Abstract
Energy drinks are frequently consumed by athletes prior to competition to improve performance. This study examined the effect of Red Bull™ on repeated sprint performance in women athletes. Fifteen collegiate soccer players participated, with mean age, height, and body mass equal to 19.5 ± 1.1 year, 168.4 ± 5.8 cm, and 63.4 ± 6.1 kg, respectively. After performing a familiarization trial, subjects performed three sets of eight bouts of the modified t test after ingestion of 255 mL of placebo or Red Bull 1 h pre-exercise in a randomized, placebo-controlled crossover design. Throughout testing, sprint time, heart rate (HR), and rating of perceived exertion (RPE) were continuously obtained. Repeated measures analysis of variance was used to examine differences in variables between drink conditions. Across athletes, t test time ranged from 10.4 to 12.7 s. Mean sprint time was similar (p > 0.05) between Red Bull (11.31 ± 0.61 s) and placebo (11.35 ± 0.61 s). HR and RPE increased (p < 0.05) during the bouts, but there was no effect (p > 0.05) of Red Bull on either variable versus placebo. Findings indicate that 255 mL of Red Bull containing 1.3 mg/kg of caffeine and 1 g of taurine does not alter repeated sprint performance, RPE, or HR in women athletes versus placebo. One serving of this energy drink provides no ergogenic benefit for women athletes engaging in sprint-based exercise.
Similar content being viewed by others
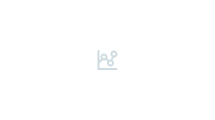
Avoid common mistakes on your manuscript.
Introduction
Caffeine is a widely used drug, as about 90% of adults in the United States consume it daily (Burke 2008). It is found in the leaves, fruits, or seeds of various plants. Caffeine is completely absorbed within the stomach and the small intestine 45 min after ingestion, and its half life in the body is 3–4 h (Astorino and Roberson 2010). Caffeine has been shown to reduce fatigue and increase wakefulness and alertness (Hewlett and Smith 2007). Due to these properties, caffeine is frequently used as an ergogenic aid for recreationally active individuals as well as athletes (Desbrow and Leveritt 2007; Burke 2008). The majority of studies indicate that caffeine improves endurance performance (Burke 2008), although there is less support for its ergogenic properties during strength- and power-based exercise dependent upon oxygen-independent metabolism (Astorino and Roberson 2010; Davis and Green 2010). Although the exact mechanism explaining caffeine’s ergogenic effects remains elusive, potential factors include adenosine antagonism (Davis et al. 2003), increased motor unit recruitment (Van Handel 1983), improved calcium handling (Doherty et al. 2004), and/or attenuated perceptions of rating of perceived exertion (RPE) (Doherty and Smith 2005) or leg pain (Motl et al. 2003).
Typically, pure anhydrous caffeine in high doses (5–6 mg/kg body weight) is provided to participants 1 h pre-exercise in a capsule or as a powder to be mixed with a beverage (Astorino and Roberson 2010). However, high doses frequently elicit side effects including anxiety and tremor, and this vehicle of caffeine intake is typically impractical to the athlete that typically does not have access to pure caffeine. In this case, use of caffeine-containing energy drinks or chewing gum containing lower doses of caffeine (1–3 mg/kg body weight) is more practical due to their availability, minimal side effects, and low cost (Paton et al. 2010). In fact, over 40% of young, elite British athletes reported energy drinks as the most widely used supplement (Petroczi et al. 2008). The most popular energy drink, Red Bull™, contains 80 mg of caffeine per serving as well as taurine and carbohydrate (Clauson et al. 2008). In a seminal study, data revealed improved reaction time, alertness, and aerobic and anaerobic endurance when one serving of Red Bull was ingested 30 min pre-trial compared to placebo (Alford et al. 2001). In young men and women, ingestion of Red Bull containing 2 mg/kg of caffeine improved bench press performance, but had no effect on peak or mean power (Forbes et al. 2007). Recently, 500 mL of Red Bull containing 160 mg of caffeine improved cycling performance in male and female cyclists (Ivy et al. 2009). However, sugar-free Red Bull containing 147 mg of caffeine did not alter run time to exhaustion in active men and women (Candow et al. 2009). Consequently, 1–2 servings of Red Bull seem to be ergogenic for aerobic activities such as cycling, although its effects seem to be equivocal for high intensity activities dependent upon oxygen-independent metabolism.
