Abstract
The boreal forest is the largest terrestrial biome in North America and holds a large portion of the world’s reactive soil carbon. Therefore, understanding soil carbon accumulation on a landscape or regional scale across the boreal forest is useful for predicting future soil carbon storage. Here, we examined the relationship between floristic composition and ecosystem parameters, such as soil carbon pools, the carbon-to-nitrogen (C/N) ratio of live black spruce needles, and normalized basal area increment (NBAI) of trees in black spruce communities, the most widespread forest type in the boreal forest of Alaska. Variability in ecosystem properties among black spruce stands was as large as that which had previously been documented among all forest types in the central interior of Alaska; we found an eightfold range in NBAI and fivefold range in mineral soil carbon and nitrogen pools. Acidic black spruce communities had significantly more carbon in the organic soil horizon than did nonacidic black spruce communities, but did not differ in any other measured ecosystem parameter. We explained 48% of the variation in total soil carbon with a combination of plant community indices and abiotic and biotic factors. Plant community composition was at least as effective as any single environmental factor or stand characteristic in predicting soil C pools in Alaskan black spruce ecosystems. We conclude that among the community properties analyzed, the presence of key groups of species, overall species composition, and diversity of certain functional types, especially Sphagnum moss species, are important predictors of soil carbon sequestration in the black spruce forest type.
Similar content being viewed by others
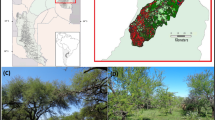
Explore related subjects
Discover the latest articles, news and stories from top researchers in related subjects.Avoid common mistakes on your manuscript.
Introduction
Plant community composition both affects and is affected by ecosystem structure and function. For example, species composition and diversity strongly influence ecosystem processes, such as nutrient cycling, productivity, decomposition rate, and trophic dynamics, particularly if the traits of one or a few species have strong ecosystem effects (for example, Hooper and Vitousek 1997; Mack and others 2004). In general, the dominant plant species account for most of these ecosystem effects because they account for most of the biotic pools and fluxes (for example, Chapin and others 1996). Thus, biogeochemical models that incorporate biotic diversity do so only at the level of biomes (for example, needle-leafed evergreen forests versus deciduous forests), or by incorporating two to four major functional types within a biome (Kittel and others 2000). Although some studies have begun to study the effect of sub-dominants on ecosystem processes (for example, Chapin 1983), there is less information on the extent to which variation in the diversity of small-statured or sub-dominant plants either reflect or influence forest ecosystem processes at the regional or landscape-scale. Here we ask whether knowledge of understory plant species composition and diversity improves the prediction of a key ecosystem property, soil carbon (C) storage, relative to predictions based only on environment and stand structure alone.
As one of the largest terrestrial biome on Earth (Whittaker 1975), the boreal forest covers an extensive part of North America, most of which has not been substantially modified by human activity. The boreal forest is important in the context of global change because of its large stock of soil C, and the speculation that it may respond to changes in climate or disturbance regime (Goulden and others 1998; Randerson and others 2006). In addition, the boreal forest is comprised of only a few tree species and has lower vascular plant diversity than the biomes to its north or south. Chapin and Danell (2001) suggested that due to its low species diversity, community and ecosystem processes are more sensitive to landscape-scale variations in plant community types than to broad latitudinal patterns of climate.
Black spruce (Picea mariana) dominated communities cover over 40% of the interior Alaska region across a broad variety of environmental conditions (Van Cleve and others 1983a). The black spruce ecosystem plays a critical role in the global C cycle because it contains more C per unit area than other major boreal forest ecosystems in North America, and high C contents at or near the soil surfaces make the black spruce ecosystem extremely susceptible to C release under a warming climate (Goulden and others 1998). Previous research in the black spruce ecosystem of boreal Alaska focused on the relationship between soil C dynamics and nutrient cycling (Chapin 1983; Van Cleve and others 1983b), fine root production (Ruess and others 2003; Vogel and others 2005), and abiotic factors (Van Cleve and others 1990; Kane and others 2005). From these and other studies in Canada (for example, Bisbee and others 2001; Dioumaeva and others 2003), we know that soil temperature and moisture, presence and depth of permafrost, age since disturbance, and litter quality control soil C storage in black spruce forests. However, there has been little or no research on the relationship between soil C patterns and regional plant community characteristics in Alaskan black spruce forests.
There are substantial differences in C pools and fluxes among Canadian black spruce communities that differ in dominant moss species and stand structural characteristics, such as stand density (Harden and others 1997; O’Connell and others 2003b), and there is also evidence that the black spruce communities of interior Alaska vary substantially in plant community composition (Viereck 1973; Hollingsworth and others 2006). A recent classification of black spruce communities revealed that floristics vary most strongly with changes in mineral soil pH and soil drainage regime (Hollingsworth and others 2006). The acidic black spruce/lichen forest is dominated by fruticose lichens and many species of mosses, whereas the nonacidic black spruce/rose/horsetail forest is characterized by greater vascular plant diversity, especially various species of Equisetum (Hollingsworth and others 2006). This floristic classification provides a framework for examining the relationship of species composition and diversity to ecosystem properties, such as soil C, across the boreal region of Alaska.
The objectives of this article are to link plant community structure to ecosystem properties to aid in our prediction of where on the landscape in interior Alaska we might expect soil C storage to be the greatest. Although plant community composition can covary with changing environmental factors, we were interested in detecting variation in soil C storage as predicted by species composition. We first describe the regional variation in tree growth/aboveground tree productivity, C/N ratio of live needles, and soil C of black spruce communities, then link community patterns of species composition and diversity and environmental variables to patterns in these ecosystem properties. Finally, we delineate sites based on community type and reexamine the patterns of soil C in that context.
