Abstract
Methylphenidate (MPD) is one of the most prescribed pharmacological agents, which is also used for cognitive enhancement and recreational purposes. The objective of this study was to investigate the repetitive dose–response effects of MPD on circadian rhythm of locomotor activity pattern of female WKY rats. The hypothesis is that a change in the circadian activity pattern indicates a long-lasting effect of the drug. Four animal groups (saline control, 0.6, 2.5, and 10.0 mg/kg MPD dose groups) were housed in a sound-controlled room at 12:12 light/dark cycle. All received saline injections on experimental day 1 (ED 1). On EDs 2–7, the control group received saline injection; the other groups received 0.6, 2.5, or 10.0 mg/kg MPD, respectively. On ED 8–10, injections were withheld. On ED 11, each group received the same dose as EDs 2–7. Hourly histograms and cosine statistical analyses calculating the acrophase (ϕ), amplitude (A), and MESOR (M) were applied to assess the 24-h circadian activity pattern. The 0.6 and 2.5 mg/kg MPD groups exhibited significant (p < 0.05) change in their circadian activity pattern on ED 11. The 10.0 mg/kg MPD group exhibited tolerance on ED 11 and also a significant change in activity pattern on ED 8 compared to ED 1, consistent with withdrawal behavior (p < 0.007). In conclusion, chronic MPD administration alters circadian locomotor activity of adult female WKY rats and confirms that chronic MPD use elicits long-lasting effects.
Similar content being viewed by others
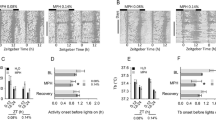
Avoid common mistakes on your manuscript.
Introduction
The circadian rhythm regulates many aspects of mammalian physiology and behavior and is an integral part of life for all bodily functions. It is seen in a range of life forms from the subcellular organelles to the complex human body (Welsh et al. 1995, Moore 1983). The suprachiasmatic nucleus (SCN) is widely accepted as the master clock (Moore 1983; Reid and Burgess 2005; Sujino et al. 2003; Yamazaki et al. 2000) and expresses several clock genes such as Per1, Per2, Cry1, Cry2, bmal1, and Rev-erb (Klein et al. 1991; Reppert and Weaver 2002). This master clock also dictates the circadian rhythm and synchronizes multiple internal clocks governing physiological processes such as hormonal release, heart rate, blood pressure, eating pattern, and sleep/wake cycle (Bittman et al. 2003; Janssen et al. 1994; Mistleberger 2005) by entraining to the light/dark cycle via the retinohypothalamic tract (Benstaali et al. 2001; Cermakian and Sassone-Corsi 2002; Johnson et al. 1988; Moore and Lenn 1972). In addition to environmental cues, pharmacological agents such as psychostimulants have been shown to have effects on diurnal activity differences. For example, chronic EtOH alters Per1 and Per2 gene expression in hypothalamus and Per2 and Per3 in SCN (Chen et al. 2004; Manev and Uz 2006) causing sleep–wake abnormalities and depression (Vitaterna et al. 2001). Methamphetamine alters striatal Per1 and Per2 genes causing a shift from nocturnal to diurnal rhythms (Iijima et al. 2002). Methylphenidate has been shown to delay the SCN electrical activity, resulting in altered sleep and circadian rhythmicity (Antle et al. 2012). Disruption of circadian rhythm can result in short-term conditions, such as jet lag, fatigue, and insomnia, and contribute to long-term pathologies such as diabetes mellitus, heart disease, and hypertension (Bray and Young 2007; Filipski et al. 2002; Karlsson et al. 2001; Lamont et al. 2007). In addition, changes in circadian rhythmic activity serve as experimental markers in animals to correlate with long-term effects of psychostimulant in humans (Algahim et al. 2009; Bergheim et al. 2012; Glaser et al. 2012; Lee et al. 2011).
Methylphenidate hydrochloride is a central nervous system stimulant resembling in structure to dextroamphetamine (Kallman and Isacc 1975, Patrick and Markowitz 1997). The d-enantiomer is pharmacologically active, but the l-enantiomer does cross the blood–brain barrier contrary to prior belief (Ding et al. 2004). When MPD is given intravenously, it binds to DA transporter (DAT) and prevents the reuptake of DA to the presynaptic terminal, resulting in the accumulation of DA in the synaptic cleft (Izenwasser et al. 1999; Massello and Carpenter 1999; Patrick and Markowitz 1997; Volkow et al. 1999). Cross sensitization between MPD and cocaine was reviewed by Gatley et al. (1999), citing 26 animal studies supporting the similarity between the two stimulants in terms of drug discrimination, self-administration, locomotor activity, and other specific behaviors, while six opposing animal studies were also reviewed. MPD, or better known through its trade name Ritalin, is currently the most prescribed treatments for attention deficit and hyperactive disorder (ADHD) according to the CDC report (2011). Moreover, MPD is gaining popularity among college students as well as middle-age adults without the diagnosis of ADHD as a cognitive enhancer and for recreational usage (Greely et al. 2008; Stix 2009). A 2005 survey of US college-age students shows that 7 % have used stimulant for “non-medical” reason at least once (McCabe et al. 2005).
This experiment aims to study the long term effect of acute and chronic MPD dose response exposure on adult female Wistar–Kyoto (WKY) rats. Female rats have mainly been excluded from studies due to their more complex reproductive system than males. It is reported that responses to morphine, amphetamine, and cocaine are stronger in females than males in animal models and humans (Brecht et al. 2004; Hernandez-Avila et al. 2004; Lynch 2008). It is reported that gender and strain affect the responses to psychostimulants (Chelaru et al. 2012; Dafny and Yang 2006; Kelly et al. 1999; Melnick and Dow-Edwards 2001). To our knowledge, no prior studies have been done on adult female WKY rats. This strain was chosen as the study subject in contrast to other strains, such as Sprague–Dawley (SD) or spontaneously hypertensive rats (SHR), for genetic variation. The immediate effects of MPD on WKY, SHR, and SD rats were reported (Amini et al. 2004; Yang et al. 2003); however, none was reported on the long-term effect of MPD on WKY rats. This study will close the gap. The hypothesis is that alteration in the circadian activity pattern after chronic drug exposure indicates that the drug does exert long-term effect. The objective of this study is to confirm the above hypothesis by recording the effect of acute and chronic MPD on circadian activity pattern of adult WKY female rats for 11 consecutive days.
