Abstract
Adult mice emit many ultrasonic vocalizations (USVs) during social interaction tasks, but only a few studies have yet reported USVs in stressed adult mice. Our aim was to study which experimental conditions favor USV emission during behaviors associated with different emotional states. As USVs likely mediate social communication, we hypothesized that temporary social isolation followed by exposure to a novel social congener would promote USV emission. USVs were recorded in three different behavioral paradigms: restraint, free moving in a new environment, and during a social interaction task. We compared USV emission, with or without the presence of a social congener, in animals socially isolated during different periods (0, 6 or 21 days). Social isolation decreased the number of USVs during free moving, whereas it increased during restraint. During the social interaction task, animals produced high-frequency USVs (median: 72.6 kHz, 25–75% range: 67.6–78.2 kHz), especially when the social partner was active and social motivation was high. During restraint, presence of a social congener increased the call rate of low-frequency USVs (median: 52.4 kHz, 25–75% range: 44.8–56.5 kHz). USV frequency followed two unimodal distributions that distinguished low-frequency USVs (≤ 60 kHz) mainly emitted during free-moving (90.9% of total USVs) and restraint (93.1%) conditions, from high-frequency USVs (> 60 kHz) mainly emitted during the social interaction task (85.1% of total USVs). The present study confirms that USV call rate and frequency depend on behavioral states, and provides evidence that the presence of a congener promotes ultrasonic vocalizations in restrained adult mice.
Article Highlights
-
Social isolation increased restraint-induced USVs, but decreased USVs induced by free-exploration of a novel environment.
-
Social contact increased call rates of high-frequency USVs (> 60 kHz), especially when social partner was active and social motivation was high.
-
Restraint induced low-frequency USVs (≤ 60 kHz) in mice, especially when a social congener was in the vicinity.
Similar content being viewed by others
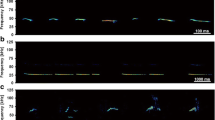
Avoid common mistakes on your manuscript.
Introduction
Ultrasonic vocalizations (USVs) are emitted by rodents in different emotionally relevant contexts. An aversive context often induces low-frequency USVs (22 kHz for rats; 30–60 kHz for mice), whereas a rewarding context promotes high-frequency USVs (50 kHz for rats; 60–100 kHz for mice) (Kaltwasser 1991; Blanchard et al. 1991; Brudzynski and Ociepa 1992; Vivian and Miczek 1993a; Borta et al. 2006; Scattoni et al. 2009; Chabout et al. 2012; Brudzynski 2013; Grimsley et al. 2016; Simola and Granon 2018) even though USV frequencies from mice showed less clearer separation than for rats. The effects of stress on USV emission have mainly been recorded in rats, and vary as a function of age (pup, adolescent, adult), stressor intensity (from mild to traumatic), duration (acute versus chronic), and modality (physical, nociceptive, or psychosocial stressor). For example, several studies have shown that maternal stress upon separation from rat pups (i.e., “separation or distress calls”) increase USVs in the range of 30–50 kHz (Blanchard et al. 1991; Litvin et al. 2007; Laloux et al. 2012). Other studies have demonstrated how physical and nociceptive stressors in adult rats, such as electrical foot shocks (Tonoue et al. 1986; Cuomo et al. 1988; Borta et al. 2006; Hegoburu et al. 2009), painful stimuli (Kurejova et al. 2010), and exposure to cold water, loud sounds (Kaltwasser 1991) or air puffs (Naito et al. 2003) can all increase the emission of 22 kHz USVs. Psychosocial stressors have also been shown to induce 22 kHz vocalizations in response to aggressive social interaction using the resident–intruder test (Vivian and Miczek 1993b), and predator exposure (Blanchard et al. 1986). Pharmacological studies using anxiolytics (benzodiazepines and serotonergic compounds), antidepressants (SSRI and tricyclic molecules), and mGluR5 or CRF receptor antagonists have depicted decreased anxiety reactivity in behavioral tasks, and associated decreased stress-induced 22 kHz vocalizations in rats (see Simola 2015 for review).
Stress-induced vocalizations have been proposed as a way to model an anxiety-like state in rats, with USVs as a promising behavioral measure for screening new drugs in the field of anxiety and stress-related disorders (Sánchez 2003; Simola 2015). In contrast, limited data are available in stressed adult mice, as reviewed recently (Simola and Granon 2018; see however Lumley et al. 1999; Chabout et al. 2012; Mun et al. 2015; Grimsley et al. 2016), even though they emit a large number of USVs during free-moving behavior in a novel environment and social interaction tasks (Scattoni et al. 2011; Chabout et al. 2012; Grimsley et al. 2016). In addition, because USVs can be non-invasively and repeatedly measured, they can be proposed as a valuable ethological measure of an animal’s well-being. It is therefore important to determine which experimental conditions favor their emission in stressed adult mice.