Methods
Field Measurements and Sampling
We studied 32 sites along a climate/latitudinal transect from the Alaska Range (Latitude ∼63°N) to the Brooks Range (Latitude ∼67°N) covering approximately 25,000 km2 to include a wide range of variability in both community structure and function because we were interested in regional and landscape-scale patterns in ecosystem parameters (Figure 1). For ease of interpretation and discussion, these sites were grouped into study areas: The Northern study area (north of the Yukon River), the Fairbanks study area (within 100 km of Fairbanks), the Delta study area (within 50 km of Delta Junction), and the Taylor study area (along the Taylor highway near the Canadian/Alaskan border). Interior Alaska has a continental climate, with a relatively constant climate (Figure 1); however, topographical effects on local climate modify regional climate through moisture and temperature redistributions, called inversions (Hinzman and others 2006). It was not feasible to establish climate monitoring stations at all sites, therefore, we captured variations in climate through measurements of soil moisture and topography (slope position, angle, and direction).
The 32 sites are a subset of a larger study that documented the regional variability in black spruce plant community composition (Hollingsworth and others 2006), in which a floristic classification was developed, and reflect the full range of floristic composition and variability encountered in the larger study. We selected sites along a toposequence (summit, shoulder, sideslope, toeslope, and valley bottom), wherever this was feasible. The lowest elevation site was 190 m and the highest elevation site was 876 m. We used a modification of the centralized replicate sampling procedure and subjectively chose plot locations in areas of homogeneous vegetation that were dominated by a black spruce overstory (Mueller-Dombois and Ellenberg 1974). At each site, we established a 10,000 m2 plot. If this area encompassed major changes in vegetation or topography (that is, if the plot included both the summit and the shoulder of a hill), the area of the site was reduced or moved to encompass a single topographic unit. Within each plot, we conducted a relevé, defined as a vegetation sample or stand, that included a list of all plant (vascular and nonvascular) species present and a visual estimate of percent cover of each species using the Braun–Blanquét cover-abundance scale (Mueller-Dombois and Ellenberg 1974; Hollingsworth and others 2006). Latitude and longitude were measured using a Trimble Geoexplorer 3 GPS unit and physical characteristics of the site (slope, aspect, elevation, topographic position, and parent material) were recorded. We cored five to ten of the largest black spruce trees (which does not necessarily correlate with the oldest trees in the stand, but likely the most productive trees in the stand) in each site as close to the base of the tree as possible to estimate the age of the oldest trees and tree growth rates. In addition, black spruce stands in interior Alaska are typically single cohort stands that arise from stand-killing fires. We employed the point center quarter method (PCQ) (Mueller-Dombois and Ellenberg 1974) randomly at five points in each site to quantify density (trees per hectare) of trees in the stand.
Sites were revisited in September or October of the same year to measure maximum thaw depth, to collect tree needles, and obtain replicate soil cores for each site. We collected soil in two ways: First, soil samples were collected from the uppermost 15 cm of mineral soil of a soil pit and percent soil moisture, soil texture, and mineral soil pH were determined following the methods of Walker and others (1994). These samples are called “Sample A”. Second, we selected three random locations within each site, where we collected a known volume of organic soil. The underlying mineral soil was then sampled volumetrically with a Giddings soil corer to a minimum depth of 15 cm at all sampling locations. Frequently, rocks or ice prevented consistent sampling of deeper soils; however, a large proportion of the total reactive soil organic matter pool is contained in the organic and surface mineral soil. These soil cores were separated in the field into organic and mineral horizons (n = 3 cores per site). These samples are called “Sample B”.
Laboratory Analysis
Sample A mineral soils were air dried, weighed, sieved, and sent to the UAF Palmer Research Station for determination of mineral pH and soil texture. Sample B organic soils were further separated by horizon (Oi, Oe, and Oa) and Sample B mineral soils were separated by depth; the top 5 cm (approximately corresponding to the A horizon) and a 5–15 cm layer (approximately corresponding to a B horizon). Sub-samples from each horizon of each core were dried, ground, and acidified to remove carbonates. Soil C and N were then measured using a Costech elemental analyzer configured for carbon and nitrogen (Valencia, CA). We used these data together with the horizon bulk density and thickness to calculate total organic C and N density per unit area down to 15 cm mineral soil. We calculated C and N pools for total soil, organic soil, and mineral soil, because vegetation properties may affect organic soil more strongly than mineral soil C and N pools, which may also reflect surface properties of soil minerals.
We separated current year’s needles and the previous year’s needles from a composite sample of 10–20 shoots from randomly selected black spruce trees per site. After drying the needles at 25°C for 48 h, needles from each age class were composited, ground to a fine powder, and analyzed for C and N content using the same methods used for soils. To assess the analytical quality of our C and N needle data, we ran three sub-samples from 10 sites and found no significant difference in C or N values within any particular site. C/N ratios were calculated to provide a general measure of litter quality.
Annual growth rings were counted with a microscope and visually cross-dated (Swetnam and others 1985). The number of years the tree took to grow to the cored height was estimated by a regression equation that relates seedling height to age of black spruce trees on the Kenai Peninsula, Alaska (Devolder 1999). These ages were analyzed in 10-year age classes to account for errors in those estimates.