Methods
Animals
Female adult WKY rats (N = 32) weighing from 200 to 250 g were housed as four rats per cage. The cages were in a sound-controlled room with ambient temperature of 21 ± 2 °C and a relative humidity of 37–42 %. In addition, food pellets and water were supplied ad libitum throughout the experiment. The experimental room was maintained on a 12:12 light/dark schedule (light on at 0600). Animals were housed in the same test cages for 7 days for acclimatization to internally synchronize their neuroendocrine systems. On the last day of the naturalization, rats were weighed and randomly divided into one control group and three treatment groups. The groups are as follow: saline (control, n = 8), 0.6 mg/kg MPD (n = 8), 2.5 mg/kg MPD (n = 8), and 10.0 mg/kg MPD (n = 8). The animals were individually housed in the test cages (these test cages became their home cages), and an additional 24 h of accommodation was allowed before locomotor activity recording was begun.
Experimental cages
The experimental protocol has been described in detail (Gaytan et al. 1997, Yang et al. 2003, 2011). In short, open field boxes (40.5 × 40.5 × 31.5 cm each) were used, which were equipped with two levels of 16 infrared beams with their motion sensors. These were placed 6 and 12 cm from the cage floor, respectively. A computerized animal activity monitoring (CAAM; AccuScan Instruments, Inc., Columbus Ohio) system checked each sensor beam at a frequency of 100 Hz to determine whether beams were interrupted. The interruption of any beam was recorded as an activity score; cumulative counts were compiled and downloaded every 10 min into OASIS data collection program that differentiated these events into various locomotor activity indices.
Drugs
Methylphenidate (MPD) hydrochloride was dissolved in 0.9 % saline and dosages calculated as freebase. The animals were injected intraperitoneally (i.p.) with an equal volume of 0.8 cc with 0.9 % saline. There are no universally recognized MPD dosage guidelines or blood levels to achieve optimum treatment. A study of 289 patients treated with MPD (White and Yadao 2000) reported that the range of doses was from 0.06 to 29.3 mg/kg with the majority being 1.0–3.0 mg/kg (Crutchley and Temlett 1999, Kollins et al. 2001, Solanto 1998 and Solanto 2000). Drug effects in rodents often require higher doses than in humans because they metabolize MPD more rapidly than humans (Gatley et al. 1999). Doses between 0.5 and 3.5 mg/kg MPD given intraperitoneally in rodents were reported to promote plasma peak concentration within the typical clinical range (Kuczenski and Segal 2002). The range of 5–10 mg/kg MPD is considered moderate dosage and above 10 mg/kg as a high dosage (Bowman and Kuhn 1996; Brandon and Steiner 2003; Kollins et al. 2001; Solanto 1998; Solanto 2000). The three treatment dosages 0.6, 2.5, and 10.0 mg/kg of MPD-D chosen in this study were based on our previous dose–response studies. Chronic treatment with 2.5 mg/kg and, in a few cases, also the 0.6 mg/kg MPD dose elicited behavioral sensitization (Gaytan et al. 1996, 1997, 2000a, 2000; Lee et al. 2008; Wanchoo et al. 2009; Yang et al. 2000a, 2001, 2000b, 2003, 2006a, 2006b, 2007). The doses in the present study correlate to low, medium, and high doses, respectively (Dafny and Yang 2006). Injections were made between 0630 and 0700 hours each day according to protocol (Table 1).
Procedure
Locomotor activity was recorded non-stop for 11 days using the CAAM system except during animal handling. The horizontal activity (HA), which measures the overall motor activity in the lowest sensor, was evaluated in this present study. The experiment was carried out in accordance with the guidelines of the NIH and the Declaration of Helsinki, and approved by our local animal welfare committee.
Data analysis
Data analysis was performed to assess dose–response effects of three different doses of MPD on the hourly total HA counts for EDs 1, 2, 7, 8, and 11 (Table 2). In addition to the three dosage groups, a control group was also used to ensure uniformity throughout the experiment (Table 1). The effect of MPD was divided into four phases of drug activity (Podet et al. 2010; Yang et al. 2006a, b, 2011). The four phases are: (1) Acute phase—the effect after MPD injection on ED 2 to ED 1, the baseline post-saline injection, was compared to observe whether a single (acute) injection was able to change the locomotor diurnal rhythm activity pattern. (2) Induction phase—the activity pattern on ED 7, the last day of the drug maintenance treatment, was compared to that of ED 2 to observe whether 6 days of MPD treatment induced any changes in activity pattern. (3) Washout phase (also known as withdrawal phase)—the activity patterns of ED 8, 9, and 10 were compared to that of ED 1 to observe whether the 6 days of MPD treatment (ED 2–7) altered the baseline activity levels of days without treatment (ED 8–10). (4) Expression phase—the activity pattern of ED 11 was compared to that of ED 1 to observe whether the effect of chronic MPD exposure during ED 2–7 was expressed after 3 days without treatment (ED 8–10).
Two forms of data analysis were performed to evaluate the dose–response effects of the three MPD doses. The 10-min activity counts were summed to hourly histograms and their mean and standard deviation was calculated. The hourly histograms with their mean and standard deviation were used to evaluate visually any changes in circadian rhythm pattern compared to the control histogram. The second evaluation used the 10-min counts to calculate the 24-h rhythms for each of the four phases using the cosine curve statistical analysis (CCSA) test (Bingham et al. 1982). This test analyzed the data using three parameters: MESOR (average activity represented by the curve and its peak activity), amplitude (distance from the MESOR to the highest point of the approximating curve), and acrophase (time at which the maximum/amplitude occurs). The estimate of these three parameters provides the ability to test for statistically significant changes in HA pattern with regard to time and intensity within the 24-h experimental period. It is this parameterization that allows for accurate representation to find out whether a significant shift in HA circadian rhythm activity pattern occurred in the acute, induction, washout, or expression phases.
