovery kit (TaKaRa, ToKyo, Japan), subcloned into PMD-18T vector (TaKaRa, Tokyo, Japan), and sequenced (Sangon, Shanghai, China).
Rapid Amplification of cDNA Ends
Sequence obtained by RT-PCR was used to design specific primers, GSP-3 (5′-CACGCGGTGGAGAAGTCGACGGGCAAGG-3′) and GSP-5 (5′-CGTGGATCTGCCCCTTCGACAACCTGG-3′) to perform 3′ and 5′ RACE, respectively. Both 3′ and 5′ RACE were carried out using a Smart RACE cDNA amplification Kit (Clontech, CA, USA) according to the manufacturer’s instructions. Amplifications were performed on a Mastercycler personal thermocycler (Eppendorf, Hamburg, Germany). Touchdown PCR was used for RACE amplification. The PCR programs were carried out five cycles of 94°C for 30 s, 72°C for 2 min, followed by five cycles of 94°C for 1 min, 70°C for 30 s, 72°C for 2 min, then 28 cycles of 94°C for 30 s, 65°C for 30 s, 72°C for 2 min, and a final extension at 72°C for 10 min. The PCR products were resolved by electrophoresis on 1% agarose gel. The interest fragment was excised, purified by agarose gel DNA fragment recovery kit (TaKaRa, ToKyo, Japan), cloned into PMD-18T vector (TaKaRa, Tokyo, Japan), and sequenced (Sangon, Shanghai, China).
Analysis and Alignment of Amino Acid Sequence
The similarity analysis of nucleotide and protein sequence was carried out by using BLAST 2.0 at NCBI (http://www.ncbi.nlm.nih.gov/blast). The deduced amino acid sequence was analyzed with the Expert Protein Analysis System (http://www.expasy.org/). According to the methods, designated naming system and criteria of Renner and Waters (2007), Hsp70s of Thalassiosira pseudonana (five types), Chlamydomonas reinhardtii (five types), Ostreococcus lucimarinus (five types), Ostreococcus tauri (five types), and Cyanidioschyzon merolae (four types) were used as criteria to distinguish different types of Hsp70s. Because the cloned LJHsp70 belonged to cytosolic group, so typical Hsp70s of land plants and algae belonging to cytosolic group having C terminus motif of EEVD or EEID were selected and used to construct phylogenetic tree. Meanwhile, some Hsp70s of algae belonging to other groups (ER, MT, and CP groups) were also used to construct phylogenetic tree to deduce phylogenetic relationship among different Hsp70 groups. Multiple alignments of the Hsp70 proteins were performed with the ClustalW multiple alignment program (http://www.ebi.ac.uk/clustalw/). A phylogenetic tree was constructed using the program Mega 4.0.
Real-Time Quantitative RT-PCR
qRT-PCR was performed with the SYBR Premix Ex Taq (Takara, Tokyo, Japan) on an ABI 7300 Real-Time Detection System (Applied Biosystems, CA, USA) to investigate mRNA expression level of LJHsp70. Two specific primers, Hsp70-F (5′-GGCAAAGATAAATCGGAGAAGC-3′) and Hsp70-R (5′-CCCTCAAATACCTGGATAAGCACA-3′) were used to amplify a PCR product of 194 bp. Two β-actin primers, Actin-F (5′-AGGACCTCTACGGCAACATCG-3′) and Actin-R (5′-CGGCCTTGGAAATCCACATC-3′) based on sequence from GenBank (accession number FJ375360) were used to amplify a β-actin gene fragment of 210 bp as the internal control for qRT-PCR. The qRT-PCR amplifications were carried out in a total volume of 20 μl containing 10 μl of SYBR Premix Ex Taq (Takara, Tokyo, Japan), 2 μl of cDNA, 0.4 μl of each forward and reverse primer (10 μM), 0.4 μl of ROX Reference Dye (50×), and 6.8 μl of PCR-grade water. The amplification protocol was carried out at 95°C for 10 s, followed by 40 cycles of 95°C for 5 s and 60°C for 30 s. Three independent biological replicates were carried out. Melting curve analysis of amplification products was performed at the end of each PCR reaction to confirm that only one PCR product was amplified and detected. After the PCR program, qRT-PCR data from three replicate