To infer aboveground tree productivity we report “basal area increment” (BAI). Typically, BAI is calculated by first calculating the average radius per year where: \( {\text{Average radius for each year}} = {\text{summing all ring widths/number of rings}} \). Then average diameter per year is calculated by: \( {\text{diameter}} = 2*({\text{Average radius}}) \). However, BAI does not take into account year-to-year dynamics (for example, competition, changes in climate, and microtopography) (A. Lloyd, personal communication). Therefore, the last 11 years of growth were used to compare across stands to infer current aboveground tree productivity. First, a radius was calculated by adding growth rate increments from the pith to the beginning of the last 11 years of growth. An area for this inner circle or “inner area” was then calculated based on this radius using the formula (\( {\text{X}} - ({\text{X}} - 1)/X - 1 \) for year X) to leave only the area for the ring in question. BAI is then expressed as a percentage of the overall basal area up to the previous year to compare growth rates for many sites and tree sizes; this BAI value is then normalized to the inner area [Normalized BAI (NBAI)] (Atkins and others 1998). NBAI for the last 11 years’ growth was then averaged for each tree and then for the site.
Statistical Analysis
Classification
Sites were classified purely based on their species composition, which strongly corresponded with the pH of the mineral soil. Therefore, instead of referring to the black spruce communities based on their floristic names, we use the convention set forth by Walker and Everett (1991) by naming floristically derived communities based on their pH tendencies (either acidic or nonacidic black spruce communities). Braun–Blanquét floristic names can be found in Hollingsworth and others (2006). Of our 32 sites 15 were part of the acidic black spruce community, 15 were part of the nonacidic black spruce community, and two were latitudinal treeline sites. The treeline sites were grouped with the nonacidic black spruce community because of the high similarity for a majority of species.
Ordination
A nonmetric multi-dimensional scaling (NMDS) ordination was run on all sites using the program PC-ORD 4.25 (McCune and Mefford 1999) to detect the main patterns in species composition, based not only on the presence, but also the abundance of each species. NMDS provides a quantitative method of separating sites based on species composition. Axis scores for each site were produced and these scores were then used as a measure of whole-site plant species composition for future analyses.
We used independent sample t-tests (equal variance not assumed) to test for differences in means of NBAI, C/N ratio, and soil C between acidic and nonacidic black spruce communities. Pearson correlation coefficients were used to correlate the C/N ratio of soil and needles, NBAI, soil N (g N m−2), and soil C (kg C m−2). We ran ordinary least square regressions to examine total soil C as a function of stand age and NBAI.
We were interested in predicting differences in soil carbon pools based on plant community characteristics (richness and composition), therefore, we ran a stepwise multiple regression to explore the correlations between plant community and ecosystem patterns, abiotic variables, and soil C pools. This employed the best subset regression method (removal P = 0.10), including nonredundant variables. We report only the best-fit model (that is, highest amount of variance explained and the most significant). It is recognized that there are many criterion methods for assessing the “best-fit” model, and we therefore report both the R 2 and the adjusted R 2. Our “best-fit” model was determined by the adjusted R 2. The adjusted R 2 is a more accurate criterion for evaluating the best-fit model because it takes the number of parameters into account through the degrees of freedom. Percent variance explained is, therefore, based on the adjusted R 2 values. We also report Beta values (regression coefficients standardized by converting to Z scores), which were used to assess the relative importance of each variable to the regression model. Beta values (b) indicate the portion of variance in a dependent variable that can be attributed directly to an independent variable, and are often termed “path coefficients” (Mitchell 1993). Descriptive statistics were run on dependent variables to test for normality, and Pearson correlation coefficients and a high variance inflation factor (VIF) (VIF > 10) were used to assess multi-collinearity among independent variables. The dependent variables for our model were total soil C, soil C in the organic soil, and soil C in the mineral soil (kg C m−2). All independent variables used in the multiple regressions are listed in Table 1. All t-tests, correlations, and regression analyses were performed in SPSS version 11.0.2.
Results
Regional Variability in Ecosystem Function
There was a wide range of values for each ecosystem parameter among the 32 black spruce study sites sampled (Figure 2, Table 2). Normalized basal area increment (NBAI, an index of aboveground tree productivity) varied eightfold across the sites (0.004–0.027, mean = 0.012 ± 0.001%), C/N ratio of first year needles varied threefold (23.5–64.3, mean = 48.5 ± 1.5), soil C pools varied fivefold (4.4–24.7, mean = 10.8 ± 0.73 kg C m−2), and soil N pools varied tenfold (172.0–1237.9, mean = 475.5 ± 34.0 g N m−2; Figure 2, Table 2). Across all sites, total soil N and C were highly correlated (r = 0.936, P = 0.0001) (Table 3), therefore, only soil C was used in the multiple regression analysis. Soil C/N, an index of organic matter quality, was moderately correlated with total soil C pools (r = 0.42, P = 0.022) (Table 3), but not significantly correlated with either the organic or mineral layer C pool. NBAI was a weak predictor of total soil C (R 2 = 0.161, P = 0.030) among these relatively mature stands (44–295 years), but there was no significant relationship between stand age and total C content (Figure 3a, b).
The regional variability in total soil carbon (kg/m2, mean ± SE), C/N of live needles, and NBAI (%, mean ± SE). Sites are grouped by study area (and within a study area: west to east). Community types are depicted by different shading. All study areas include both the nonacidic and acidic community type. (*) Mineral soil carbon was estimated using % C and bulk density from a small soil sample instead of the soil core. This was because we could not reach the mineral soil due to permafrost when we were coring. The maximum depth of mineral soil C stocks was 10 cm.