Results
Control (saline injection)
The locomotor activity of eight adult female WKY rats injected i.p., with 0.8 cc 0.9 % saline served as the control group. Each animal was injected every day between 0630 and 0700 hours for seven consecutive days and then a period of 3 days with no injection, followed by another saline rechallenge injection on ED 11 (Table 1). Recording of motor activity began after the first injection at about 0700 hours, nonstop until 24 h after the last injection of ED 11. Figure 1a shows a summary of hourly HA counts for ED 1, 2, 7, 8, and 11 of the saline control group, which shows the (control) rhythmic activity of a nocturnal animal: an increase in locomotor activity during the dark phase (night) and decreased motor activity during the light period (day). During ED 1, 2, 7, 8, and 11, the HA followed this general pattern with minor changes that were deemed insignificant (Fig. 1a). Figure 1b provides the superimposed 10-min counts of HA and the calculated CCSA test of all four groups on ED 1 post-injection, demonstrating that all the locomotor activity exhibits the same general locomotor activity pattern with only minor insignificant fluctuations, i.e., all animal groups have similar baseline activity pattern. Based on these findings, one can conclude that significant deviations from the locomotor activity obtained on ED 1 could be attributed to the drug (MPD) effect (Algahim et al. 2009; Lee et al. 2011; Bergheim et al. 2012). Due to the observation that HA obtained at ED 1 was the same as all other EDs in the control group and that all four groups had similar activity pattern post-injection on ED1, it follows that the activity of ED 1 after saline injection in each MPD group can and was used as the control for the drug effect of that dose group.
Baseline activity pattern. a Hourly histogram of the control group demonstrating consistent rhythm of the average horizontal activity counts per hour of EDs 1, 2, 7, 8, and 11. The arrows indicate time of injection (saline) and shaded areas indicate the dark period of the 23-h experimental period. Only experimental day 1 begins at 0600 hours, whereas all other days thereafter begin at 0000 hours. The rhythmic activity of a nocturnal animal, i.e., increased locomotor activity during the dark phase (night) and decreased motor activity during the light period (day), is observed. b A 24-h cosine curve statistical analysis of horizontal activity circadian rhythm pattern in counts per 10 min, post-injection, for all treatment groups on ED 1. The locomotor activities of the four animal groups exhibit the same general locomotor activity pattern with only minor insignificant fluctuations. Time 0 = 0700 hours; the shaded area indicates a dark period of the 24-h experimental period. CCSA cosine curve statistical analysis
MPD acute effects (comparing ED 2 to ED 1)
The 0.6 mg/kg MPD dose showed an initial increase in activity on ED 2 following MPD injection compared to ED 1 (Fig. 2a—0.6 mg/kg dose) and return to baseline level for the remaining period. The CCSA showed no significant alteration in the HA pattern activity on ED 2 compared to ED 1 (Fig. 2b—0.6 mg/kg; Table 2). The 2.5 mg/kg MPD injection caused an increase in activity during the initial post-injection hours and between 1800 and 2000, i.e., from the 11th to 13th hour post-injection (Fig. 2a—2.5 mg/kg). However, the CCSA test (Fig. 2b—2.5 mg/kg) found this increase in activity at ED 2 compared to ED 1 to be of no statistical significance. The acute 10 mg/kg MPD dose exposure resulted in an increase in HA within the first 3 h post-injection (Fig. 2a, b—10 mg/kg). This immediate high-activity response to MPD injection exerted an exaggerated effect on the 24-h fitted CCSA test, i.e., “pulling” the curve “up” at the far left at approximately hour zero (Fig. 2b—10 mg/kg). To appropriately parameterize the long-term effects of MPD on the locomotor activity patterns, these first 3 h of data were excluded from the CCSA test, resulting in a cosine curve more accurately representing the long-term effects of the entire time post-injection pattern of ED 2. These data were used for the analysis of this paper. The 10 mg/kg MPD dose also exhibited an increase in activity between 6th and 8th, 9th and 12th, and 13th and 16th hours post-injection (Fig. 2a—10 mg/kg). The CCSA showed statistically significant decrease in the acrophase (p = 0.013) (Fig. 2c—10 mg/kg; Table 2).
Acute phase response in the three experimental dose groups. a Histogram representation of HA counts/h of experimental days 1 and 2. Arrow indicates time of injection (saline on ED1 and MPD on ED2). b Cosine curve comparing experimental days 1 and 2 over a 23-h period post-drug injection. Time 0 = 0700 hours; the shaded area indicates a dark period of the 24-h experimental period. The CCSA test shows no significant alteration in the HA pattern activity on ED 2 compared to ED 1 for the 0.6 and 2.5 mg/kg dose groups; the 10.0 mg/kg dose group exhibited a statistical significant decrease in acrophase
MPD induction phase (comparing ED 7 to ED 2)
The 0.6 mg/kg MPD injection at ED 7 elicited the same increase in activity post-MPD injection as at ED 2 (Fig. 3a—0.6 mg/kg). However, this increase in activity was prolonged and lasted from 0 to 5th hour post-injection. The activity also increased during the 7th–10th and 11th–16th hours after injection on ED 2 (Fig. 3a—0.6 mg/kg dose). The statistical comparison using the CCSA test (Fig. 3b—0.6 mg/kg; Table 2) showed that these changes in locomotion were not statistically different (Table 2). The locomotor activity following 2.5 mg/kg MPD at ED 7 exhibited an increase in activity that lasted from 0 to 11th hour after injection. In addition, an increase in activity was seen again from 13th to 16th hour after injection (Fig. 3a—2.5 mg/kg dose). However, the CCSA test showed that these changes in locomotive activity pattern were statistically insignificant (Fig. 3b—2.5 mg/kg; Table 2), i.e., 6 days of 2.5 mg/kg MPD had no effects on the HA circadian activity patterns. The 10 mg/kg of MPD exposure on ED 7 compared to ED 2 locomotor activity only exhibited an increase in activity between the 1st and 5th hour after injection (Fig. 3a—10 mg/kg). The CCSA test for this group shows the change to be statistically insignificant (Fig. 3b—10 mg/kg; Table 2).