The principal aim of this study was to develop a better understanding of how behavior correlates with the ultrasonic vocalizations of mice. Previously, restraint was associated with anxiety-like behavior and an increase in plasma and central stress-induced corticosterone levels (Chauveau et al. 2012; Nosjean et al. 2015; Grimsley et al. 2016). This restraint-induced aversive state is linked to avoidance behavior (open arms in the elevated plus maze). In contrast, social interaction has been shown to induce approach behavior (for social contact), and is probably associated with a reward state (Panksepp and Lahvis 2007; Ben-Ami Bartal et al. 2011; Chabout et al. 2013). Therefore, we compared USV emission relative to restraint and social interaction, because these behaviors have been associated with opposite emotional states, i.e., aversion versus motivation, respectively.
Free-moving behavior in a novel environment was also studied due to its conflicting or ambiguous nature with both aversive (potential risk of dangers, predators, etc.) and rewarding (potential benefit of new food, congeners, etc.) components. USVs were recorded during the three behavioral conditions, and a quantitative analysis (number of USVs/minute and their mid-point frequency) was performed. Although USV functions are not consistently understood, it is generally acknowledged that they convey an individual’s characteristics (age, sex, body size), emotional states, and/or social status. Mice emit vocalizations particularly in the presence of social congeners or social olfactory cues (Holy and Guo 2005; Grimsley et al. 2011; Chabout et al. 2012; Ey et al. 2013). Therefore, the second aim of the present study was to address how social context influences USV emission in adult male mice during restraint and free-moving behavior. For this, we compared USV emission in socially isolated animals during different delays (with social isolation durations of 0, 6 or 21 days) with or without the presence of a social congener. Considering that one purpose of USVs is to sustain social communication, we hypothesized that social isolation and the presence of a social congener modulates the quantity and/or quality of USVs emitted.
Materials and methods
Animals
C57Bl/6J male mice (n = 122, obtained from Charles River) were 56 days old at arrival in the animal facility and were 120–150 days old during behavioral testing. Mice were maintained in cages with 3–4 animals per cage for 5 weeks before being housed individually (see the protocols for social isolation duration below). All animals received standard food and water ad libitum. A circadian light cycle of 12 h light/12 h dark (on at 8:00 a.m.) was maintained in the animal facility. All experiments were performed during the light cycle, between 09:00 a.m. and 5:00 p.m. All procedures were approved by an independent Institutional Animal Care and Use Committee (CEEA Paris centre et sud n°59; protocol number Granon 2015-04).
Free-moving behavior and the social interaction task (SIT)
Free-moving behavior and the social interaction task (SIT) took place in a transparent Plexiglas cage (50 cm × 25 cm × 30 cm deep) exposed to a diffuse 100 lx light. To determine the effect of social motivation on USV emission, mice were tested in the SIT after 0 (SIT0; basic social motivation), 6 (SIT6; moderate level of social motivation) or 21 (SIT21; high level of social motivation) days of social isolation. The test mouse, referred to as the ‘isolated host’ mouse, was placed in the SIT cage for 30 min of novel free-moving behavior. We recorded USVs for the first 8 min as an index of this behavior. After the 30-min habituation period, another mouse of the same age, sex, and strain reared in social cages, referred to as the ‘social visitor’, was gently placed in the box for an 8-min social interaction, and USVs were recorded from the two mice. The duration of social contact between the two conspecifics was manually scored for 8 min. All social contact subtypes were included in the analysis (oro-oral, oro-flank, oro-genital, flank–flank, and genital–genital). To determine the contribution of the active social visitor, USVs from the test mouse were also analyzed while alone in front of a mirror or when confronted with an anesthetized congener (passive visitor; ANEST group). In these two cases, the host mouse had been previously isolated for 21 days.
Restraint
Animals were restrained so as to prevent mobility for 8 min in a 50 ml Falcon® tube that was perforated at the end (1 cm diameter hole) to allow ventilation and USV recordings. Four conditions were designed to determine the effect of a social congener presence after 8 min of solitary restraint. The test mouse either remained alone (condition 1), or was confronted by: a social visitor behind a transparent solid plexiglas wall (10 cm from the restraint tube; distal condition 2); a social visitor under a basket (9 cm in diameter × 10 cm high) perforated with a 1 cm hole (5 cm from the restraint tube; proximal condition 3); or a visitor that was free to explore within a Plexiglas cage (50 cm × 25 cm × 30 cm deep; condition 4).