samples were analyzed with a 7300 System SDS Software v1.3 (Applied Biosystems, CA, USA) to estimate transcript copy numbers for each sample. To maintain consistency, the baseline was set automatically by the software. The comparative cycle threshold (C T) method was used to analyze the expression level of LJHsp70. The C T for the target amplification of LJHsp70 and the C T for the internal control β-actin were determined for each sample. Differences between the C T for the target and the internal control, called \(^\Delta C_{\text{T}} \), were calculated to normalize the differences in the amount of total nucleic acid added to each reaction and the efficiency of the RT-PCR. The blank group was used as the reference sample, called the calibrator. The \(^\Delta C_{\text{T}} \) for each sample was subtracted from the \(^\Delta C_{\text{T}} \) of the calibrator; the difference was called \(^{\Delta \Delta } C_{\text{T}} \) value. The expression level of LJHsp70 could be calculated by \(2^{ - \Delta \Delta } C_{\text{T}} \), and the value stood for an n-fold difference relative to the calibrator. The average cycle threshold (C T) measurement for the three determinations was used in the calculation of relative expression using β-actin as the internal control. The data obtained from real-time PCR analysis were subjected to one-way analysis of variance followed by Tukey honestly significant difference test to determine differences in the mean values among the treatments. Significance was concluded at P < 0.05. Statistical analysis was performed using SPSS 13.0 for Windows.
Results
RNA Extraction, cDNA Cloning, and Sequencing of LJHsp70 Gene
In this study, total RNA of L. japonica isolated by using the method of CTAB mentioned above was high quality enough for RT-PCR and RACE-PCR. LJHsp70 cDNA fragment was amplified by degenerated primers mentioned above and confirmed highly similar with known cytosolic Hsp70 genes of algae and land plants. The full-length LJHsp70 cDNA was obtained by 3′ and 5′ RACE-PCR. The complete sequence of LJHsp70 cDNA was deposited in GenBank under accession number FJ375359.
Analysis and Alignment of Amino Acid Sequence
The full length of LJHsp70 cDNA was 2,918 bp, with a 5′ untranslated region of 248 bp with highly repeated sequence of CAA and rich content of nucleotide C and A, a 3′ untranslated region of 696 bp, and an open reading frame of 1,974 bp encoding a polypeptide of 657 amino acids (Fig. 1). The theoretical molecular weight and isoelectronic point of LJHsp70 based on the deduced amino acid sequence was calculated to be 72.03 kDa and 4.97, respectively. The LJHsp70 was comprised of 105 negatively charged residues (Asp and Glu) and 81 positively charged residues (Arg and Lys). The contents of Glu (9.6%), Ala (9.1%), Gly (8.5%), and Lys (7.3%) were high, while the contents of Cys (0.9%), His (0.8%), and Trp (0.5%) were low. The deduced amino acid sequence of LJHsp70 cDNA included three typical motifs of the Hsp70 family at residues 11–18 (IDLGTTYS), 199–212 (IFDLGGGTFDVSVL), and 312–326 (VVLVGGSTRIPKVQQ). The LJHsp70 was composed of an ATPase domain from residues 1 to 383, a substrate peptide binding domain from residues 384 to 546, and a C terminus domain from residues 547 to 657. Cytosolic Hsp70 C terminus generally contained the cytosolic Hsp70-specific motif, EEVD. However, the amino acid sequence of cytosolic LJHsp70 C terminus was EEID, which existed in cytosolic Hsp70s of brown algae, Undaria pinnatifida, and diatom, T. pseudonana. There were three degenerate repeats of tetrapeptide GGMP in the amino acid sequence of LJHsp70. In the whole Hsp70 amino acid sequence, the conservation of the N terminus was higher than the C terminus.