Induction phase effect in the three experimental dose groups. a A histogram representing HA counts/h of experimental days 2 and 7. Arrows indicate time of injection (MPD). b Cosine curve comparing experimental days 2 and 7 over a 23-h period post-drug injection. Time 0 = 0700 hours; the shaded area indicates a dark period of the 24-h experimental period. The CCSA test shows no significant alteration in the HA pattern activity for all three dose groups
Washout phase (comparing ED 8 to ED 1)
On ED 8, MPD injections were held to determine whether withdrawal was induced by the six daily administrations of MPD. The 0.6 mg/kg MPD dose group exhibited increased activity from 0 to 2nd hour post-injection, at the 5th hour, and 10–12th hour post-injection (Fig. 4a—0.6 mg/kg). The CCSA test of ED 8 versus ED 1 locomotor activity was found to be statistically insignificant (Fig. 4b—0.6 mg/kg; Table 2). The 2.5 mg/kg MPD dose group exhibited an increase in activity from 0 to 2nd, 5th to 7th, 9th to 12th, and 13th to 17th hours post-injection (Fig. 4a—2.5 mg/kg dose curve). However, the CCSA test shows these increases to be statistically insignificant (Fig. 4b—2.5 mg/kg; Table 2). The 10 mg/kg MPD dose group also exhibited an increase in locomotor activity from 0 to 4th and 9th to 17th hours post-injection (Fig. 4a—10 mg/kg). The CCSA test showed statistical significance with regard to the acrophase (p = 0.007) (Fig. 4b—10 mg/kg; Table 2). At the beginning of ED 8, all of the experimental animals showed an increase in activity at the time that they had received the MPD injections during the previous 6 days. This suggests that the animals were expecting to get the next dose of the MPD drug or that they were expressing behavioral withdrawal.
Washout phase effect in the three experimental dose groups. a Histogram representation of HA counts/h of experimental days 1 and 8. Arrow indicates time of injection (saline on experimental day 1). At the beginning of ED 8, all of the experimental animals showed an increase in activity at the time that they had received the MPD injections during the previous 6 days. b Cosine curve comparing experimental days 1 and 8 over a 23-h period. Time 0 = 0700 hours; the shaded area indicates a dark period of the 24-h experimental period. The CCSA test showed statistical significance with regard to the acrophase of the 10.0 mg/kg MPD dose group
MPD expression phase (comparing ED 11 to ED 2)
To determine whether the changes induced by repetitive MPD administration were transient or long-lasting, the locomotor activity of ED 11 was compared to that of ED 2. The 0.6 mg/kg MPD groups exhibited an increase in activity of during the 3rd and 12th–17th hours post-MPD injection (Fig. 5a—0.6 mg/kg). The CCSA test shows a significant increase in amplitude (p = 0.041) (Fig. 5b—0.6 mg/kg; Table 2). The 2.5 mg/kg MPD groups exhibited an increase in activity from 0 to 5th hour post-injection, followed by an increase in activity between 8th and 12th hour post-injection, as well as 13th to 17th hour post-injection (Fig. 5a—2.5 mg/kg dose). The CCSA test of the ED 11 activity compared to that of ED 2 activity post-MPD exposure exhibits significant difference in the MESOR (p = 0.027) (Fig. 5b; Table 2—2.5 mg/kg). The 10 mg/kg MPD group at ED 11, i.e., after six daily MPD exposures and three washout days, showed an initial increase in locomotor activity when compared with ED 2 between the 0 and 4th hour post-injection followed by an increase in activity from 11th to 13th hour post-injection (Fig. 5a—10.0 mg/kg dose histogram). The CCSA test shows statistically insignificant difference between ED 11 and ED 2 (Fig. 5b—10 mg/kg cosine curve; Table 2).
Expression phase effect in the three experimental dose groups. a Histogram representation of HA counts/h of experimental days 2 and 11. Arrows indicate the time of injection (MPD). b Cosine curve comparing experimental days 2 and 11 over a 23-h experimental period. Time 0 = 0700 hours; the shaded area indicates a dark period of the 24-h experimental period. The CCSA test shows statistical significance in amplitude of the 0.6 mg/kg MPD group and MESOR of the 2.5 mg/kg MPD group
Discussion
The neurotransmitter dopamine facilitates multiple critical facets of human physiology such as locomotion, cognition, emotion, and reward. Thus, it is prudent that a pharmacological agent that increases the extracellular concentrations of dopamine in synaptic clefts be further investigated, especially when the prescribed and non-prescribed usage of such agent is on the rise. It would also stand to reason that animal models of a known subpopulation that use this substance be specifically studied, since using human subjects for this purpose in a prospective manner would be impractical and unethical.
Three major findings of this study
(1) The 0.6 mg/kg dose group shows an increased amplitude at ED 11, the expression phase, that was statistically significant (p = 0.041) (Table 2). This change in locomotor activity after a washout period implied that chronic 0.6 mg/kg MPD exposure altered the circadian rhythm locomotor activity pattern of the animals and/or that the animals were sensitized to 0.6 mg/kg MPD. (2) The 2.5 mg/kg dose group demonstrated MESOR trending up over the 11 EDs and statistical significance (p = 0.041) at the expression phase (Table 2; Fig. 6). The chronic exposure of 2.5 mg/kg MPD was able to establish a new baseline locomotor activity pattern. This is in contrast to the MESOR of the 0.6 mg/kg dose group, which also trended up, but the p value did not reach statistical significance (Table 2; Fig. 6). Perhaps, the 0.6 mg/kg MPD dose was too low and not enough MPD and, subsequently, DA accumulated in the synaptic clefts to effect a change in locomotor activity as in the 2.5 mg/kg dose group. Perhaps, more time is needed for this change in locomotor activity to manifest as statistical significance. Of note, comparing the change in amplitude of the 2.5 mg/kg dose group from ED8 to ED11 (235 → 320 average counts/10 min) to the change in amplitude from ED2 to ED7 (220 → 248 average counts/10 min), it was observed that the change in amplitude was four times greater at the expression phase than the induction phase (Table 2; Fig. 6). One could not help but wonder that perhaps a degree of sensitization was at play. (3) The 10 mg/kg dose group showed that the acrophase shifted approximately 2.5 h earlier on ED 2 (p = 0.013) (Table 2). This change in locomotor activity pattern was effected by a single 10.0 mg/kg MPD injection and persisted through six daily injections, 3 days of washout, and upon MPD rechallenge at ED 11, suggesting that, indeed, a new baseline of locomotor activity had been established. The change in acrophase also highlights the fact that it is important to analyze the data and trends fully, despite the numerical analysis suggesting statistical insignificance, i.e., acrophase of ED 11 versus 2 was p = 0.697, while the acrophase was actually shifted ~2.5 h earlier from EDs 2 to 11. While the change in the amplitude of circadian rhythm activity pattern from ED 11 versus 2 did not show a statistical significance, it may be due to the expression of tolerance, i.e., the 10 mg/kg MPD dose did not alter the activity pattern but induced tolerance, which is also a long-term effect of the drug.