USV recording and analysis
Condenser ultrasound microphones (Polaroid/CMPA) were placed above the test mouse (7 cm above the SIT chamber or 5 cm in front of the restraint tube) and close to the social visitor for conditions 2 and 3. Microphones were connected to an UltraSoundGate 416H ultrasound recording interface plugged into a computer equipped with the Avisoft Recorder USG recording software (sampling frequency: 250 kHz; low pass filter: 125 kHz; Avisoft Bioacoustics, Berlin, Germany). Spectrograms were generated with the following characteristics: FFT length: 1024 points; 16-bit format; Blackman window, overlap: 87.5%, time resolution: 0.512 ms, frequency resolution: 244 Hz.
We manually categorized ten USV subtypes based on their duration and frequency modulation extracted from the waveform pattern: (1) short: ≤ 50 ms and ≤ 10 kHz frequency modulation; (2) flat: ≥ 50 ms and ≤ 10 kHz frequency modulation; (3) one frequency jump: instantaneous frequency step, like a vertical discontinuity with no time gap; (4) multiple frequency jumps: multiple instantaneous frequency step; (5) U: U shape wave ≥ 10 kHz frequency modulation; (6) chevron: inverted U shape ≥ 10 kHz frequency modulation; (7) modulated: ≥ 10 kHz of modulation, with several decreases and increases in frequency; (8) composite: two or more components emitted simultaneously; (9) upward: continuous increase in peak frequency ≥ 10 kHz frequency modulation; (10) downward: continuous decrease in peak frequency ≥ 10 kHz frequency modulation.
USV was isolated by visualizing spectrograms generated by Avisoft (Avisoft Bioacoustics, Berlin, Germany) and parameters such as duration, minimum–maximum frequencies (20–100 kHz range) were extracted. USV call rate was expressed as the mean number of USVs/minute ± s.e.m. For each USV, the mid-point of the min–max frequency values was calculated; for each animal/group of animals, USV frequencies (kHz) were expressed as a median [25% percentile; 75% percentile].
Statistical analyses
The distribution of each variable (call rate, frequency and social contacts) was tested using the Kolmogorov–Smirnov normality test, and then variables were analyzed using a (paired) Student’s t test or analysis of variance (ANOVA) followed by a Bonferroni post hoc test. Mean ± standard error mean (s.e.m.) was used for the graphical representation of the results. Statistical thresholds were set with p values < 0.05; non-significant results are written as ns. Degrees of freedom for the t test are written as df. The Mann–Whitney U test was used for the frequency analysis of raw data. The 30–100 kHz frequency distribution was plotted using a 10 kHz bin width and extra sum-of-squares F tests were used to compare the distribution of the fitting curve.
Results
Social isolation effect on USV call rate
The duration of social isolation was studied by comparing USV emissions of non-isolated animals, animals isolated for 6 days, and animals isolated for 21 days in both free-moving (three independent groups; left bar graph) and restrained conditions (three independent groups; right bar graph Fig. 1). During free-moving behavior, the number of USVs was altered when considering the main independent factor “duration of social isolation” (one-way ANOVA, F(2,25) = 14.04, p < 0.0001). The USV rate during free-moving behavior of isolated mice was lower than for non-isolated animals (Bonferroni post hoc test: p < 0.001 and p < 0.01, respectively, as compared to 6 and 21 days of social isolation). In contrast, the duration of social isolation increased USV emissions in mice during the restraint condition (two-way ANOVA, F(2,23) = 14.24, p < 0.0001), with 21-day isolated animals vocalizing more than the other groups (p < 0.001 and p < 0.01, respectively, with 0 and 6 days of social isolation). Comparing both conditions, animals vocalized more in the free-moving condition than during restraint (two-way time × condition ANOVA: F(2,44) = 17.52, p < 0.0001; duration of isolation factor: F(2,44) = 71.11, p < 0.001; condition factor: F(1,44) = 15.57, p < 0.001). More precisely, animals vocalized more in the free-moving condition than during restraint following a period of no social isolation (Bonferronni post hoc test: p < 0.01). In addition, after 6 days of social isolation, mice vocalized more in the restraint condition than while free moving (p < 0.02). No differences in the USV call rate were found 21 days after social isolation (free moving versus restraint, ns).
Effect of social isolation on USV call rate. Mice were either free moving (left, bar graph) or restrained (right) for 8 min. Data are presented as means ± s.e.m. for USV call rate (number of USVs/minute). n values: 8, 8 and 10 for the free-moving condition (0, 6 and 21 days of social isolation, respectively); n = 9, 9 and 6 for the restrained condition. Bonferroni post hoc test: *p < 0.05; **p < 0.01; ***p < 0.001
Rapid habituation effect on USV call rate
Next, we quantified the amount of USVs emitted during the first 4 min (Fig. 2) and the last 4 min of 21-day isolated animals. USV rates significantly decreased with time, both during free-moving behavior (paired t test first 4 min versus last 4 min, t = 9.83, df = 9, p < 0.0001) and restraint (t = 11.52, df = 9, p < 0.0001), suggesting a habituation effect for USV emission in both conditions. No differences were found between the free-moving and restraint conditions in the time course of USV emission (two-way time × condition ANOVA: F(2,38) = 0.13, ns; time effect: F(1,38) = 221.80, p < 0.0001) although a significant condition effect was observed (one-way ANOVA condition effect: F(1,38) = 7.24, p < 0.02). Post hoc analysis showed that mice vocalized more during restraint in the 4–8 min period than during free moving (p < 0.0001). Nevertheless, the absolute number of calls emitted in the last 4 min in both conditions was very low.