Nucleotide and deduced amino acid sequences of Laminaria japonica heat shock protein 70 cDNA. An asterisk below the last three nucleotides indicates a stop codon. Hsp70 signatures motif-1, motif-2, and motif-3, highly repeated sequences of CAA, and degenerate repeat of tetrapeptide GGMP are underlined
The BLAST program analysis showed that the deduced amino acid sequence of the LJHsp70 shared homology with amino acid sequences of other known cytosolic Hsp70s. According to the method and criteria of Renner and Waters (2007), different types of Hsp70s of algae and land plants (mentioned above) were used to construct a phylogenetic tree using the programs ClustalW and Mega 4.0 (Fig. 2). In the phylogenetic tree of Hsp70s, LJHsp70 clustered together with cytosolic Hsp70s. The result of phylogenetic tree of Hsp70s, the BLAST program analysis, and cytosolic Hsp70-specific motif of LJHsp70 verified that the cloned LJHsp70 belonged to cytosolic Hsp70 family. LJHsp70 kept close with cytosolic Hsp70s of brown algae, U. pinnatifida and Fucus serratus, kept relatively close with cytosolic Hsp70s of diatom, green algae, and land plants but were relatively distant from C. merolae (red algae). At present, phylogenetic analysis of other types of Hsp70s (ER Hsp70s, MT Hsp70s, and CP Hsp70s) is difficult because there was limited information about other types of Hsp70s of brown algae and other algae. The relationships displayed in the phylogenic tree were in general agreement with traditional taxonomy.
Phylogenetic tree of heat shock protein 70 family members constructed with the neighbor-joining method. Numbers at each branch indicate the percentage of times a node was supported in 1,000 bootstrap pseudoreplications by neighbor joining. Accession numbers are shown in parentheses. Designated naming system of Hsp70s of T. pseudonana, C. reinhardtii, O. lucimarinus, O. tauri, C. merolae referred to criteria of Renner and Waters (2007)
Expression Levels of LJHsp70 Under Different Heat Shock Temperatures
Real-time quantitative PCR was used to investigate the expression levels of LJHsp70 under various stress conditions. The mRNA expression levels of LJHsp70 under different heat shock temperatures were shown in Fig. 3. The mRNA expression of LJHsp70 could be detected under different temperatures for 1 h, but the mRNA expression levels of LJHsp70 were different. In 5°C, 10°C, or 15°C treatment groups, the expression level of LJHsp70 was relatively low. The expression level of LJHsp70 at 30°C for 1 h was twofold higher than that of treatment group at 10°C for 1 h. However, the expression levels of LJHsp70 of L. japonica sporophyte at 35°C or 40°C for 1 h were lower than those at 25°C or 30°C for 1 h. According to the results of different heat shock temperatures for 1 h, the expression level of LJHsp70 was highest at 30°C for 1 h.
Expression Levels of LJHsp70 at Different Heat Shock Times
In our experiment, juvenile L. japonica sporophyte died when the organism was kept in high temperature (over 30°C) for long time (over 6 h). Thus, the mRNA expression levels of LJHsp70 at 25°C for different times were investigated how different heat shock times affect the expression levels of LJHsp70. The results were shown in Fig. 4. In the experiment, the control group was treated as stress groups except that the control group was kept at 10°C. The expression level of LJHsp70 significantly increased at 25°C for 1 h compared to that of control at 10°C. After that, the expression level of LJHsp70 increased slowly. The expression level reached the peak after heat shock time for 7 h, which was three times higher than that of control at 10°C. After that, the mRNA expression level of LJHsp70 dropped slowly and returned to low expression level after heat shock time for 12 h.
Expression Levels of LJHsp70 Under Different Salt Concentrations
The expression levels of LJHsp70 at different salt concentrations for 2 h were detected, and the results were shown in Fig. 5. The expression level of LJHsp70 at 35‰ or 40‰ salt concentration for 2 h was not significantly different from that at 30‰ salt concentration for 2 h (P < 0.05). Compared to the expression of LJHsp70 at 30‰ salt concentration for 2 h, the expression level of LJHsp70 at salt concentration lower than 25‰ increased significantly (P < 0.05). The expression levels of LJHsp70 at 0‰ or 5‰ salt concentration was almost twofold higher than that at 30‰ salt concentration for 2 h. The results showed that L. japonica had strong stress response to low salt concentration.