Psychostimulants affecting neuroadaptation
Neuroadaptation by mesocorticolimbic dopaminergic pathway which includes the ventral tegmental area (VTA), nucleus accumbens (NAc), and the prefrontal cortex (PFC) is a major factor in the development of behavioral sensitization (Chen et al. 2009; Chong et al. 2012; Claussen and Dafny 2012; Everitt and Robbins 2005; Kalivas and Stewart 1991; Nestler 2001; Podet et al. 2010; Robinson and Becker 1986; Salek et al. 2012). Neuroadaptation is mediated by neuronal structural plasticity manifested by changes in the size of cell bodies or changes in spine morphology, such as arborization and the density of dendritic spines (Robinson and Kolb 2004; Russo et al. 2010; Sklair-Tavron et al. 1996). Chronic psychostimulant exposure leads to an increased density and complexity of spines of medium-sized spiny neurons in the NAc (Lee et al. 2006), which result in long-term potentiation (Nagerl et al. 2004; Okamoto et al. 2004; Matsuzaki et al. 2004). MPD initially interacts with dopamine and glutamine in the dendritic spines of medium-spiny neurons (MSN) in the ventral and dorsal striatum (Robinson and Kolb 2004). This study shows that the 0.6 mg/kg MPD dose failed to elicit effect following the initial MPD exposure, while eliciting increased locomotor activity amplitude only after repetitive MPD exposure at the expression phase. Perhaps, silent receptors were recruited (Boudreau et al. 2007; Boudreau and Wolf 2005; Conrad et al. 2008; Huang et al. 2009; Malenka and Nicoll 1999; Marie et al. 2005) following repetitive exposure to the drug, and the behavioral response manifested as increased amplitude.
Different brain region exhibit different levels of DA receptor types (Wirz-Justice 1987). Both D1 and D2 receptors are found in high concentration in NAc (Girault and Greengard 2004) with excitatory and inhibitory roles, respectively (Einhorn et al. 1988; Ruskin et al. 2001; Shi et al. 1997). D1-like DA receptors mediate extracellular signal-kinase (ERK) which upregulates ∆FosB, a marker of increased sensitivity to behavioral effects and increased motivation for the drug (Nestler 2001; Nestler and Malenka 2004), which is reported to induce long-term neuroadaptations in the brain (Shi and Mcginty 2010; Zhang et al. 2004). When D1 receptors are inhibited, there is an increase in phosphorylation of cAMP response element-binding protein (CREB) which leads to homeostatic negative feedback adaptation, inhibiting sensitivity, i.e., behavioral tolerance (Chao and Nestler 2004; Nestler and Malenka 2004). On the other hand, D2-like DA receptors are reported to exert an inhibitory effect (Ferguson et al. 2010; Kreitzer and Malenka 2008; Zhang et al. 2004). Of note, in this experiment, the 10.0 mg/kg MPD dose group showed MESOR trending down during the acute phase (366.37 → 358.61 average counts/10 min), further down during induction (358.61 → 303.11 average counts/10 min), up during washout (303.11 → 338.16 average counts/10 min), and, finally, down during the expression phase (338.16 → 334.97 average counts/10 min). This trend suggests that chronic exposure of 10.0 mg/kg MPD exerts an attenuation effect on the animals’ locomotor activity. Perhaps at this dosage, D2 receptors were preferentially activated. Furthermore, the magnitude of decrease in MESOR during the expression phase was less than during the induction phase, and behavior tolerance may be the underlying reason for this observation. Since chronic treatment of MPD showed an increase in locomotion, one might assume that psychostimulants not only inhibit uptake of DA by blocking the DAT, but also may increase production of D1 and/or D2 receptors (Kleven et al. 1990).
The chronic MPD effects observed in this study suggest that MPD, like cocaine, is capable of inducing both tolerance and sensitization (Izenwasser and French 2002). Sensitization was observed in this study at 0.6 and 2.5 mg/kg MPD, while tolerance was observed at 10.0 mg/kg MPD. Cocaine and amphetamine may not only inhibit uptake of DA, but also induce the production of D1 and D2 receptors (Kleven et al. 1990). Therein, the question arises as to whether chronic administration of MPD would also induce both D1 and D2 receptors, which may account for the simultaneous manifestation of opposing behavioral responses, sensitization, and tolerance.
Conclusion
After chronic treatment of MPD and a washout period, all three dose groups manifested changes in diurnal locomotor activity indices upon rechallenge with the drug. These changes in locomotor activity pattern are interpreted as long-term effect exerted by MPD. Perhaps, the behavioral changes resulted from alteration of the clock genes, regulation of transcription factors, or preferential activation of receptors. Further investigation into the mechanism of change in the circadian rhythm pattern exerted by MPD, thereby affecting the various homeostatic functions within the body, is warranted.
