Abstract
The objective of this study was to evaluate the impact of thermal stress on milk production and physiological traits of Baladi goats under subtropical Egyptian conditions. Sixty dairy Baladi goats were exposed to three different levels of temperature-humidity index (THI), including low (less than 70), moderate (over 70 and up to 80), and high levels (over 80). The influence of THI on the milk composition and physiological, hematological, and biochemical traits was investigated. Rectal temperature and respiration rate were significantly greater at the higher THI than at low and moderate THI (p = 0.016 and 0.002, respectively). Baladi goats had decreased daily milk yield in a rate of 27.3 and 19.3 % at high THI level, compared with low and moderate THI, respectively (p = 0.031). On the contrary, no significant differences have been reported in protein, fat, and total solids percentages at different THI levels. Total leucocyte count, serum glucose, and total protein were significantly reduced at high THI in comparison with low and moderate THI levels (p = 0.043, 0.001, and 0.001, respectively). However, dairy goats maintained relatively stable estimates for erythrocytes count, hemoglobin, serum triglycerides, cholesterol, catalase, total antioxidant capacity, and triiodothyronine at different THI levels. Our results indicate that dairy Baladi goats can tolerate THI levels up to 80; however, variable reduction in milk yield and few biochemical (serum total protein and glucose) and hematological (leucocytes count) parameters have been reported at a THI level higher than 80.
Similar content being viewed by others
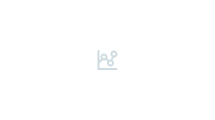
Avoid common mistakes on your manuscript.
Introduction
For thousands of years, goats are among the earliest domesticated farm animals, and their milk has been consumed by humans; however, evidence of using goat’s milk for making dairy products goes back to ancient Egypt (Edelstein 2014). On a global scale, the majority of goat milk production is obtained in developing countries where goat’s milk plays a significant role in the sustenance of millions of human beings (FAO 2013). It was recently concluded that goat will continue to have an important role in harsh conditions as well as tropical, subtropical, desert, and Mediterranean environments (Silanikove and Koluman 2015). Goat breeds living in tropical and desert environments are considered as the most efficient ruminants that adjust to such areas, where an adaptive capacity of a species is defined by its ability to cope with climate change by adopting different strategies (Silanikove 2000).
Stress is the response of the body to a stimulus that disturbs homeostasis, usually associated with harmful effects (David et al. 1990). Domestic animals experience various forms of stress such as chemical, physical, nutritional, psychological, and thermal exertion. High ambient temperature is considered as the major constraint on animal production in tropical and subtropical zones (Nardone et al. 2010). Heat stress reduces milk yield of dairy animals; however, half of this decrease in milk yield is due to decreased DMI (Rhoads et al. 2009). The other half of milk production losses might be demonstrated by the increment in maintenance requirements (NRC 2007), reduction in the secretion of growth hormone, and dropping blood influx to the udder (Lough et al. 1990), upregulating the activity of apoptosis genes in the mammary tissues and downregulating the expression of milk protein genes (Collier et al. 2006).
Heat tolerance is known as the ability of the animals to preserve expression of their hereditary functional potential during their life-time when raised under hot conditions (Marai and Haeeb 2010). Numerous studies have been performed to establish comfortable zone and heat tolerance thresholds in dairy animals on the basis of temperature-humidity index (THI) values (Kadzere et al. 2002; Finocchiaro et al. 2005; El-Tarabany and El-Tarabany 2015). The temperature-humidity index allows the integration of temperature and humidity into one value, which allows an objective comparison of environmental conditions. Recently, the index is broadly used, allowing the investigators to understand better the effects of thermal conditions. However, the index should not be handled as the absolute estimate to determine thermal nuisance. The reduction in the profit of dairy farms subjected to heat stress (THI is extremely high) is not only a sequel of reduced milk production but also includes deteriorated milk quality, augmented healthcare costs, reproduction problems, and even animal loss. In spite of the large number of studies achieved in dairy cows, relatively little is recognized about the effects of thermal pressure in dairy goats. Silanikove (1997) stated that goats are the best adapted animals to harsh hot environments among the domestic ruminant species. Moreover, goats have been considered to be more tolerant at high THI values compared with dairy cows due to their higher capacity in water conservation and related metabolic size (Silanikove 2000). Brown et al. (1988) reported that Alpine goats exposed to moderate level of heat stress for 5 weeks (THI = 79) have had lowered milk yield, but not in Nubian goats.