Discussion
Many molecular biological researches on macroalgae are hindered because it is difficult to isolate high-quality DNA and RNA from macroalgae, which are rich in phenolics, polysaccharides, and other compounds (Sharma et al. 2002; Joubert and Fleurence 2005). The lack of genomic information on macroalgae is another disadvantaged factor for molecular research in macroalgae (Collén et al. 2007). So far, no full-length cDNA gene in Phaeophyta has been cloned and analyzed. L. japonica is a typical seaweed in Phaeophyta suitable for genetic, physiological, and molecular biological researches.
Organisms, especially for non-mobile organisms such as seaweeds, in nature have adapted to various harsh habitats and developed several mechanisms to withstand environmental stresses, such as behavioral adaptations, morphological changes, physiological regulations, and biochemical and cellular specializations. Hsp70s have received attention in marine organisms as a kind of biomarker of stresses because their expression is highly variable in the presence or absence of stimuli (Dahlhoff 2004; Ireland et al. 2004). Several studies have confirmed the rapid expression of the Hsp70 family in aquatic organisms as a result of osmotic (Smith et al. 1999), heavy metal (Geraci et al. 2004; Piano et al. 2004), and thermal stress (Piano et al. 2002, 2004), but there was little research about the expression levels of macroalgae Hsp70s in the presence of stress factors. The goal of the study was to clone full-length cDNA of cytosolic LJHsp70 and study mRNA expression levels of LJHsp70 in various stress conditions.
The first full-length sequence of cytosolic LJHsp70 cDNA in Phaeophyta was cloned using RT-PCR coupled with 5′ and 3′ RACE. The full-length cDNA of the LJHsp70 gene is 2,918 bp, which encodes a polypeptide of 657 amino acids with several highly conserved functional motifs, such as three conserved Hsp70 family signatures, an N-terminal adenosine triphosphatase (ATPase) domain, a substrate-binding domain, and a highly variable C-terminal domain. It was interesting that in 5′ untranslated region of LJHsp70, there was highly repeated sequence of CAA and rich content of nucleotide C and A, which existed in nucleotide sequence of U. pinnatifida Hsp70 (accession number FJ375361), but did not exist in nucleotide sequences of other Hsp70s. The meaning of highly repeated sequence of CAA in Hsp70 of L. japonica and U. pinnatifida should be further studied. At present, the unique motif for the cytosolic Hsp70 group in three brown algae (L. japonica, U. pinnatifida, and F. serratus) is EEID, which existed in the cytosolic Hsp70 of T. pseudonana. Similar with sequences of other cytosolic Hsp70s, there was degenerate repeat of tetrapeptide GGMP in the amino acid sequence of LJHsp70, and the conservation of the N terminus was higher than the C terminus. The sequence alignment, structure comparison and phylogenetic analysis suggested that LJHsp70 was a member of the cytosolic Hsp70 family.
For nearly all organisms studied, a typical heat shock response pattern has been observed with maximum stress protein expression at 10–15°C above optimum growth temperature. L. japonica usually grows in cold-water zones, and a temperature range from 5°C to 15°C is suitable for its growth. In suitable growth condition, the expression levels of Hsp70s in organisms are always low. Therefore, for a typical heat shock response, maximum LJHsp70 expression would be expected in the range 25–30°C, and this is what was observed in the work. When treatment temperature was higher than normal growth temperature over 15°C, the enzyme-related mRNA synthesis and expression of L. japonica were immediately deactivated or strongly inhibited. As a result, the mRNA expression level of L. japonica sporophyte LJHsp70 at 35°C or 40°C for 1 h was lower than that at 25°C or 30°C for 1 h. Salt concentration is an important factor that affects the physiological condition and metabolism of algae in natural environment (Gimmler 2000; Li and Brawley 2004; Iwamoto and Shiraiwa 2005). In natural environment, L. japonica generally grows in seawater with salt concentration about 30‰, but different rainfall and river flow to sea in different seasons affect the salt concentration of estuaries and offshore where L. japonica inhabits. Thus, L. japonica confronts salt concentration stress during the period of growth. In the study, the mRNA expression levels of LJHsp70 were different at different salt concentrations. Our results suggested that the LJHsp70 gene was expressed in response to thermal stress, heat shock time, and salt concentration challenge and, therefore, may play an important role in the response of stress environment. Therefore, LJHsp70 may be a kind of potential biomarker of stress and can be applied to monitor environment conditions.