Regression line showing the relationship between total soil carbon (kg/m2) and (A) stand age (years), (B) NBAI (%) across all sites, (C) C/N ratio of needles where open circles indicate nonacidic sites and closed circles indicate acidic sites. The maximum depth of mineral soil C stocks was 19 cm. There was no significant relationship between total soil carbon and stand age (P = 0.81); however, there was a slight significant relationship (P = 0.03) between total soil carbon and NBAI. There was no significant relationship between total soil carbon and C/N of live needles across all sites (P = 0.19), however, within the acidic black spruce community (inset); C/N of live needles explains 30% of the total variance in total soil carbon (P = 0.005).
Acidic and nonacidic black spruce community types did not differ significantly in NBAI, needle C/N ratio, or total soil C pools. However, there was significantly more C stored in the organic layer of acidic than nonacidic black spruce community types (t = −2.35, P = 0.027) (Figure 4). It is important to note that total soil C was barely nonsignificant (P = 0.05) and, therefore, this difference in total soil C is most likely ecologically meaningful.
Regional Floristic Variability
An NMDS ordination was run on all sites. The Monte Carlo test of significance showed a P-value of 0.0467 for all six axes run. From these results we conclude that the best of the 1-D to 6-D solutions provide significantly more reduction in stress that expected by chance. There were 264 iterations for the final solution. The final instability value was 0.00049 and a final stress of 16.47840. From this ordination, we obtained Axis 1, 2, and 3 ordination values for each site (Table 2). These ordination axes values are a measure of the overall floristic composition of these sites and were used in further analyses.
Predictors of Regional Soil C Pools
We were interested in abiotic and biotic variables that predict soil C pools at the regional scale of interior Alaska. We looked at the soil C pools across all sites in three ways using a multiple regression approach. We considered total soil C (organic plus surface mineral), C found in the organic horizon alone, and C found in the surface mineral soil alone because the relative importance of biotic versus abiotic controls over soil C storage might differ for the organic and mineral horizons.
Total Soil C Pools for all Sites
Plant community composition was at least as important as abiotic or stand characteristics in predicting total soil C, when all sites were considered together. Sphagnum richness, Axis 1 of the NMDS ordination (floristic composition related to soil acidity) and Axis 2 (floristic composition related to soil moisture) were significant predictors of total soil C (Table 4). Soil mineral pH was the only abiotic variable, and stand age and NBAI, an estimate of recent productivity, were the only stand variables that were significant predictors of total soil C. The regression model explained 48% of the total variance in total soil C across all sites (F-value = 5.47, P = 0.001, df = 6), with the majority of this variance being explained by Axis 1 (b = −0.75) and pH (b = −0.62).
C in the Organic Soil for all Sites
Within the soil organic horizon across all sites, higher C storage was associated with lower aboveground production of black spruce trees, and in wetter sites occurring in slightly sloping or lowland areas. No plant community composition traits were good predictors of soil C in the organic soil (Table 4). The multiple regression model explained 35% of the overall variance in soil C in the organic layer across all sites (F-value = 5.10, P = 0.004, df = 4), with aspect (b = 0.48), and NBAI (b = −0.43) explaining the most variance.
C in the Mineral Soil for all Sites
Within the soil mineral layers across all sites, higher C amounts were associated with acidic, steep slopes, slower aboveground tree growth, and higher vascular and moss species richness (Table 4). The multiple regression model explained 41% of the total variance in the C of the mineral soil across all sites (F-value = 3.84, P = 0.007, df = 7), with aspect (b = −0.73) and Axis 1 (b = −0.57) explaining the most variance. Slope had an opposing effect on predicting soil C in the organic and mineral horizons, whereas NBAI showed a consistent negative correlation with organic, mineral, and total soil C.
Predictors of Soil C Pools within Communities
Because plant community composition (NMDS Axis 1) (which is highly correlated with mineral soil pH acidity) was such a strong predictor of total soil C across all sites (Table 4), we evaluated the controls over soil C in acidic (n = 15) and nonacidic (n = 17) community types separately.
The Acidic Black Spruce Community
Total soil C pools: Within the acidic black spruce community, larger total soil C pools were associated with a greater C/N ratio of the needles, suggesting that controls over decomposition (that is, C/N ratio reflecting litter quality) or overall site fertility could be important controls over soil C pool size (Table 4). Variation in the C/N ratio of live needles explained 31% of the overall variance in total soil C in the acidic black spruce community variation (F-value = 6.71, b = 0.60, df = 1) (Figure 3c).
C in the Organic Soil: Larger C amounts in the organic layer were associated with lower moss richness, a higher C/N ratio of the live needles, and lower Axis 3 values, floristic composition associated with productivity (Table 4). These variables together explained 70% of the variation in C storage in the organic layer (F-value = 12.02, P = 0.001, df = 3) with Axis 3 (b = −0.79) and moss richness (b = −0.76) contributing greatly to the variability. This suggests a strong relationship between moss richness, soil acidity, and soil carbon amounts.
C in the Mineral Soil: Larger C amounts in the mineral soil were associated with sites on steeper slopes and a higher C/N ratio of the live needles of this type (Table 4). The regression model explained 36% of the overall variation in C pools in the mineral soil (F-value = 4.62, P = 0.035, df = 2) with slope contributing more to the model (b = 0.51). Live needle C/N ratio correlated positively with soil C pools in both the organic and mineral horizons and total soil C content on an area basis in the acidic community.