There is growing evidence that acute caffeine intake enhances repeated sprint performance in athletes. Carr et al. (2008) showed that caffeine (6 mg/kg) enhanced sprint-running performance and reduced reaction time in team sport players. Similar results were revealed in studies mimicking the intense demands of team sports (Stuart et al. 1995; Glaister et al. 2008), although Paton et al. (2001) revealed no effect of caffeine intake on repeated sprint performance. However, to our knowledge, the effects of lower caffeine doses, as found in Red Bull, on sprint performance have yet to be investigated. Another ingredient contained in Red Bull, taurine, has been shown to enhance exercise performance (Zhang et al. 2004) and augment mood, alertness, and concentration (Ivy et al. 2009), and therefore could aid short-term performance by altering perception of effort and/or reducing reaction time.
Therefore, the aim of the study was to examine the effects of one serving of Red Bull energy drink (containing 80 mg of caffeine) on sprint performance and RPE in women athletes. It is common for athletes to consume energy drinks prior to athletic competition (Hoffman 2010), yet it is unresolved whether this is an effective strategy to increase performance, especially in activities requiring speed and agility. It was hypothesized that acute Red Bull ingestion will improve repeated sprint performance versus placebo, but have no effect on RPE. A single serving was used, as it was shown to be ergogenic in a previous study (Alford et al. 2001), and likely would prevent onset of stomach discomfort during the intense, dynamic sprint-based protocol.
Methods
Subjects
Fifteen young, healthy women competing on an NAIA women’s soccer team volunteered for the study. Their age, height, and body mass were equal to 19.5 ± 1.1 year, 168.4 ± 5.8 cm, and 63.4 ± 6.1 kg, respectively. They had been playing soccer for 12–15 years, and were currently completing approximately 12 h/week of intense exercise as they were in-season. Twelve of 15 women were current caffeine consumers, although intake ranged from 1 to 7 days/week. Subjects provided written informed consent, and filled out a health questionnaire to ensure they met all inclusion criteria of the study. All experimental procedures were approved by the University Institutional Review Board.
Design
A randomized, placebo-controlled, single-blind crossover design was used in the present study. One serving of Red Bull or placebo was ingested 1 h pre-exercise. Subsequently, participants completed a dynamic warm up and then performed repeated, ‘all-out’ sprinting on a soccer field. The t test was used, as it requires running speed and agility which are important components of performance in competitive team sports such as soccer. During exercise, sprint time, heart rate (HR), and RPE were regularly assessed. On the second day of testing (at least 72 h after drink #1, and not more than 96 h) at the same time of day within subjects, they ingested the other drink, and repeated the experimental protocol. Women were instructed not to ingest any caffeine for 48 h and refrain from completing intense exercise in the 24 h before each trial, which were confirmed via written dietary and exercise recalls.
Baseline testing
Subjects’ age, height, and body mass were recorded prior to testing. On the same day, a familiarization test consisting of one set of eight sprints was completed to ensure that they were familiar with the demands of the t test. Subjects were instructed to exercise maximally during all bouts.
Drinks
The experimental and placebo drink were housed in opaque bottles, were of equal volume, and appeared, smelled, and tasted similar. The experimental solution consisted of an unlabeled bottle of Red Bull (255 mL) containing 110 kcal, and the placebo drink consisted of 190 mL of Canada Dry Gingerale (caffeine- and taurine-free) containing 91 kcal mixed with one package of lemon-flavored Crystal Light containing 5 kcal and 64 mL of cold water. One bottle of Red Bull contains 80 mg of caffeine, a dose (1.3 mg/kg) shown to be ergogenic in the form of Coca-Cola (Cox et al. 2002) as well as in energy drinks (Alford et al. 2001), as well as 1 g of taurine and 27 g of carbohydrate. Subjects were unaware of the order of treatment and were instructed to drink each solution 1 h before testing. A researcher watched each subject consume the drink.