References
Algahim MF, Yang PB, Wilcox VT, Burau KD, Swann AC, Dafny N (2009) Prolonged methylphenidate treatment alters the behavioral diurnal activity pattern of adult male Sprague–Dawley rats. Pharmacol Biochem Behav 92:93–99
Amini B, Yang PB, Swann AC, Dafny N (2004) Differential locomotor responses in male rats from three strains to acute methylphenidate. Int J Neurosci 114:1063–1084
Antle MC, van Diep HC, Deboer T, Pedram P, Pereira RR, Meijer JH (2012) Methylphenidate modifies the motion of the circadian clock. Neuropsychopharmacology 37:2446–2455
Benstaali C, Mailloux A, Bogdan A, Auzeby A, Touitou Y (2001) Circadian rhythms of body temperature and motor activity in rodents their relationships with the light–dark cycle. Life Sci 68:2645–2656
Bergheim M, Yang PB, Burau KD, Dafny N (2012) Adolescent rat circadian activity is modulated by psychostimulants. Brain Res 1431:35–45
Bingham C, Arbogast B, Guillaume GC, Lee JK, Halberg F (1982) Inferential statistical methods for estimating and comparing cosinor parameters. Chronobiologia 9(4):397–439
Bittman EL, Doherty L, Huang L, Paroskie A (2003) Period gene expression in mouse endocrine tissues. Am J Physiol Regul Integr Comp Physiol 285:561–569
Boudreau AC, Wolf ME (2005) Behavioral sensitization to cocaine is associated with increased AMPA receptor surface expression in the nucleus accumbens. J Neurosci 25:9144–9151
Boudreau AC, Reimers JM, Milovanovic M, Wolf ME (2007) Cell surface AMPA receptors in the rat nucleus accumbens increase during cocaine withdrawal but internalize after cocaine challenge in association with altered activation of mitogen-activated protein kinases. J Neurosci 27:10621–10635
Bowman BP, Kuhn CM (1996) Age-related differences in the chronic and acute response to cocaine in the rat. Dev Psychobiol 29:597–611
Brandon CL, Steiner H (2003) Repeated methylphenidate treatment in adolescent rats alters gene regulation in the striatum. Eur J Neurosci 18(6):1584–1592
Bray MS, Young ME (2007) Circadian rhythms in the development of obesity: potential role for the circadian clock within the adipocyte. Obes Rev 8:169–181
Brecht ML, O’Brien A, van Mayrhauser C, Anglin MD (2004) Methamphetamine use behaviors and gender differences. Addict Behav 29:89–106
CDC 2011 report. Division of Human development, National Center on Birth Defects and Developmental Disabilities, Centers for Disease Control and Prevention. www.cdc.gov/ncbddd/index.html. Page last updated: December 12, 2011
Cermakian N, Sassone-Corsi P (2002) Environmental stimulus perception and control of circadian clocks. Curr Opin Neurobiol 12:359–365
Chao J, Nestler EJ (2004) Molecular neurobiology of drug addiction. Annu Rev Med 55:113–132
Chelaru MI, Yang PB, Dafny N (2012) Sex differences in the behavioral response to methylphenidate in three adolescent rat strains (WKY, SHR, SD). Behav Brain Res 226:8–17
Chen CP, Kuhn P, Advis JP, Sarkar DK (2004) Chronic ethanol consumption impairs the circadian rhythm of pro-opiomelanocortin and period genes mRNA expression in the hypothalamus of the male rat. J Neurochem 88(6):1547–1554
Chen JC, Chen PC, Chiang YC (2009) Molecular mechanisms of psychostimulant addiction. Chang Gung Med J 32:148–154
Chong SL, Claussen CM, Dafny N (2012) Nucleus accumbens neuronal activity in freely behaving rats is modulated following acute and chronic methylphenidate administration 2 204. Brain Res Bull 87:445–456
Claussen C, Dafny N (2012) Acute and chronic methylphenidate modulates the neuronal activity of the caudate nucleus recorded from freely behaving rats 4 207. Brain Res Bull 87:387–396
Conrad KL, Tseng KY, Uejima JL, Reimers JM, Heng LJ, Shaham Y, Marinelli M, Wolf ME (2008) Formation of accumbens GluR2-lacking AMPA receptors mediates incubation of cocaine craving. Nature 454:118–121
Crutchley A, Temlett JA (1999) Methylphenidate (Ritalin) use and abuse. S Afr Med J Oct 89(10):1076–1079
Dafny N, Yang PB (2006) The role of age, genotype, sex, and route of acute and chronic administration of methylphenidate: a review of its locomotor effects. Brain Res Bull 68:393–405
Ding YS, Gatley SJ, Thanos PK, Shea C, Garza V, Xu Y, Carter P, King P, Warner D, Taintor N, Park D, Pyatt B, Fowler JS, Volkow N (2004) Brain kinetics of methylphenidate (Ritalin) enantiomers after oral administration. Synapse 53:168–175
Einhorn LC, Johansen PA, White FJ (1988) Electrophysiological effects of cocaine in the mesoaccumbens dopamine system: studies in the ventral tegmental area. J Neurosci 8:100–112
Everitt BJ, Robbins TW (2005) Neural systems of reinforcement for drug addiction: from actions to habits to compulsion. Nat Neurosci 8:1481–1489
Ferguson SM, Eskenazi D, Ishikawa M, Wanat MJ, Phillips PE, Dong Y et al (2010) Transient neuronal inhibition reveals opposing roles of indirect and direct pathways in sensitization. Nat Neurosci 14(1):22–24
Filipski E, King VM, Li X, Granda TG, Mormont MC, Liu X, Claustrat B, Hastings MH, Levi F (2002) Host circadian clock as a control point in tumor progression. J Natl Cancer Inst 94:690–697
Gatley SJ, Volkow ND, Gifford AN, Fowler JS, Dewey SL, Ding YS, Logan J (1999) Dopamine-transporter occupancy after intravenous doses of cocaine and methylphenidate in mice and humans. Psychopharmacol 146:93–100
Gaytan O, Swann AC, Dafny N (1996) Effects of amphetamine at the beginning of the light cycle on multiple indices of motor activity in the rat. Eur J Pharmacol 300(1–2):1–8
Gaytan O, Al-Rahim S, Swann A, Dafny N (1997) Sensitization to locomotor effects of methylphenidate in the rat. Life Sci 61:PL101–PL107
Gaytan O, Nason R, Alagugurusamy R, Swann A, Dafny N (2000a) MK 801 blocks the development of sensitization to the locomotor effects of methylphenidate. Brain Res Bull 51:485–492
Gaytan O, Yang P, Swann A, Dafny N (2000b) Diurnal differences in sensitization to methylphenidate. Brain Res 864:24–39
Girault JA, Greengard P (2004) The neurobiology of dopamine signaling. Arch Neurol 61:641–644