The Nonacidic Black Spruce Community
Total Soil C Pools: Within the nonacidic community type, larger total soil C pools were associated with younger, relatively moist sites with high Sphagnum richness and lower rates of aboveground tree production (Table 4). The model explained 73% of the variation in soil C (F-value = 11.32, P = 0.001, df = 4) with the largest contribution from soil moisture (b = 0.55) and Sphagnum richness (b = 0.46).
C in the Organic Soil: Larger C amounts were associated with lower aboveground tree production and higher Sphagnum richness in this type. These variables explained 56% of the total variance in soil C in the organic layer (F-value = 6.72, P = 0.010, df = 2) and NBAI contributed the most to the overall variance (b = −0.49).
C in the Mineral Soil: In the mineral soils of the nonacidic community type, higher total soil C was associated with wet, young, lowland or flat sites with a shallow active layer (Table 4). The regression model explained 71% of the total variance observed (F-value = 10.74, P = 0.001, df = 4) with active layer depth (b = −0.49) contributing the most to the model.
Discussion
Regional Variability in the Black Spruce Ecosystem in Interior Alaska
Many ecosystem studies in interior Alaska have concentrated around the Fairbanks study area (for example, Van Cleve and others 1990; Ruess and others 2003; Vogel and others 2005). However, the fivefold range in surface mineral soil C pools (2.4–13.3 kg C m−2) observed across interior Alaska was larger than the range of values in mineral soil reported across a range of black spruce forest stands within the Fairbanks study area (4.1–10.4 kg C m−2). More remarkably, mineral soil C pools reported here are almost as great as the range of values reported across five different forest types within the Fairbanks study area (3.0–18.1 kg C m−2) (Van Cleve and others 1983b). This broad range of values was also observed for mineral soil N pools (110–554 g N m−2 as compared to 190–406 g N m−2) and mineral soil C/N (13–29 as compared to 11 in birch stands to 30 in black spruce stands) (Figure 2, Table 2) (Van Cleve and others 1983b). In addition, stand density varied 20-fold (639–13,212 trees/ha), which are larger ranges of values than those reported across a range of successional stands in the Fairbanks area (261–4,730 trees/ha) (Table 2) (Viereck 1983). These results clearly indicate that regional variation in forest structure and function within the black spruce ecosystem is as great as among-ecosystem variation within a landscape or study area. This breadth of variation is probably more pronounced within the black spruce ecosystem than in other conifer or hardwood types in boreal Alaska, because of the wide range in environmental conditions in which black spruce can survive and grow (Viereck and Johnston 1990).
Predicting Regional Variation in Soil C Pools
Most of the variation in ecosystem parameters reflected variation within, rather than among, geographic study areas (Figure 2). All of the four study areas included acidic and nonacidic communities, making it unlikely that differences in ecosystem processes between these community types were inadvertent consequences of regional variation in climate or other underlying factors. Topography was the primary landscape factor that drove variation in ecosystem patterns across all sites. Topography generates strong differences in soil moisture (ridgetop versus valley bottoms) and temperature (north versus south facing slopes; ridge versus valley bottom) that in turn drive regional variation in ecosystem dynamics (Van Cleve and others 1990; Kane and others 2005). Topographic variation in interior Alaska, probably accounts for much of the differences in vegetation and ecosystems when comparing interior Alaska to other areas of the boreal forest. For example, abundance of peatlands in continental Canada is often attributed to the gentle topography in continental Canada (Kuhry and others 1993), whereas in interior Alaska, peatlands are restricted to lowland areas (Chapin and others 2006).
Total soil C pools were larger (P = 0.055) in acidic than in nonacidic black spruce communities, due to differences in organic-horizon C (P = 0.027), although no difference in surface mineral soil C was detected. This observation suggests that regional and landscape variations in soil acidity affected C accumulation indirectly through ecosystem processes rather than being a direct pH effect on soil C accumulation.
The Importance of Plant Community Composition
Plant community composition was at least as effective as environmental or abiotic and stand characteristics in predicting soil C pools among 32 sites across interior Alaska (Table 4). This could occur if plant community composition was more sensitive than stand structural characteristics to important environmental variables. Axis 1 of the NMDS ordination was the most significant predictor of total soil C, and half of the variables that contributed significantly to the multiple regression predicting total soil C pool were floristic in nature: Axis 1, Axis 2, and Sphagnum richness. Community floristic variables were equally important when considering variation in the mineral horizon (Axis 1, vascular richness, and moss richness). Across all sites, young, acidic stands with high Sphagnum richness had the largest pools of total soil C (Table 4). Soil moisture alone was not a significant predictor of total soil C pools, although it was significantly negatively correlated with organic soil C pool (Table 4). Therefore, community composition may be a more effective time-integrated measure of soil moisture than direct measurements of soil moisture, which are also strongly influenced by recent weather.
It is not surprising that soil moisture, aspect, active thaw depth, and other abiotic factors appear as important predictors throughout the regression models, as these factors have been shown to influence C storage on the regional scale in boreal forests as well as other systems (Liski and Westman 1997). The unique contribution of the results presented here is that the ordination axes, which represent differences in plant species composition among sites, do a good job of representing integrated environmental conditions, and could reflect processes that directly influence soil C storage. By holding the species composition of the overstory (black spruce) constant, we increase the likelihood of detecting the relationships between understory species composition and soil C. We suggest that some of these sub-dominant functional groups (for example, Sphagnum) interact with the environment to directly drive forest processes. If correct, this has large implications for regional extrapolation and modeling, which are typically purely based on the remotely sensed distributions of overstory dominants (for example, McGuire and others 2002). Our study suggests that direct experimental work linking black spruce understory species composition (richness and abundance) to carbon accumulation is needed to fully understand and model carbon dynamics in the boreal forest of Alaska.