Procedures
Subjects arrived on the soccer field 1 h after drink consumption, and were asked to describe onset of symptoms or side effects from drink intake. Initially, they completed a typical dynamic warm-up for 10 min. HR was recorded during exercise using a chest strap and a watch (Polar Electro, Lake Success, NY, USA). Subjects were required to compete 24 “all-out’ sprints, separated into three sets of eight trials of the t test (Delextrat and Cohen 2008). This protocol has previously been used to assess agility in athletes including basketball (Delextrat and Cohen 2008) and football players (Hickey et al. 2009). Each trial was timed from start to completion by two researchers, and the mean of these times was recorded out of view of participants. Mean sprint time was also recorded from each set of eight sprints. HR and RPE (Borg 1982) were recorded after every four sprints as participants crossed the finish line. After each trial, subjects were allotted 30 s of recovery before the next sprint, and 5 min of rest was allotted between each set. Pilot testing in these athletes completing repeated t tests over 2 days revealed significant reliability (ICC = 0.89), no difference in performance (t = −0.36, p > 0.05) between days (11.42 ± 0.49 s vs. 11.45 ± 0.48 s), as well as coefficient of variation equal to 4.2% and standard error of measurement = 0.08 s. These values are similar to those reported in a recent study examining the reliability of this protocol (Sassi et al. 2009).
Statistical analysis
Data were expressed as mean ± SD and analyzed using three-way ANOVA with repeated measures (SPSS Version 17.0, Chicago, IL, USA), with bouts and drink representing the within-subjects factors, and fitness as a between-subjects factor. Subjects were separated into “fast” (mean sprint time = 10.80 ± 0.22 s) and “slow” (mean sprint time = 11.80 ± 0.36 s) groups, as sprint time differed (p < 0.05) between groups. This was done as it has been reported that fitness level may mediate individual responses to acute caffeine intake (Graham 2001). The Greenhouse-Geisser correction was used to account for the sphericity assumption of unequal variances across groups. Effect size for the F ratio was expressed as partial eta-squared (η 2). When a significant F ratio was obtained, Tukey’s post hoc test was used to identify differences between means. Effect size for sprint time and RPE was equal to 0.07 and 0.06, respectively. Significance level was set as p < 0.05.
Results
t Test performance
Compared to placebo, there was no main effect (p = 0.68) of Red Bull on sprint performance; data are revealed in Fig. 1. Sprint time was significantly different (p = 0.02) across bouts (F(23,299) = 1.77, η 2 = 0.12), yet there was no drink X bout X group interaction (p = 0.71). Similarly, no effect (p = 0.64) of Red Bull intake on mean sprint time was revealed in sets 1 (11.33 ± 0.59 vs. 11.34 ± 0.53 s, 95%CI = 11.14–11.48 s), 2 (11.29 ± 0.60 vs. 11.29 ± 0.52 s, 95%CI = 11.12–11.40 s), or 3 (11.30 ± 0.53 vs. 11.43 ± 0.62 s, 95%CI = 11.18–11.49 s) versus placebo, but a significant (p = 0.03) drink X sets X group interaction was revealed, F(2,26) = 3.96, η 2 = 0.23. Sprint time was lower (p = 0.02) in the “fast” group compared to the “slow” group. Overall, 5 of 15 subjects (3 caffeine users and 2 non-users) revealed improved performance (on the order of 0.30–0.80 s reductions in sprint time) with Red Bull, 5 (4 caffeine users and 1 non-user) performed better in the placebo trial, and 5 showed no difference in sprint performance between drinks.
Rating of perceived exertion
There was a significant main effect of bouts on RPE (F(5,65) = 25.47, η 2 = 0.66, p = 0.00), but no main effect of drink (p = 0.67) or interactions were revealed (p > 0.05). RPE increased from values equal to approximately 4.0 (95% CI = 3.87–4.55) after bout 4–6.0 (95% CI = 5.75–6.62) after bout 24 of testing (Fig. 2). All means were significantly different from each other with the exception of RPE between bouts 4 and 12, 8 and 16, and 12 and 20.
Heart rate
There was a significant change in HR across bouts (F(5,65) = 13.1, η 2 = 0.50, p = 0.00), as HR increased from values equal to 158.0 ± 11.0 beats/min (95% CI = 149.0–167.1 beats/min) after bout 4 to near 170 beats/min (95% CI = 158.1–179.1 beats/min) in both treatments at bout 24, reflecting the intense nature of the sprint-based protocol (Fig. 3). Post-hoc analyses revealed that all means were significantly different from each other with the exception of HR between bouts 8 and 12, 8 and 20, and 16 and 24. There was no main effect for Red Bull versus placebo (p = 0.40), yet significant interactions (p = 0.03) were revealed for bouts X drink (F(5,65) = 2.7, η 2 = 0.17) and bouts X drink X group (F(5, 65) = 2.6, η 2 = 0.17). Across bouts, HR was consistently higher in “faster” women in the Red Bull treatment versus placebo.