Glaser AM, Reyes-Vazquez C, Prieto-Gomex B, Burau K, Dafny N (2012) Morphine administration and abrupt cessation alter the behavioral diurnal activity pattern. Pharmacol Biochem Behav 101:544–552
Greely H, Sahakian B, Harris J, Kessler RC, Gazzaniga M, Campbell P, Farah MJ (2008) Towards responsible use of cognitive-enhancing drugs by the healthy. Nature 456:702–705
Hernandez-Avila CA, Rounsaville BJ, Kranzler HR (2004) Opioid-, cannibis-, and alcohol-dependent women show more rapid progression to substance abuse treatment. Drug Alcohol Depend 74:265–272
Huang YH, Lin Y, Mu P, Lee BR, Brown TE, Wayman G, Marie H, Liu W, Yen Z, Sorg BA, Schluter OM, Zukin RS, Dong Y (2009) In vivo cocaine experience generates silent synapses. Neuron 63:40–47
Iijima M, Nikaido T, Akiyama M, Moriya T, Shibata S (2002) Methamphetamine-induced, suprachiasmatic nucleus-independent circadian rhythms of activity and mPer gene expression in the striatum of the mouse. Eur J Neurosci 16:921–929
Izenwasser S, French D (2002) Tolerance and sensitization to the locomotor-activating effects of cocaine are mediated via independent mechanisms. Pharmacol Biochem Behav 73:877–882
Izenwasser S, Coy AE, Ladenheim B, Loeloff RJ, Cadet JL, French D (1999) Chronic methylphenidate alters locomotor activity and dopamine transporters differently from cocaine. Eur J Pharmacol 373:187–193
Janssen BJA, Tyssen CM, Duindam H, Rietweld WJ (1994) Suprachiasmatic lesions eliminate 24-h blood pressure variability in rats. Physiol Behav 55:307–311
Johnson RF, Moore RY, Morin LP (1988) Loss of entrainment and anatomical plasticity after lesions of the hamster retinohypothalamic tract. Brain Res 460:297–313
Kalivas PW, Stewart J (1991) Dopamine transmission in the initiation and expression of drug- and stress-induced sensitization of motor activity. Brain Res Rev 16:223–244
Kallman WM, Isacc W (1975) The effects of age and illumination on the dose–response curve for three stimulants. Psychopharmacology (Berlin) 40:313–318
Karlsson B, Knutsson A, Lindahl B (2001) Is there an association between shift work and having a metabolic syndrome? Results from a population based study of 27,485 people. Occup Environ Med 58:747–752
Kelly SJ, Ostrowski NL, Wilson MA (1999) Gender differences in brain and behavior: hormonal and neural bases. Pharmacol Biochem Behav 64:655–664
Klein DC, Moore RY, Reppert SM (1991) Suprachiasmaic nucleus: the mind’s clock. Oxford UP, New York ISBN-13: 978-0195062502
Kleven MS, Perry BD, Woolverton WL, Seiden LA (1990) Effects of repeated injections of cocaine on D1 and D2 dopamine receptors in rat brain. Brain Res 532:265–270
Kollins SH, MacDonald EK, Rush CR (2001) Assessing the abuse potential of methylphenidate in nonhuman and human subjects: a review. Pharmacol Biochem Behav 68(3):611–627
Kreitzer AC, Malenka RC (2008) Striatal plasticity and basal ganglia circuit function. Neuron 60(4):543–554
Kuczenski R, Segal DS (2002) Exposure of adolescent rats to oral methylphenidate: preferential effects on extracellular norepinephrine and absence of sensitization and cross-sensitization to methamphetamine. J Neurosci 22(16):7264–7271
Lamont EW, James FO, Boivin DB, Cermakian N (2007) From circadian clock gene expression to pathologies. Sleep Med 8:547–556
Lee KW, Kim Y, Kim AM, Helmin K, Nairn AC, Greengard P (2006) Cocaine-induced dendritic spine formation in D1 and D2 dopamine receptor-containing medium spiny neurons in nucleus accumbens. Proc Natl Acad Sci USA 103:3399–3404
Lee MJ, Swann AC, Dafny N (2008) Methylphenidate sensitization is prevented by prefrontal cortex lesion. Brain Res Bull 76:131–140
Lee MJ, Yang PB, Wilcox VT, Burau KD, Swann AC, Dafny N (2011) Repetitive methylphenidate administration modulates the diurnal behavioral activity pattern of adult female SD rats. J Neural Transm 118:285–298
Lynch WJ (2008) Acquisition and maintenance of cocaine self-administration in adolescent rats: effects of sex and gonadal hormone. Psychopharmacology 197:237–246
Malenka RC, Nicoll RA (1999) Long-term potentiation—a decade of pregress? Science 285:1870–1874
Manev H, Uz T (2006) Clock genes: influencing and being influenced by psychoactive drugs. Trends Pharmacol Sci 27(4):187–189
Marie H, Morishita W, Yu X, Calakos N, Malenka RC (2005) Generation of silent synapses by acute in vivo expression of CaMKIV and CREB. Neuron 45:741–752
Massello WI, Carpenter DA (1999) A fatality due to the intranasal abuse of methylphenidate (Ritalin). J Forensic Sci 44:220–221
Matsuzaki M, Honkura N, Ellis-Davis GC, Kasai H (2004) Structural basis of long-term potentiation in single dendritic spines. Nature 429:761–766
McCabe SE, Knight JR, Teter CJ, Wechsler H (2005) Non-medical use of prescription stimulants among US college students: prevalence and correlates from a national survey. Addiction 100:96–106
Melnick SM, Dow-Edwards DL (2001) Differential responses to chronic amphetamine in adult male and female rats exposed to postnatal cocaine treatment. Pharmacol Biochem Behav 69:219–234
Mistleberger RE (2005) Circadian regulation of sleep in mammals: role of the suprachiasmatic nucleus. Brain Res Brain Res Rev 49:429–454
Moore RY (1983) Organization and function of a central nervous system circadian oscillator: the suprachiasmatic hypothalamic nucleus. Fed Proc 42(11):2783–2789
Moore RY, Lenn NJ (1972) A retinohypothalamic projection in the rat. J Comp Neurol 146:1–14
Nagerl UV, Eberhorn N, Cambridge SB, Bonhoeffer T (2004) Bidirectional activity-dependent morphological plasticity in hippocampal neurons. Neuron 44:759–767
Nestler EJ (2001) Molecular basis of long-term plasticity underlying addiction. Nat Rev Neurosci 2:119–128
Nestler EJ, Malenka RC (2004) The addicted brain. Sci Am 290:78–85
Okamoto KI, Nagai T, Miyawaki A, Hayashi Y (2004) Rapid and persistent modulation of actin dynamics regulates postsynaptic reorganization underlying bidirectional plasticity. Nat Neurosci 7:1104–1112