Overall plant species composition, as measured by the NMDS ordination scores for each site, was not as significant a predictor of soil C pools either within acidic or nonacidic community types, only Axis 3 was significant, and only in the acidic community type (Table 4). Instead, measures of species richness, such as vascular plants, moss, and Sphagnum became important. This is a logical consequence of the reduction in variation of overall species composition within, compared to among, communities. It suggests that within a given black spruce community type, the presence of particular species, and not abundance of that species, is most tightly linked to variation in soil C. When only acidic communities were considered, moss richness became the most significant predictor of total soil C and when only nonacidic sites were considered Sphagnum richness was a highly significant predictor of soil C pools. Due to the complex interactions between community patterns, abiotic factors, and ecosystem processes, the causes for these correlations are not fully understood. However, we suggest that plant diversity and composition are important integrative variables that capture the environmental heterogeneity that also affects patterns of biogeochemical cycling. This could occur if variation in biotic diversity and composition were a consequence of C accumulation that reflected the long-term physical nature of a site and/or if variation in biotic diversity and composition of the community are important determinants of C accumulation and soil environment.
In particular, it seems clear that bryophytes contribute significantly, not only to the diversity of black spruce communities of interior Alaska, but also to the functioning of these ecosystems. In fact, the wetter black spruce sites studied here have similar nonvascular species composition to other wetlands (rich and poor fens and bogs) (Camill and Clark 1998) across the circumboreal region. Mosses are excellent indicators of local conditions in peatlands, including water chemistry, height above water table, depth to permafrost, and whether a peatland is ombriotrophic or minerotrophic (for example, Kuhry and others 1993; Vitt and others 2000). For example, in central Canada, permafrost is restricted mostly to acidic ombriotrophic bogs (Vitt and others 1994) and localized permafrost bogs are one of the most bryologically diverse peatland types in boreal Canada (Beilman 2001). In addition, the presence of key species such as Sphagnum fuscum has been shown to affect local-scale peatland stability (Camill and Clark 1998). It is therefore likely that moss diversity and the presence of key Sphagnum sp. in acidic black spruce communities is likely linked to underlying ecosystem processes such as permafrost dynamics, which have a huge effect on soil C accumulation (for example, Turetsky and others 2000; Vitt and others 2000).
Importance of Abiotic Factors and Stand Structural Characteristics
The fact that community composition and diversity were strong predictors of soil C accumulation (previous section) does not mean that the physical environment is not important. The relationships that we observed between abiotic and stand characteristics and soil C pools provide insight into the potential mechanisms by which environment controls soil C accumulation in boreal black spruce forests. For example, the consistent negative correlation between aboveground tree productivity and soil C pools (organic, mineral, and total soil C) suggests that factors controlling decomposition were more important than factors controlling production as causes of regional variation in soil C pools, assuming that tree productivity correlates to overall ecosystem productivity (Mack and others 2008). In turn, thick organic mats may constrain productivity. The generally weak negative relationship between stand age and soil C accumulation (Table 4) supports Harden and others’ (1997) suggestion that black spruce sites may accumulate carbon only to the age of 80–100 years (younger than most of these stands) and in fact, older black spruce stands could be releasing carbon from the soil (Ruess and others 2003). Our results, together with these other studies, suggest that stand age may have complex and unexpected effects on regional carbon budgets and warrant further study.
The lack of relationship between stand density and soil C pools (across all sites) has practical significance because it suggests that some remotely sensible aspects of stand structure, like density, are not necessarily good predictors of soil C pools across interior Alaska. Many process-based studies have used structure as a proxy of productivity and age (as a stand gets older, it gets less productive and more open in stature) (for example, Van Cleve and others 1983b). However, these results, coupled with previous work looking more specifically at community structure (Hollingsworth and others 2006), suggest that, on the regional scale of interior Alaska, density is not a good indicator of stand-level composition and ecosystem processes. These results are similar to those of Litton and others (2004), who suggested that C allocation patterns in a post-fire lodgepole pine ecosystem are independent of tree density.
Live needle C/N ratio and active layer became significant contributors to predicting soil C pools within acidic and nonacidic black spruce communities (respectively). Live needle C/N ratio, which made no significant contribution to the model of total soil C storage across all sites, contributed most significantly in explaining all soil C pools (organic, mineral, and total) within the acidic black spruce community. The reason for the appearance of C/N ratio as a predictor of soil C in acidic sites is unclear but may relate to low N availability, leading to reduced tissue N (high leaf C/N ratio) in the most acidic sites, where C storage is most pronounced. Active layer was a significant predictor within the nonacidic black spruce community suggesting that the presence and depth of permafrost is particularly important in this community type.
Conclusions
These results clearly demonstrate the tremendous range in soil C storage within the black spruce forest type of interior Alaska. This variation appears unrelated to regional differences in climate and, therefore, is most likely biological or microclimate related. In addition, understory community composition was at least as effective a predictor of soil C pools as were the abiotic and stand structural characteristics that are typically used in models to simulate soil C dynamics across the interior Alaskan region. In particular, it appears that the presence of key species and diversity of certain functional types, such as Sphagnum, rather than abundance or overall species richness, are good predictors of black-spruce soil C storage.