The USV call rate undergoes rapid habituation. USV call rate (number of USVs/minute) during the first (white bars) and last 4 min (black bars) of free-moving and restraint conditions in 21-day isolated animals. n values: 10 for both conditions. Data are presented as means ± s.e.m. **p < 0.01; ***p < 0.001 for the paired t test (first 4 min versus last 4 min)
Social effect on USV call rate during the free-moving condition
Next, we sought to determine the role of social contact in USV emission during free social interaction. For this, social contacts and USV emissions were analyzed when a social partner was either represented by a self-mirroring presentation (“MIRROR 21” condition), an inactive congener (anesthetized visitor; “ANEST 21”), or an active partner (Fig. 3). Different levels of social motivation were tested using the prior social isolation duration of 0, 6 or 21 days. Contact time analysis revealed a significant group effect (one-way ANOVA: F(4,51) = 52.15, p < 0.0001). More precisely, mice interacted more with the anesthetized social partner than any other social visitor (post hoc test: p < 0.0001 for all comparisons). With the active social partner (SIT protocols), the time spent in social contact increased with the duration of the tested host’s social isolation (comparison with SIT0: p < 0.001 for SIT6 and SIT21). USV rates also showed a significant group effect (F(4,46) = 11.88, p < 0.0001). Mice from the SIT21 group emitted significantly more USVs than all other groups (post hoc tests: p < 0.001, p < 0.001, p < 0.05 and p < 0.01 for MIRROR 21, ANEST 21, SIT0 and SIT6, respectively).
USV call rate in free-moving animals depends on the state of the social visitor. Social visitors were either self-mirrored (the “MIRROR 21” group), anesthetized (passive; “ANEST 21” group) or actively interacting with an isolated host (different durations of social isolation SIT for 0, 6, or 21 days) for 8 min. Tested host mice in the anesthetized and mirrored conditions had been isolated for 21 days. Data are presented as 8-min means ± s.e.m. for contact time (left) and number of USVs/min (right). Contact time was measured as the time spent in physical contact with the mirror or the social congener. For clarity, only Bonferroni post hoc analyses including the SIT0 group as a reference are presented: *p < 0.05; **p < 0.01; ***p < 0.001. n values: 12, 12, 9, 8 and 11 for the MIRROR 21, ANEST, SIT0, SIT6, and SIT21 conditions, respectively
To conclude, we observed an increase in USV emission during social interaction only when the social partner was active and social isolation lasted for at least 21 days.
Effect of a congener on USV call rate during restraint
The following protocol was designed to determine whether the vicinity of a social partner could influence USV emission in a restrained mouse. We compared USV emission of the same restraint mice in different conditions where a social visitor was either absent, active (behind a wall; distal condition), visible (under a basket; proximal condition), or free to explore (Fig. 4). One-way analysis of variance with repeated measure indicated a significant effect of the conditions on USV rate (F(4,39) = 5.05, p < 0.01). More precisely, mice vocalized more in the proximal condition when the visitor was under a basket or was free to explore than when the visitor was absent (Dunnett’s multiple comparison post hoc test using “Visitor absent” as the control group: p < 0.05 and p < 0.01, respectively). However, there was no difference in the call rate when the visitor was absent or behind a wall.
USV emission increases during restraint when a social visitor is in the vicinity. The social visitor was either absent, behind a wall, under a basket, or free to explore and the same restraint mouse was recorded after 6 days of social isolation. The number of USVs/minute) is represented by mean ± s.e.m. Dunnett’s multiple comparison post hoc test using “Visitor absent” as control group: p < 0.05 and p < 0.01, respectively: *p < 0.05; **p < 0.01. n values: n = 10
USV frequency distribution as a function of behavioral state
The global one-way ANOVA of median frequency did not reach the statistical level (Kruskal–Wallis value; W = 6.94, ns). However, mice during the free-moving condition alone or in front of the mirror and restraint vocalized mostly at low frequencies (median 52.1 kHz, 25–75% range: 44.3–58.1 kHz; median: 55,5 kHz, 25–75% range: 49,2–67,7 kHz; median: 52.4 kHz, 25–75% range: 44.8–56.5 kHz, respectively). In contrast, both groups from social interaction task (SIT) vocalized at higher frequencies (median: 72.6 kHz, 25–75% range: 67.6–78.2 kHz and median: 70.0 kHz, 25–75% range: 62.1–74.5 kHz for the SIT- and ANEST 21 groups, respectively). Median frequencies of the SIT group were higher than the free-moving (U = 2939, p < 0.0001), mirror (U = 8175, p < 0.0001), and restrained groups (U = 4194, p < 0.0001). Median frequencies of the SIT–ANEST group were higher than the free-moving (U = 1471, p < 0.0001), mirror (U = 2722, p < 0.0001) and restrained groups (U = 2754, p < 0.0001). Median frequencies of the free-moving versus restrained groups were not different (U = 1151, NS), whereas the SIT group vocalized at a higher frequency than the SIT–ANEST group (U = 3392, p < 0.0001).