Patrick KS, Markowitz JS (1997) Pharmacology of methylphenidate, amphetamine enantiomers, and penoline in attention deficit/hyperactivity disorder. Hum Psychopharmacol 12:527–546
Podet A, Lee MJ, Swanda AC, Dafny N (2010) Nucleus accumbens lesion modulates the effects of methylphenidate. Brain Res Bull 82:293–301
Reid K, Burgess H (2005) Circadian rhythm sleep disorders. Prim Care 32(2):449–473
Reppert SM, Weaver DR (2002) Coordination of circadian timing in mammals. Nature 418:935–941
Robinson TE, Becker JB (1986) Enduring changes in brain and behavior produced by chronic amphetamine administration: a review and evaluation of animal models of amphetamine psychosis. Brain Res 396:157–198
Robinson TE, Kolb B (2004) Structural plasticity associated with exposure of drugs of abuse. Neuropharmacology 47(Suppl 1):33–46
Ruskin DN, Bergstrom DA, Shenker A, Freeman LE, Baek D, Walters JR (2001) Drugs used in the treatment of attention-deficit/hyperactivity disorder affect postsynaptic firing rate and oscillation without preferential dopamine autoreceptor action. Biol Psychiatry 49:340–350
Russo SJ, Dietz DM, Dumitriu D, Morrison JH, Malenka RC, Nestler EJ (2010) The addicted synapse: mechanisms of synaptic and structural plasticity in nucleus accumbens. Trends Neurosci 33:267–276
Salek RL, Claussen CM, Perez A, Dafny N (2012) Acute and chronic methylphenidate alters prefrontal cortex neuronal activity recorded from freely behaving rats. Eur J Pharmacol 679:60–67
Shi X, McGinty JF (2010) D1 and D2 dopamine receptors differentially mediate the activation of phosphoproteins in the striatum of amphetamine-sensitized rats. Psychopharmacology 214(3):653–663
Shi WX, Zheng P, Liang XF, Bunney BS (1997) Characterization of dopamine-induced depolarization of prefrontal cortical neurons. Synapse 26:415–422
Sklair-Tavron L, Shi WX, Lane SB, Harris HW, Bunney BS, Nestler EJ (1996) Chronic morphine induces visible changes in the morphology of mesFolimbic dopamine neurons. Proc Natl Acad Sci USA 93:11202–11207
Solanto MV (1998) Neuropsychopharmacological mechanisms of stimulant drug action in attention deficit hyperactivity disorder: a review and integration. Behav Brain Res 94(1):127–152
Solanto MV (2000) Clinical psychopharmacology of AD/HD: implications for animal models. Neurosci Biobehav Rev 24(1):27–30
Stix G (2009) Turbocharging the brain. Sci Am. 301(4):46–49 52–55
Sujino M, Masumoto KH, Yamaguchi S, van der Horst GT, Okamura H, Inouye ST (2003) Suprachiasmatic nucleus grafts restore circadian behavioral rhythms of genetically arrhythmic mice. Curr Biol 13:664–668
Vitaterna MH, Takahashi JS, Turek FW (2001) Overview of circadian rhythms. Alcohol Res Health 25:85–93
Volkow ND, Wang GJ, Fowler JS, Logan J, Wong C, Hitzemann R, Papas NR (1999) Reinforcing effects of psychostimulants in humans are associated with increases in brain dopamine and occupancy of D2 receptors. J Pharmacol Exp Ther 291:409–415
Wanchoo SJ, Swann AC, Dafny N (2009) Descendin glutamatergic pathway of PFC are involved in acute and chronic action of methylphenidate. Brain Res 1301:68–79
Welsh DK, Logothetis DE, Meister M, Reppert S (1995) Individual neurons dissociated from rat suprachiasmatic nucleus express independently phased circadian firing rhythms. Neuron 14:697–706
White SR, Yadao CM (2000) Characterization of methylphenidate exposures reported to a regional poison control center. Arch Pediatr Adolesc Med 154(12):1199–1203
Wirz-Justice A (1987) Circadian rhythms in mammalian neurotransmitter receptors. Prog Neurobiol 29:219–259
Yamazaki S, Numano R, Abe M, Hida A, Takahashi R, Ueda M, Block GD, Sakaki Y, Menaker M, Tei H (2000) Resetting central and peripheral circadian oscillators in transgenic rats. Science 288:682–685
Yang PB, Beasley A, Swann A, Dafny N (2000a) Valproate modulates the expression of methylphenidate (Ritalin) sensitization. Brain Res 874:216–220
Yang PB, Swann A, Dafny N (2000b) NMDA receptor antagonist disrupt acute and chronic effects of methylphenidate. Physiol Behav 71:133–145
Yang PB, Singhal N, Modi G, Swann A, Dafny N (2001) Effects of lithium chloride on induction and expression of methylphenidate sensitization. Eur J Pharmacol 426(1–2):65–72
Yang PB, Amini B, Swann AC, Dafny N (2003) Strain differences in the behavioral responses of male rats to chronically administered methylphenidate. Brain Res 971(2):139–152
Yang PB, Swann A, Dafny N (2006a) Dose response characteristics of methylphenidate on locomotor behavior and on sensory evoked potentials recorded from VTA, NAc and PFC in freely behaving rats. Behav Brain Funct 4:25–36
Yang PB, Swann AC, Dafny N (2006b) Acute and chronic methylphenidate dose–response assessment on three adolescent male rat strains. Brain Res Bull 71(1–3):301–310
Yang PB, Swann AC, Dafny N (2007) Chronic administration of methylphenidate produces neurophysiological and behavioral sensitization. Brain Res 1145:66–80
Yang PB, Cuellar DO III, Swann AC, Dafny N (2011) Age and genetic strain differences in response to chronic methylphenidate administration 103. Behav Brain Res 218:206–217
Zhang L, Lou D, Jiao H, Zhang D, Wang X, Xia Y et al (2004) Cocaine-induced intracellular signaling and gene expression are oppositely regulated by the dopamine D1 and D3 receptors. J Neurosci 24(13):3344–3354
Acknowledgments
We would like to thank Mallinckrodt for their donation of methylphenidate. This research was supported in part by NIH R01 DA027222. All of the authors declare no conflict of interest.
Author information
Authors and Affiliations
Corresponding author
Rights and permissions
About this article
Cite this article
Trinh, T.N., Kohllepel, S.R., Yang, P.B. et al. Adult female rats' altered diurnal locomotor activity pattern following chronic methylphenidate treatment. J Neural Transm 120, 1717–1731 (2013). https://doi.org/10.1007/s00702-013-1063-4
Received:
Accepted:
Published:
Issue Date:
DOI: https://doi.org/10.1007/s00702-013-1063-4