It is worth emphasizing that all results presented in this study represent short-term acclimation of L. japonica in laboratory, and the expression of LJHsp70 was affected by more complex natural environmental factors. Due to different components of cells between young and older sporophytes, the expression level of LJHsp70 in young sporophytes may be different from that of older sporophytes.
References
Ananthan J, Goldberg AL, Voellmy R (1986) Abnormal proteins serve as eukaryotic stress signals and trigger the activation of heat shock genes. Science 232:522–524
Barua D, Heckathorn SA (2006) The interactive effects of light and temperature on heat-shock protein accumulation in Solidago altissima (Asteraceae) in the field and laboratory. Am J Bot 93:102–109
Boorstein WR, Ziegelhoffer T, Craig EA (1994) Molecular evolution of the Hsp70 multigene family. J Mol Evol 38:1–17
Collén J, Guisle-Marsollier I, Leger JJ, Boyen C (2007) Response of the transcriptome of the intertidal red seaweed Chondrus crispus to controlled and natural stresses. New Phytol 176:45–55
Dahlhoff EP (2004) Biochemical indicators of stress and metabolism: applications for marine ecological studies. Annu Rev Physiol 66:183–207
Downs CA, Heckthorn SA, Bryan JK, Coleman JS (1998) The methionine-rich low-molecular-weight chloroplast heat shock protein: evolutionary conservation and accumulation in relation to thermotolerance. Am J Bot 85:175–183
Feder ME, Hofmann GE (1999) Heat-shock proteins, molecular chaperones, and the stress response: evolutionary and ecological physiology. Annu Rev Physiol 61:243–282
Fink AL (1999) Chaperone-mediated protein folding. Physiol Rev 79:425–449
Geraci F, Pinsino A, Turturici G, Savona R, Giudice G, Sconzo G (2004) Nickel, lead, and cadmium induce differential cellular responses in sea urchin embryos by activating the synthesis of different Hsp70s. Biochem Biophys Res Commun 322:873–877
Gething MJ, Sambrook J (1992) Protein folding in the cell. Nature 355:33–45
Gimmler H (2000) Primary sodium plasma membrane ATPases in salt-tolerant algae: facts and fictions. J Exp Bot 51:1171–1178
Goodman R, Blank M (1998) Magnetic field stress induces expression of hsp70. Cell Stress Chaperon 3:79–88
Gupta R, Golding G (1993) Evolution of HSP70 gene and its implications regarding relationships between archaebacteria, eubacteria, and eukaryotes. J Mol Evol 37:573–582
Guy CL, Li QB (1998) The organization and evolution of the spinach stress 70 molecular chaperone gene family. Plant Cell 10:539–556
Halpin PM, Sorte CJ, Hofmann GE, Menge BA (2002) Patterns of variation in levels of Hsp70 in natural rocky shore populations from microscales to mesoscales. Integr Comp Biol 42:815–824
Hamdoun AM, Cheney DP, Cherr GN (2003) Phenotypic plasticity of HSP70 and HSP70 gene expression in the pacific oyster (Crassostrea gigas): Implications for thermal limits and induction of thermal tolerance. Biol Bull 205:160–169
Hartl FU (1996) Molecular chaperones in cellular protein folding. Nature 381:571–579
Hartl FU, Hayer-Hartl M (2002) Molecular chaperones in the cytosol: from nascent chain to folded protein. Science 295:1852–1858
Henkel SK, Hofmann GE (2008a) Differing patterns of hsp70 gene expression in invasive and native kelp species: evidence for acclimation-induced variation. J Appl Phycol 20:915–924
Henkel SK, Hofmann GE (2008b) Thermal ecophysiology of gametophytes cultured from invasive Undaria pinnatifida (Harvey) Suringar in coastal California harbors. J Exp Mar Biol Ecol 367:164–173