Next, we plotted USV frequency distribution (30–100 kHz; 10 kHz bin width) as a function of behavioral state (Fig. 5). The Gaussian-logarithmic curve was the best predictor of the data (R2 = 0.97, 0.96 and 0.99, respectively, for the free moving, restrained, SIT groups). Values including width, center, and amplitudes showed that the frequency distribution of the SIT group significantly differed from the free-moving (F(3,10) = 137.0, p < 0.0001) and restrained groups (F(3,10) = 143.5, p < 0.0001). In contrast, the frequencies were equally distributed between the free-moving and restrained groups (F(3,10) = 0.04, ns). Analysis of USV frequency showed two unimodal distributions separating low-frequency USVs (≤ 60 kHz), which were mainly emitted during the free-moving (90.9% of total USVs) or restraint (93.1%) states, from high-frequency USVs (> 60 kHz), which were mainly emitted during the social interaction task (85.1% of total USVs).
USV frequency distribution as a function of behavioral state. USV frequency distributions were plotted using a 10 kHz bin width with the percentage of USVs for 330 (free moving), 720 (restrained), and 1359 (SIT) calls. Data for animals from the SIT-ANEST 21 and MIRROR 21 groups were not plotted so as to improve the clarity of the figure. For each behavioral state, the best non-linear (log-Gaussian) distribution is plotted, showing that the distribution of USV frequencies of SIT group is significantly different between both restrained and free-moving mice (p < 0.0001 for both comparisons)
Discussion
Our results demonstrate that USVs are emitted by adult mice during restraint and free moving in a new environment, which are behavioral conditions associated with increased arousal. USV emission decreased drastically after 4 min, suggesting a rapid habituation effect in both conditions. The rearing social condition (i.e., social group versus social isolation for various durations) differentially altered USV call rate by decreasing it during free moving while increasing it during restraint. Social contact increased the call rate of high-frequency USVs (median: 72.6 kHz, 25–75% range: 67.6–78.2 kHz) during free moving, especially when the social partner was active and social motivation was high (i.e., longer social isolation). Restraint primarily induced low-frequency USV emission (median: 52.4 kHz, 25–75% range: 44.8–56.5 kHz), particularly when a social congener was both active and in the vicinity.
It has been suggested in the literature that adult mice will not produce USVs during aversive situations such as physical restraint or electric foot shock (Portfors and Perkel 2014), even though multiple studies have reported stress-induced USVs (Lumley et al. 1999; Chabout et al. 2012; Mun et al. 2015; Grimsley et al. 2016). The present data confirm that restrained adult mice significantly emit USVs, although in modest quantities. Indeed, despite a low call rate and a rapid habituation, all experimental mice emitted USVs. Our analysis of frequency distribution revealed that most of the USVs were below or equal to 60 kHz during restraint (93.1% of total USVs) and free-moving (90.9% of total USVs) states. Previously, restraint was shown to induce anxiety-like behavior in the elevated plus maze (Chauveau et al. 2012), while free-moving behavior increased stress-induced corticosterone levels up to the level of restrained animals (Nosjean et al. 2015). Low-frequency USVs are likely to reflect both the excitement of novel exploration as well as the aversive state that can be associated with an unpleasant or anxiogenic situation such as restraint. Our data revealed that differences in experimental paradigms between studies could explain the discrepancy with the literature. Indeed, since USV emission shows a rapid and consistent decrease after the first 4 min of novel exposure, it is possible that some studies have failed to detect fleeting USVs. The high level of background noise and the low-power feature of ultrasound represent critical factors in USV analyses (Kurejova et al. 2010). To lower the noise, we used Plexiglas boxes in a dedicated low-noise recording chamber. The present data confirm that USV emission in the mouse depends on the novelty effect (i.e., habituation after the first 4 min), probably via an increase in the phasic and rapid arousal mechanisms that are known to be involved in the response to aversive situations. In contrast to one study that used CBA/CaJ mice (Grimsley et al. 2016), audible vocalizations (below 20 kHz) were not recorded here using C57Bl/6J mice, suggesting that USVs might be a more relevant marker of behavioral state for genetic studies using different strains of mice (Faure et al. 2017).