Side effects
Eleven of 15 participants correctly identified the Red Bull condition compared to placebo. Side effects from Red Bull intake occurred in only two women, including onset of a stomach ache and feelings of mild tremor.
Discussion
The purpose of this study was to examine the effects of Red Bull energy drink on t test performance in women athletes. Findings indicated that intake of one serving of Red Bull did not alter repeated sprint performance, as sprint time in the placebo trial (11.35 ± 0.61 s) was similar to sprint time with Red Bull (11.31 ± 0.61 s). Furthermore, there were no significant differences in RPE or HR with Red Bull intake. These findings show that one serving of Red Bull containing 80 mg of caffeine, 27 g of carbohydrate, and 1 g of taurine has minimal effect upon repeated sprint performance in athletes.
In contrast to our findings, previous studies reveal improved performance with Red Bull for activities requiring muscular (Forbes et al. 2007) as well as cardiovascular endurance (Geiss et al. 1994; Alford et al. 2001; Ivy et al. 2009). In studies by Geiss et al. (1994) and Ivy et al. (2009), 500 mL of Red Bull increased cycling time to exhaustion in endurance athletes. In college students, sugar-free Red Bull (containing 147 mg caffeine) did not alter run time to exhaustion compared to placebo (Candow et al. 2009), similar to no effect revealed on the pro-agility test with 6 mg/kg of caffeine (Lorino et al. 2006). Similarly, the present study showed no ergogenic effect of one serving of Red Bull containing 80 mg of caffeine and 1 g of taurine. However, closer examination of the data revealed improved performance in the latter bouts of sets 2 and 3 of repeated sprinting, although it was not significant. Whether inclusion of additional exercise bouts would elicit an ergogenic effect of a single serving of this energy drink remains to be determined.
Reductions in RPE have been reported during submaximal exercise in response to acute caffeine intake (Doherty and Smith 2005). However, our data reveal no change (p > 0.05) in RPE during repeated sprinting compared to placebo. Ivy et al. (2009) reported no difference in RPE during cycling in response to 500 mL of Red Bull, although performance was improved. Perkins and Williams (1975) revealed no effect of various doses of caffeine on RPE during cycling to exhaustion in women, a similar finding to Candow et al. (2009). Recently, Astorino et al. (2011) reported no caffeine-mediated difference in RPE during maximal knee extension/flexion exercise in caffeine-habituated men. It seems that during high intensity exercise during which energy demands are more severe and fatigue occurs, caffeine is unable to reduce RPE, supporting previous conclusions (Doherty and Smith 2005).
There is clear variation in individuals’ responses to caffeine ingestion, which does not appear to be due to differences in caffeine habituation across subjects (Wiles et al. 1992; Graham 2001). However, this may be mediated by discrepancies in caffeine metabolism between individuals caused by a single substitution in the gene coding for caffeine degradation (Sasche et al. 1999). In the present study, five women (two in the “fast” group and three in the “slow” group) revealed improved (>4.2%) sprint performance with Red Bull; however, an additional five (two in the “fast” group and three in the “slow” group) performed better in response to placebo. Consequently, scientists should consider examining individual data in response to caffeine ingestion, and should be aware that aggregate data revealing no effect of caffeine intake do not necessarily mean that no one benefited from caffeine ingestion. Often, individual data are more important than group means in studies investigating efficacy of various ergogenic aids, and concluding no treatment effects across groups without considering individual responses may be flawed.