Hofmann GE, Somero GN (1995) Evidence for protein damage at environmental temperatures—seasonal changes in levels of ubiquitin conjugates and Hsp70 in the intertidal mussel Mytilus trossulus. J Exp Biol 198:1509–1518
Hong SW, Vierling E (2000) Mutants of Arabidopsis thaliana defective in the acquisition of tolerance to high temperature stress. Proc Natl Acad Sci U S A 97:4392–4397
Howarth CJ (1991) Molecular responses of plants to an increased incidence of heat-shock. Plant Cell Environ 14:831–841
Ireland HE, Harding SJ, Bonwick GA, Jones M, Smith CJ, Williams JH (2004) Evaluation of heat shock protein 70 as a biomarker of environmental stress in Fucus serratus and Lemna minor. Biomarkers 9:139–155
Iwamoto K, Shiraiwa Y (2005) Salt-regulated mannitol metabolism in algae. Mar Biol 7:407–415
Jolly C, Morimoto RI (1999) Stress and the cell nucleus: dynamics of gene expression and structural reorganization. Gene Expr 7:261–270
Joubert Y, Fleurence J (2005) DNA isolation protocol for seaweeds. Plant Mol Biol Rep 23:197a–197g
Karlin S, Brocchieri L (1998) Heat shock protein 70 family: multiple sequence comparisons, function, and evolution. J Mol Evol 47:565–577
Lewis S, May S, Donkin ME, Depledge MH (1998) The influence of copper and heat shock on the physiology and cellular stress response of Enteromorpha intestinalis. Mar Environ Res 46:421–424
Lewis S, Donkin ME, Depledge MH (2001) Hsp70 expression in Enteromorpha intestinalis (Chlorophyta) exposed to environmental stressors. Aquat Toxicol 51:277–291
Li QB, Guy CL (2001) Evidence for non-circadian light/dark regulated expression of hsp70s in spinach leaves. Plant Physiol 125:1633–1642
Li R, Brawley SH (2004) Improved survival under heat stress in intertidal embryos (Fucus spp.) simultaneously exposed to hypersalinity and the effect of parental thermal history. Mar Biol 144:205–213
Mager WH, de Boer AH, Siderius MH, Voss HP (2000) Cellular responses to oxidative and osmotic stress. Cell Stress Chaperon 5:73–75
Manitašević S, Dunderski J, Matic G, Tucic B (2007) Seasonal variation in heat shock proteins Hsp70 and Hsp90 expression in an exposed and a shaded habitat of Iris pumila. Plant Cell Environ 30:1–11
Mayer MP, Bukau B (2005) Hsp70 chaperones: cellular functions and molecular mechanism. Cell Mol Life Sci 62:670–684
Morimoto RI, Kline MP, Bimston DN, Cotto JJ (1997) The heat-shock response: regulation and function of heat-shock proteins and molecular chaperones. Essays Biochem 32:17–29
Mycko MP, Cwiklinska H, Walczak A, Libert C, Raine CS, Selmaj KW (2008) A heat shock protein gene (Hsp70.1) is critically involved in the generation of the immune response to myelin antigen. Eur J Immunol 38:1999–2013
Nelson RJ, Ziegelhoffer T, Nicolet C, Werner-Washburne M, Craig EA (1992) The translation machinery and 70 kD heat shock protein cooperate in protein synthesis. Cell 71:97–105
Ohtsuka K, Hata M (2000) Molecular chaperone function of mammalian Hsp70 and Hsp40-a review. Int J Hyperther 16:231–245
Piano A, Asirelli C, Caselli F, Fabbri E (2002) Hsp70 expression in thermally stressed Ostrea edulis, a commercially important oyster in Europe. Cell Stress Chaperon 7:250–257
Piano A, Valbonesi P, Fabbri E (2004) Expression of cytoprotective proteins, Hsp70, and metallothioneins in tissues of Ostrea edulis exposed to heat and heavy metals. Cell Stress Chaperon 9:134–142
Renner T, Waters ER (2007) Comparative genomic analysis of the Hsp70s from five diverse photosynthetic eukaryotes. Cell Stress Chaperon 12:172–185
Rizhsky L, Liang H, Mittler R (2002) The combined effect of drought stress and heat shock on gene expression in tobacco. Plant Physiol 130:1143–1151