The tight coupling between vegetation and the environment make it difficult to disentangle the cause and effect relationship between topography, vegetation, and soil C storage in an observational study, but there is likely truth in both interpretations. The rich literature on plant functional types and the diversity–function relationship indicates that variation in floristic composition should affect most ecosystem processes, including soil C storage. Conversely, variations in soil C storage will likely alter nutrient availability and other ecosystem properties in ways that alter the competitive balance among species and, therefore, floristic composition. We, therefore, suggest that inclusion of vegetation composition in studies of ecosystem processes such as C balance may provide insights that are overlooked when only physical and biogeochemical properties or biome types are considered.
References
Atkins S, Bosak S, Heinz A, Seldon J, Matson N, St John A. 1998. Methods of ecosystem analysis: dendrochronology (AKA Tree rings). Totoket MT, North Brandford, CT. http://www.yale.edu/fes519b/totoket/treering/treerings_results.html
Beilman DW 2001. Plant community and diversity change due to localized permafrost dynamics in bogs of western Canada. Can J Bot 79:983–93.
Bisbee KE, Gower ST, Norman JM 2001. Environmental controls on ground cover species composition and productivity in a boreal black spruce forest. Oecologia 129:261–70.
Camill P, Clark JS 1998. Climate change disequilibrium of boreal permafrost peatlands caused by local processes. Am Nat 151(3):207–22.
Chapin FS III 1983. Nitrogen and phosphorus nutrition and nutrient cycling by evergreen and deciduous understory shrubs in an Alaskan black spruce forest. Can J For Res 13:773–81.
Chapin FS III, Bret-Harte MS, Hobbie SE, Zhong H 1996. Plant functional types as predictors of transient responses of arctic vegetation to global change. J Veg Sci 7: 347–58.
Chapin FS III, Danell K 2001. Boreal forest. In: Chapin FS III, Sala OE, Huber-Sannwald E, Eds. Ecological studies 152. Biodiversity in a changing climate. New York (NY): Springer-Verlag. pp 101–20.
Chapin FS III, Hollingsworth TN, Murray DF, Viereck LA, Walker MD 2006. Floristic diversity and vegetation distribution in Alaskan boreal forest. In: Chapin FS III, Oswood M, Van Cleve K, Viereck LA, Eds. Alaska’s changing boreal forest. New York (NY): Oxford University Press. pp 81–99.
Devolder A. 1999. Fire and climate history of black spruce forests, Kenai National Wildlife Refuge, Alaska. MS thesis. Flagstaff, Arizona: Northern Arizona University
Dioumaeva I, Trumbore S, Schuur EAG, Goulden ML, Litvak M, Hirsch A 2003. Decomposition of peat from upland boreal forest: temperature dependence and sources of respired carbon. J Geophys Res 108:8222–34.
Goulden ML, Wofsy SC, Harden JW, Trumbore S, Crill PM, Gower ST, Fries T 1998. Sensitivity of boreal forest carbon balance to soil thaw. Science 279:214–7.
Harden JW, O’Neill KP, Trumbore SE, Veldhuis H, Stocks BF 1997. Accumulation and turnover of carbon in soils of the BOREAS NSA: two soil contribution to annual net C flux in a maturing spruce-moss forest (OBS NSA). J Geophys Res 102:805–16.
Hinzman LD, Viereck LA, Adams PC, Romanovsky VE, Yoshikawa K 2006. Climate and permafrost dynamics of the Alaskan boreal forest. In: Chapin FS III, Oswood M, Van Cleve K, Viereck LA, Eds. Alaska’s changing boreal forest. New York (NY): Oxford University Press. pp 39–61.
Hollingsworth TN, Walker MD, Chapin FS III, Parsons A 2006. Scale-dependent environmental controls over species composition in Alaskan black spruce communities. Can J For Res 36:1781–96.
Hooper DU, Vitousek PM 1997. The effects of plant composition and diversity on ecosystem processes. Science 277:1302–5.
Kane ES, Valentine DW, Schuur EAG, Dutta K 2005. Soil carbon stabilization along climate and stand productivity gradient in black spruce forests of interior Alaska. Can J For Res 35:2118–29.
Kittel TGF, Steffen WL, Chapin FS III 2000. Global and regional modeling of arctic–boreal vegetation distribution and its sensitivity to altered forcing. Glob Chang Biol 6(Suppl 1):1–18.
Kuhry P, Nicholson BJ, Gignac LD, Vitt DH, Bayley SE 1993. Development of Sphagnum-dominated peatlands in boreal continental Canada. Can J Bot 71:10–22.
Liski J, Westman CJ 1997. Carbon storage in forest soil in Finland 1. Effect of thermoclimate. Biogeochemistry 36:239–60.
Litton CM, Ryan MG, Knight DH 2004. Effects of tree density and stand age on carbon allocation patterns in postfire lodgepole pine. Ecol Appl 14:460–75.
Mack MC, Schuur EAG, Bret-Harte MS, Shaver GR, Chapin FS III 2004. Carbon storage in arctic tundra reduced by long-term nutrient fertilization. Nature 431:440–3.