One limitation of the current study included the relatively low caffeine dose equal to 80 mg contained in one serving of Red Bull. Despite Alford et al. (2001) revealing an ergogenic effect of this dose, recent studies revealed that higher volumes of Red Bull containing up to 160 mg of caffeine enhanced endurance performance (Ivy et al. 2009) as well as bench press performance (Forbes et al. 2007). This absolute caffeine dose approximated 1.1–1.5 mg/kg across athletes of varied size and sprint ability. To our knowledge, no one has examined potential differences in responses to ergogenic aid intake in athletes varying in body mass, gender, body composition, or fitness, which has a lot of practical significance to the athlete or coach. In addition, it may be that higher caffeine doses (5–6 mg/kg) are necessary to improve sprint performance in athletes (Stuart et al. 1995; Carr et al. 2008; Glaister et al. 2008). It is also plausible that the number of sprints completed was insufficient to elicit an ergogenic effect of Red Bull. A greater number of sprints were used in previous studies in which performance was improved with caffeine ingestion (5–6 mg/kg) (Carr et al. 2008; Glaister et al. 2008). However, pilot testing revealed that subjects could not complete more than three sets of this protocol without onset of extreme fatigue and/or nausea, which would have most likely prevented subsequent efforts. Lastly, women participating in the study were trained athletes completing repeated tests requiring agility and speed, so results cannot be applied to men, less active populations, or to performance of straight-line sprints.
In conclusion, findings suggest little benefit of one serving of Red Bull containing caffeine, taurine, and carbohydrate on repeated sprint performance in women athletes. Additional studies are merited to examine the effect of larger doses of Red Bull on performance in various sports involving repeated bouts of high-intensity exercise. Increasing the number of bouts could contribute to a higher rate of fatigue, which may help to reveal if one serving of Red Bull improves sprint performance.
References
Alford C, Cox H, Wescott R (2001) The effects of red bull energy drink on human performance and mood. Amino Acids 21(2):139–150
Astorino TA, Roberson DW (2010) Efficacy of acute caffeine ingestion for short-term, high-intensity exercise performance: a systematic review. J Strength Cond Res 24(1):257–265
Astorino TA, Terzi MN, Roberson DW, Burnett TR (2011) Effect of caffeine intake on pain perception during high-intensity exercise. Int J Sports Nutr Exerc Metab (in press)
Borg G (1982) Category scale with ratio properties for intermodal and interindividual comparisons. In: Geisler HG, Petzold P (ed) Psychophysical judgment and the process of perception. North-Holland, Berlin, pp 25–33
Burke LM (2008) Caffeine and sports performance. Appl Physiol Nutr Metab 33:1319–1334
Candow DC, Kleisinger AK, Grenier S, Dorsch KD (2009) Effect of sugar-free Red Bull energy drink on high-intensity run time-to-exhaustion in young adults. J Str Cond Res 23(4):1271–1275
Carr A, Dawson B, Schneiker K, Goodman C, Lay B (2008) Effect of caffeine supplementation on repeated sprint running performance. J Sports Med Phys Fitness 48(4):472–478
Clauson KA, Shields KM, McQueen CE, Persad N (2008) Safety issues associated with commercially available energy drinks. J Am Pharm Assoc 48:55–67
Cox GR, Desbrow B, Montgomery PG, Anderson ME, Bruce CR, Macrides TA, Martin DT, Moquin A, Roberts A, Hawley JA, Burke LM (2002) Effect of different protocols of caffeine intake on metabolism and endurance performance. J Appl Physiol 93:990–999
Davis JK, Green JM (2010) Caffeine and anaerobic performance: ergogenic value and mechanisms of action. Sports Med 39(10):813–832
Davis JM, Zhao Z, Stock HS, Mehl KA, Buggy J, Hand GA (2003) Central nervous system effects of caffeine and adenosine on fatigue. Am J Physiol 284:R399–R404
Delextrat A, Cohen D (2008) Physiological testing of basketball players: toward a standard evaluation of anaerobic fitness. J Str Cond Res 22(4):1066–1072
Desbrow B, Leveritt M (2007) Well-trained endurance athletes’ knowledge, insight, and experience of caffeine use. Int J Sports Nutr Exerc Metab 17:328–339