Roeder V, Collén J, Rousvoal S, Corre E, Leblanc C, Boyen C (2005) Identification of stress genes from Laminaria digitata (Phaeophyceae) protoplast cultures by expressed sequence tag analysis. J Phycol 41:1227–1235
Sagarin RD, Somero GN (2006) Complex patterns of expression of heat-shock protein 70 across the southern biogeographical ranges of the intertidal mussel Mytilus californianus and snail Nucella ostrina. J Biogeogr 33:622–630
Sharma AD, Gill PK, Singh P (2002) DNA isolation from dry and fresh samples of polysaccharide-rich plants. Plant Mol Biol Rep 20:415a–415f
Smith TR, Tremblay GC, Bradley TM (1999) Hsp70 and a 54 kDa protein (Osp54) are induced in salmon (Salmo salar) in response to hyperosmotic stress. J Exp Zool 284:286–298
Snyder MJ, Girvetz E, Mulder EP (2001) Induction of marine mollusc stress proteins by chemical or physical stress. Arch Environ Contam Toxicol 41:22–29
Sørensen JG, Loeschcke V (2001) Larval crowding in Drosophila melanogaster induces hsp70 expression, and leads to increased adult longevity and adult thermal stress resistance. J Insect Physiol 47:1301–1307
Sung DY, Kaplan F, Guy CL (2001) Plant Hsp70 molecular chaperones: protein structure, gene family, expression and function. Physiol Plant 113:443–451
Suzuki S, Furuya K, Takeuchi I (2006) Growth and annual production of the brown alga Laminaria japonica (Phaeophyta, Laminariales) introduced into the Uwa Sea in southern Japan. J Exp Mar Biol Ecol 339:15–29
Tanaka KI, Namba T, Arai Y, Fujimoto M, Adachi H, Sobue G, Takeuchi K, Nakai A, Mizushima T (2007) Genetic evidence for a protective role for heat shock factor 1 and heat shock protein 70 against colitis. J Biol Chem 282:23240–23252
Tomanek L, Sanford E (2003) Heat-shock protein 70 (HSP70) as a biochemical stress indicator: an experimental field test in two congenic intertidal gastropods (genus: Tegula). Biol Bull 205:276–284
Vayda ME, Yuan ML (1994) The heat shock response of an Antarctic alga is evident at 5°C. Plant Mol Biol 24:229–233
Verbeke P, Fonager J, Clark BC, Rattan SIS (2001) Heat shock response and ageing: mechanisms and applications. Cell Biol Intern 25:845–857
Wagner M, Hermanns I, Bittinger F, Kirkpatrick CJ (1999) Induction of stress proteins in human endothelial cells by heavy metal ions and heat shock. Am J Physiol 277:L1026–L1033
Wickner S, Mauriz MR, Gottesman S (1999) Posttranslational quality control: folding, refolding and degrading proteins. Science 286:1888–1893
Yao JT, Fu WD, Wang XL, Duan DL (2009) Improved RNA isolation from Laminaria japonica Aresch (Laminariaceae, Phaeophyta). J Appl Phycol. doi:10.1007/s10811-008-9354-0
Acknowledgments
This work was supported by the Shandong Agriculture Seedstock Breeding Project, knowledge Innovation Program of the Chinese Academy of Sciences (KSCX2-YW-N-47-02), and the CAS/SAFEA International Partnership Program for Creative Research Teams (Research and Applications of Marine Functional Genomics) for financial assistance. The author thanks anonymous reviewers for the critical comments and suggestions for the manuscript.
Author information
Authors and Affiliations
Corresponding author
Rights and permissions
About this article
Cite this article
Fu, W., Yao, J., Wang, X. et al. Molecular Cloning and Expression Analysis of a Cytosolic Hsp70 gene from Laminaria japonica (Laminariaceae, Phaeophyta). Mar Biotechnol 11, 738–747 (2009). https://doi.org/10.1007/s10126-009-9188-z
Received:
Accepted:
Published:
Issue Date:
DOI: https://doi.org/10.1007/s10126-009-9188-z