Mack MC, Treseder KK, Manies KL, Harden JW, Schuur EAG, Vogel JG, Randerson JT, Chapin FS III. 2008. Recovery of aboveground plant biomass and productivity after fire in mesic and dry black spruce forests of Interior Alaska. Ecosystems. doi: 10.1007/s10021-007-9117-9
McCune B, Mefford MJ. 1999. PC-ORD. Multivariate analysis of ecological data, version 4.25. Gleneden Beach (OR): MjM Software Design. p 237
McGuire AD, Wirth C, Apps MJ, Beringer J, Clein J, Epstein H, Kicklighter DW, Bhatti JS, Chapin FS III, de Groot B, Efremov D, Euguster W, Fukuda M, Gower ST, Hinzman L, Huntley B, Jia GJ, Kasischke E, Melillo J, Romanovsky VE, Shvidenko A, Vaganov E, Walker DA 2002. Environmental variation, vegetation distribution, carbon dynamics and water/energy exchange at high latitudes. J Veg Sci 13:301–14.
Mitchell RJ 1993. Path analysis: pollination. In: Scheiner SM, Gurevitch J, Eds. Design and analysis of ecological experiments. New York (NY): Chapman & Hall. pp 211–31.
Mueller-Dombois D, Ellenberg H 1974. Aims and methods of vegetation ecology. New York (NY): John Wiley & Sons. p 547.
O’Connell KEB, Gower ST, Norman JM 2003b. Net ecosystem production of two contrasting boreal black spruce forest communities. Ecosystems 6:248–60.
Randerson JT, Liu H, Flanner M, Chambers SD, Jin Y, Hess PG, Pfister G, Mack MC, Treseder KK, Welp L, Chapin FS III, Harden JW, Goulden ML, Lyons E, Neff JC, Schuur EAG, Zender C 2006. The impact of boreal forest fire on climate warming. Science 314:1130–2.
Ruess RW, Hendrick RL, Burton AJ, Pregitzer KS, Sveinbjornsson B, Allen MF, Maurer GE 2003. Coupling fine root dynamics with ecosystem carbon cycling in black spruce forests of interior Alaska. Ecol Monogr 73:643–62.
Swetnam T, Thompson MA, Sutherland EK. 1985. Using dendrochronology to measure the growth of defoliated trees. Washington, DC: USDA Forest Service Agricultural Handbook no. 639
Turetsky MR, Wieder RK, Williams CJ, Vitt DH 2000. Organic matter accumulation, peat chemistry, and permafrost melting in peatlands of boreal Alberta. Ecoscience 7(3):379–92.
Van Cleve K, Dyrness CT, Viereck LA, Fox JF, Chapin FS III, Oechel WC 1983a. Taiga ecosystems in interior Alaska. Bioscience 33:39–44.
Van Cleve K, Oliver L, Schlentner R 1983b. Productivity and nutrient cycling in the taiga forest ecosystem. Can J For Res 13:747–66.
Van Cleve K, Oechel WC, Hom JL. 1990. Response of black spruce ecosystems to soil temperature modification in interior Alaska. Can J For Res 20:1530–5.
Viereck LA 1973. Wildfire in the taiga of Alaska. J Quatern Res 3:465–95.
Viereck LA 1983. Vegetation, soils, and forest productivity in selected forest types in interior Alaska. Can J For Res 13: 703–20.
Viereck LA, Johnston WF 1990. Picea mariana (Mill.) B.S.P. black spruce. In: Burns RM, Honkala BH, Eds. Silvics of North America. Washington, DC: Forest Service, US Department of Agriculture. pp 227–37.
Vitt DH, Halsey LA, Zoltai SC 1994. The bog landforms of continental western Canada in relation to climate and permafrost patterns. Arct Alp Res 26:1–13.
Vitt DH, Halsey LA, Zoltai SC 2000. The changing landscape of Canada’s western boreal forest: the current dynamics of permafrost. Can J For Res 30:283–87.
Vogel J, Valentine D, Ruess RW 2005. Soil and root respiration in mature Alaskan black spruce that vary in soil organic matter decomposition rates. Can J For Res 35:161–71.
Walker DA, Everett KR 1991. Loess ecosystems of northern Alaska: regional gradient and toposequence at Prudhoe Bay. Ecol Monogr 61:437–64.
Walker MD, Walker DA, Auerbach NA 1994. Plant communities of a tussock tundra landscape in the Brooks Range foothills, Alaska. J Veg Sci 5: 843–66.
Whittaker RJ 1975. Communities and ecosystems. New York (NY): The Macmillan Company. p 158.
Acknowledgments
We thank A. Parsons, J. Hollingsworth, J. Arseneau, L. Rose, and T. Buxbaum for field and laboratory assistance, and M. Zhurbenko, O. Afonina, C. Parker and A. Batten for help in verification of specimens. Roger Ruess, David Valentine, David Verbyla, Les Viereck, Evan Kane, Jason Vogel, Merritt Turetsky, and two anonymous reviewers provided comments on previous drafts. This work was funded through grants to the Bonanza Creek Long-Term Ecological Research (LTER) site (NSF grant numbers 9810217 and 0080609), USDA PNW Research Station (joint venture PNW01-JV11261952-231), a student research grant from the Center of Global Change and Arctic System Research at the University of Alaska Fairbanks, the UAF Austin Cooley Talent grant, and a NASA New Investigator grant to EAGS.
Author information
Authors and Affiliations
Corresponding author
Rights and permissions
About this article
Cite this article
Hollingsworth, T.N., Schuur, E.A.G., Chapin, F.S. et al. Plant Community Composition as a Predictor of Regional Soil Carbon Storage in Alaskan Boreal Black Spruce Ecosystems. Ecosystems 11, 629–642 (2008). https://doi.org/10.1007/s10021-008-9147-y
Received:
Revised:
Accepted:
Published:
Issue Date:
DOI: https://doi.org/10.1007/s10021-008-9147-y