Doherty M, Smith PM (2005) Effects of caffeine ingestion on rating of perceived exertion during and after exercise: a meta-analysis. Scand J Med Sci Sports 15:69–78
Doherty M, Smith PM, Hughes M, Davison R (2004) Caffeine lowers perceptual response and increases power output during high-intensity cycling. J Sports Sci 22:637–643
Forbes SC, Candow DG, Little JP, Magnus C, Chilibeck PD (2007) Effect of Red Bull energy drink on repeated Wingate cycle performance and bench-press muscle endurance. Int J Sports Nutr Exerc Metab 17(5):433–444
Geiss KR, Jester I, Falke W, Hamm M, Waag KL (1994) The effect of a taurine-containing drink on performance in ten endurance athletes. Amino Acids 7:45–56
Glaister M, Howatson G, Abraham CS, Lockey RA, Goodwin JE, Foley P, McInnes G (2008) Caffeine supplementation and multiple sprint running performance. Med Sci Sports Exerc 40(10):1835–1840
Graham TE (2001) Caffeine and exercise: metabolism, endurance, and performance. Sports Med 31(11):785–807
Hewlett P, Smith A (2007) Effects of repeated doses of caffeine on performance and alertness: new data and secondary analyses. Hum Psychopharmacol 22(6):339–350
Hickey KC, Quatman CE, Myer GD, Ford KR, Brosky JA, Hewett TE (2009) Methodological report: dynamic field tests used in an NFL combine setting to identify lower-extremity functional asymmetries. J Str Cond Res 23(9):2500–2506
Hoffman JR (2010) Caffeine and energy drinks. Str Cond J 32(1):15–20
Ivy JL, Kammer L, Ding Z, Wang B, Bernard JR, Liao YH, Hwang J (2009) Improved cycling time-trial performance after ingestion of a caffeine energy drink. Int J Sports Nutr Exerc Metab 19:61–78
Lorino AJ, Lloyd LK, Crixell SH, Walker JL (2006) The effects of caffeine on athletic agility. J Str Cond Res 20(4):851–854
Motl RW, O’Connor PJ, Dishman RK (2003) Effect of caffeine on perceptions of leg muscle pain during moderate intensity cycling exercise. J Pain 4(6):316–321
Paton CD, Hopkins WG, Vollebregt L (2001) Little effect of caffeine ingestion on repeated sprints in team-sports athletes. Med Sci Sports Exerc 33(5):822–825
Paton CD, Lowe T, Irvine A (2010) Caffeinated chewing gum increases repeated sprint performance and augments increases in testosterone in competitive cyclists. Eur J Appl Physiol 110(6):1243–1250
Perkins R, Williams MH (1975) Effect of caffeine upon maximal muscular endurance of females. Med Sci Sports 7(3):221–224
Petroczi A, Naughton DP, Pearce G, Bailey R, Bloodworth A, McNamee MJ (2008) Nutritional supplement use by elite young UK athletes: fallacies of advice regarding efficacy. J Int Soc Sports Nutr 5:22
Sasche C, Brockmoller J, Bauer S, Roots I (1999) Functional significance of a C to A polymorphism in intron 1 of the cytochrome P450 1A2 (CYP1A2) gene tested with caffeine. Br J Clin Pharmacol 47:445–449
Sassi RH, Dardouri W, Yahmed MH, Gmada N, Mahfoudhi ME, Gharbi Z (2009) Relative and absolute reliability of a modified agility T-test and its relationship with vertical jump and straight sprint. J Str Cond Res 23(6):1644–1651
Stuart GR, Hopkins WG, Cook C, Cairns SP (1995) Multiple effects of caffeine on simulated high-intensity team-sport performance. Med Sci Sports Exerc 37(11):1998–2005
Van Handel P (1983) Caffeine. In: Williams MH (ed) Ergogenic aids in sport. Human Kinetics, Champaign, pp 128–163
Wiles JD, Bird SR, Hopkins J, Riley M (1992) Effect of caffeinated coffee on running speed, respiratory factors, blood lactate, and perceived exertion during 1500 m treadmill running. Br J Sports Med 26:116–120
Zhang M, Izumi I, Kagamimori S, Sokejima S, Yamagami T, Liu Z, Qi B (2004) Role of taurine supplementation to prevent exercise-induced oxidative stress in healthy young men. Amino Acids 26:203–207
Acknowledgments
The authors thank the athletes for providing outstanding effort during the course of testing.
Conflict of interest
The authors declare that they have no conflict of interest in completion of this study.
Author information
Authors and Affiliations
Corresponding author
Rights and permissions
About this article
Cite this article
Astorino, T.A., Matera, A.J., Basinger, J. et al. Effects of red bull energy drink on repeated sprint performance in women athletes. Amino Acids 42, 1803–1808 (2012). https://doi.org/10.1007/s00726-011-0900-8
Received:
Accepted:
Published:
Issue Date:
DOI: https://doi.org/10.1007/s00726-011-0900-8