Classically, stressors such as electric foot shocks or restraint are administered to a mouse that is alone in the apparatus. Our data suggest that producing USVs without any conspecifics in the vicinity might not be so relevant for mice, since placing a congener in the vicinity increases USV calls, likely because of an increase in arousal. Indeed, social context is a crucial factor in the emission of rodent USVs (Chabout et al. 2012; Seffer et al. 2014; Saito et al. 2016). The present data confirm the pro-social feature of USVs in restrained mice since call rate, contrary to median frequency, increased when social motivation was high (i.e., with increasing length of social deprivation Fig. 2) and when a social congener was in the vicinity. Interestingly, social presence increased the amount of restraint-induced USVs when the social congener was proximal (under a basket), but not when it was distal (behind a wall). In other words, these data affirm that seeing a conspecific is not sufficient. One plausible hypothesis is that the basket might additionally allow social olfactory cues through the holes, whereas the wall does not allow it. Further experiments are required to verify this hypothesis. Testing social proxy using animals from the same home cage versus another cage could also be interesting for studying the social relevance of USVs emitted during restraint. One weakness of this study is the lack of certainty regarding the origin of USVs, as we are not yet capable of determining which individual produced the USVs. However, several points allow us to suggest that the recorded USVs originate from the restraint animal: first, we never obtained any USVs in mice placed alone under the basket or behind a wall in our pilot studies; and second, the position of the microphone favored the recording of USVs originating from the restrained mouse.
Despite conflicting results across different strains and a lack of a clear categorical separation of mice USV frequencies, previous reports have shown that exploration of a new context increases low-frequency USV emission (< 60 kHz) in mice, mainly occurring during supported rearing behavior (Chabout et al. 2012; Mun et al. 2015). High-frequency USVs (> 60 kHz) have mostly been detected during walking, unsupported rearing behavior (Mun et al. 2015), and social contact (Chabout et al. 2012). The present data confirm that free moving in a novel environment favors low-frequency USV emission (mostly ≤ 60 kHz), and probably reflects an aversive emotional state such as that observed during restraint (Chauveau et al. 2012). Social interaction is known to provoke USV emission in mice, especially during physical contact (Chabout et al. 2012). Therefore, we asked whether physical contact itself was sufficient to increase the emission of USVs using a mirror image (i.e., a self-moving visual stimulus) or an inactive anesthetized social congener (i.e., unresponsive physical contact). Our data showed that social contact increased the call rate of high-frequency USVs (> 60 kHz), representing 85.1% of total USVs. This increase in USV call rate during the social interaction task was observed only when the social partner was active and social motivation was high (i.e., following 21 days of social isolation Fig. 3), confirming that animals have to be motivated to engage in social contact, and must be rewarded by such contact to emit calls (Panksepp and Lahvis 2007). This social reward, as indexed by the high-frequency USVs, seems to be obtained when the mouse receives a real physical and/or vocal response, but not by the simple perception of a social partner’s motion or odor. Analysis of USV mean frequencies showed a bimodal distribution dissociating low-frequency USVs (≤ 60 kHz), which were mainly emitted during free moving in a novel environment or restraint, from high-frequency USVs (> 60 kHz), which were mainly emitted during the social interaction task. Previous studies demonstrated that the calling rate of mice and the proportion of the different USV types emitted differed depending on the context (mating, isolation or restraint) (Chabout et al. 2015; Grimsley et al. 2016). The present data also confirm a previous study showing that the mean dominant frequency is lower during restraint (48.8 kHz) than mating (64.6 kHz; Grimsley et al. 2016). These authors also demonstrated that mice emitted a higher proportion of low-frequency harmonics during restraint stress below 20 kHz, suggesting a different vocal repertoire between the C57/Bl6J (here) and CBA/CaJ mice strains (Grimsley et al. 2016).
Overall, the present experimental work suggests that a congener in the vicinity is required to increase the quantity of USVs in restrained and free-moving adult mice. The median frequency of USVs qualitatively dissociated the behavioral states: low-frequency USVs (≤ 60 kHz) were mostly produced during restraint and free moving in a novel environment, most likely reflecting an aversive state. In contrast, high-frequency USVs (> 60 kHz) were mainly emitted during approach behavior towards an active congener, possibly due to the rewarding property of reciprocal social contact.
The ability to promote USV emission during a behavioral procedure creates an interesting opportunity to dissociate aversive and reward states, using a non-invasive and repeatable behavioral measure in mice. Although pharmacological studies are now needed, recording USVs might be a useful means to refine this animal model for the study of stress-related brain disorders, and animal welfare.
References
Bartal I, Decety J, Mason P (2011) Empathy and pro-social behavior in rats. Science 334:1427–1430. https://doi.org/10.1126/science.1210789
Blanchard RJ, Flannelly KJ, Blanchard DC (1986) norvegicus. J Comp Psychol 100:101–107
Blanchard RJ, Blanchard DC, Agullana R, Weiss SM (1991) Twenty-two kHz alarm cries to presentation of a predator, by laboratory rats living in visible burrow systems. Physiol Behav 50:967–972
Borta A, Wöhr M, Schwarting RKW (2006) Rat ultrasonic vocalization in aversively motivated situations and the role of individual differences in anxiety-related behavior. Behav Brain Res 166:271–280. https://doi.org/10.1016/j.bbr.2005.08.009
Brudzynski SM (2013) Ethotransmission: communication of emotional states through ultrasonic vocalization in rats. Curr Opin Neurobiol 23:310–317. https://doi.org/10.1016/j.conb.2013.01.014
Brudzynski SM, Ociepa D (1992) Ultrasonic vocalization of laboratory rats in response to handling and touch. Physiol Behav 52:655–660
Chabout J, Serreau P, Ey E et al (2012) Adult male mice emit context-specific ultrasonic vocalizations that are modulated by prior isolation or group rearing environment. PLoS ONE ONE 7:e29401. https://doi.org/10.1371/journal.pone.0029401
Chabout J, Cressant A, Hu X et al (2013) Making choice between competing rewards in uncertain vs. safe social environment: role of neuronal nicotinic receptors of acetylcholine. Front Hum Neurosci 7:468. https://doi.org/10.3389/fnhum.2013.00468
Chabout J, Sarkar A, Dunson DB, Jarvis ED (2015) Male mice song syntax depends on social contexts and influences female preferences. Front Behav Neurosci. https://doi.org/10.3389/fnbeh.2015.00076
Chauveau F, Lange MD, Jungling K et al (2012) Prevention of stress-impaired fear extinction through neuropeptide s action in the lateral amygdala. Neuropsychopharmacology 37:1588–1599. https://doi.org/10.1038/npp.2012.3
Cuomo V, Cagiano R, De Salvia MA et al (1988) Ultrasonic vocalization in response to unavoidable aversive stimuli in rats: effects of benzodiazepines. Life Sci 43:485–491
Ey E, Torquet N, Le Sourd A-M et al (2013) The autism ProSAP1/Shank2 mouse model displays quantitative and structural abnormalities in ultrasonic vocalisations. Behav Brain Res 256:677–689. https://doi.org/10.1016/j.bbr.2013.08.031
Faure A, Pittaras E, Nosjean A et al (2017) Social behaviors and acoustic vocalizations in different strains of mice. Behav Brain Res 320:383–390. https://doi.org/10.1016/j.bbr.2016.11.003
Grimsley JMS, Monaghan JJM, Wenstrup JJ (2011) Development of social vocalizations in mice. PLoS ONE ONE 6:e17460. https://doi.org/10.1371/journal.pone.0017460
Grimsley JMS, Sheth S, Vallabh N et al (2016) Contextual modulation of vocal behavior in mouse: newly identified 12 kHz “Mid-Frequency” vocalization emitted during restraint. Front Behav Neurosci 10:38. https://doi.org/10.3389/fnbeh.2016.00038
Hegoburu C, Sevelinges Y, Thevenet M et al (2009) Differential dynamics of amino acid release in the amygdala and olfactory cortex during odor fear acquisition as revealed with simultaneous high temporal resolution microdialysis. Learn Mem 16:687–697
Holy TE, Guo Z (2005) Ultrasonic songs of male mice. PLoS Biol 3:e386. https://doi.org/10.1371/journal.pbio.0030386
Kaltwasser MT (1991) Acoustic startle induced ultrasonic vocalization in the rat: a novel animal model of anxiety? Behav Brain Res 43:133–137
Kurejova M, Nattenmüller U, Hildebrandt U et al (2010) An improved behavioural assay demonstrates that ultrasound vocalizations constitute a reliable indicator of chronic cancer pain and neuropathic pain. Mol Pain 6:18. https://doi.org/10.1186/1744-8069-6-18
Laloux C, Mairesse J, Van Camp G et al (2012) Anxiety-like behaviour and associated neurochemical and endocrinological alterations in male pups exposed to prenatal stress. Psychoneuroendocrinology 37:1646–1658. https://doi.org/10.1016/j.psyneuen.2012.02.010
Litvin Y, Blanchard DC, Blanchard RJ (2007) Rat 22 kHz ultrasonic vocalizations as alarm cries. Behav Brain Res 182:166–172. https://doi.org/10.1016/j.bbr.2006.11.038
Lumley LA, Sipos ML, Charles RC et al (1999) Social stress effects on territorial marking and ultrasonic vocalizations in mice. Physiol Behav 67:769–775
Mun H-S, Lipina TV, Roder JC (2015) Ultrasonic vocalizations in mice during exploratory behavior are context-dependent. Front Behav Neurosci 9:316. https://doi.org/10.3389/fnbeh.2015.00316
Naito H, Nakamura A, Inoue M, Suzuki Y (2003) Effect of anxiolytic drugs on air-puff-elicited ultrasonic vocalization in adult rats. Exp Anim 52:409–414
Nosjean A, Cressant A, de Chaumont F et al (2015) Acute stress in adulthood impoverishes social choices and triggers aggressiveness in preclinical models. Front Behav Neurosci. https://doi.org/10.3389/fnbeh.2014.00447
Panksepp JB, Lahvis GP (2007) Social reward among juvenile mice. Genes Brain Behav 6:661–671. https://doi.org/10.1111/j.1601-183X.2006.00295.x
Portfors CV, Perkel DJ (2014) The role of ultrasonic vocalizations in mouse communication. Curr Opin Neurobiol 28:115–120. https://doi.org/10.1016/j.conb.2014.07.002
Saito Y, Yuki S, Seki Y et al (2016) Cognitive bias in rats evoked by ultrasonic vocalizations suggests emotional contagion. Behav Processes. https://doi.org/10.1016/j.beproc.2016.08.005
Sánchez C (2003) Stress-induced vocalisation in adult animals. A valid model of anxiety? Eur J Pharmacol 463:133–143
Scattoni ML, Crawley J, Ricceri L (2009) Ultrasonic vocalizations: a tool for behavioural phenotyping of mouse models of neurodevelopmental disorders. Neurosci Biobehav Rev 33:508–515. https://doi.org/10.1016/j.neubiorev.2008.08.003
Scattoni ML, Ricceri L, Crawley JN (2011) Unusual repertoire of vocalizations in adult BTBR T+tf/J mice during three types of social encounters. Genes Brain Behav 10:44–56. https://doi.org/10.1111/j.1601-183X.2010.00623.x
Seffer D, Schwarting RKW, Wöhr M (2014) Pro-social ultrasonic communication in rats: insights from playback studies. J Neurosci Methods 234:73–81. https://doi.org/10.1016/j.jneumeth.2014.01.023
Simola N (2015) Rat ultrasonic vocalizations and behavioral neuropharmacology: from the screening of drugs to the study of disease. Curr Neuropharmacol 13:164–179
Simola N, Granon S (2018) Ultrasonic vocalizations as a tool in studying emotional states in rodent models of social behavior and brain disease. Neuropharmacology. https://doi.org/10.1016/j.neuropharm.2018.11.008
Tonoue T, Ashida Y, Makino H, Hata H (1986) Inhibition of shock-elicited ultrasonic vocalization by opioid peptides in the rat: a psychotropic effect. Psychoneuroendocrinology 11:177–184
Vivian JA, Miczek KA (1993a) Diazepam and gepirone selectively attenuate either 20–32 or 32–64 kHz ultrasonic vocalizations during aggressive encounters. Psychopharmacology 112:66–73
Vivian JA, Miczek KA (1993b) Morphine attenuates ultrasonic vocalization during agonistic encounters in adult male rats. Psychopharmacology 111:367–375
Acknowledgements
We thank Ophélie Joly and Lorie Carassini for participating in the animal experiment during internships in the laboratory. This work benefited from the animal platform facility at Neuro-PSI (Orsay, France). The manuscript was corrected by a native English proofreader (Brandon Loveall; contact@improvence.net).
Funding
This research was supported by the Service de Santé des Armées, by the CNRS, Université Paris Sud, and by a grant to FC (PEA 13co709) from the Direction Générale de l’Armement (DGA, France).
Author information
Authors and Affiliations
Corresponding author
Ethics declarations
Conflict of interest
The authors declare that there is no conflict of interest. This research received no specific grant from any funding agency in the commercial sector.
Ethical approval
All applicable international and/or institutional guidelines for the care and use of animals were followed. Experiments were conducted in accordance with the recommendations for animal experiments issued by the European Community Council guidelines (directive 2010/63/EU). The protocol was approved beforehand by an independent ethical board (reference 2015-04).
Additional information
Publisher's Note
Springer Nature remains neutral with regard to jurisdictional claims in published maps and institutional affiliations.
Rights and permissions
About this article
Cite this article
Lefebvre, E., Granon, S. & Chauveau, F. Social context increases ultrasonic vocalizations during restraint in adult mice. Anim Cogn 23, 351–359 (2020). https://doi.org/10.1007/s10071-019-01338-2
Received:
Revised:
Accepted:
Published:
Issue Date:
DOI: https://doi.org/10.1007/s10071-019-01338-2