Besides having an effect on milk yield, heat stress could also alter the milk composition. In Mediterranean dairy sheep, however, Finocchiaro et al. (2005) observed a decrease of daily fat-plus-protein production by 8.6 g (4.4 %) per unit increase of the THI value registered at the day before the test day over the threshold of 23. Furthermore, Menéndez-Buxadera et al. (2012) reported losses in fat plus protein yield, as a response to heat stress, which represent up to 1.9 and 3.1 % of annual fat-plus-protein yields of Payoya and Murciano-Granadina dairy goats, respectively. However, Robertshaw (1982) reported that black Bedouin goats sweat at a higher rate, can lose more water, and preserve a greater body temperature without harmful effects than Saanen goats, denoting that the response to heat stress differs according to breed. Therefore, the objective of the present study was to monitor the physiological and lactation responses to thermal stress conditions in dairy Baladi goats.
Materials and methods
The current work was approved by the Committee of Animal Care and Welfare, Zagazig University, Egypt (ANWD-206). The experiment was carried out at the experimental farm of the Nuclear Research Center, Atomic Energy Authority, Inshas, Egypt.
Animals and management
Sixty Baladi goats (33.5 ± 2.6 kg of BW) were selected from the experimental farm of the Nuclear Research Center. The study was conducted for a period of 6 months (February–July). The selected dairy goats are multiparous (2nd parity) with healthy and symmetrical udders and used at early lactation (45 ± 1.7 days in milk (DIM); 1.13 ± 0.07 L/day). Goats were kept in an open shelter all over the experimental period, providing 4 m2 of shaded slatted floor and 4 m2 of concrete-surfaced yard/goat. Goats were fed on a high-quality total mixed ration composed of alfalfa hay, wheat straw, and concentrate according to their BW requirements (NRC 2007). The hay, straw, and concentrate were in a 35:20:45 ratio. The concentrate contained yellow corn, solvent-extracted soybean meal, corn gluten, undecorticated cottonseed cake, rice bran, sugarcane molasses, and a vitamin and mineral mix. The DM chemical composition was determined by standard protocol (AOAC 1990; Table 1). Feed was offered in mangers (feeder space per animal = 0.4 m), and water in the shades was available at all times.
Metrological data and physiological responses
The THI is a unique value allowing the integration of temperature and humidity into one value, which allows an objective comparison of climatic conditions. This index has been developed as a weather safety index to adjust and reduce heat stress-related losses. The daily ambient temperature and relative humidity in the farm area were collected from the local meteorological station, approximately 12 km far away. These raw data were used to calculate the daily THI, using a previously reported equation (Kendall and Webster 2009). THI = (1.8 × AT + 32) − [(0.55 − 0.0055 × RH) × (1.8 × AT − 26)], where AT is the air temperature (°C) and RH is the relative humidity (%). The monthly average temperature and THI is shown in Fig. 1. To investigate the influence of the THI on production and physiological performance, all studied variables were allotted according to the THI into the following: low THI, which includes the months with average less than 70 (February and March); moderate THI, which includes the months with average over 70 and up to 80 (April and May); and high THI, which includes the months with average over 80 (June and July). Regarding physiological responses, rectal temperature (RT), skin temperature (ST) and respiration rate (RR) were monitored once daily at 12:00 h to explore adaptive physiological response at the point of maximum THI in this area. Also, we avoid repeated measures to avoid stress of excessive handling. Rectal and ST temperatures were measured by a clinical thermometer (ICO Technology, Spain; range, 34 to 43.7 °C; accuracy, ±0.1 °C), whereas the RR was expressed as the number of inhalations and exhalations during 1 min.
Milk yield and composition
All dairy goats were examined daily to explore the presence or confirm the absence of clinical signs of mastitis, such as fever, painful reaction, or gland swelling. Daily milk yield of individual goats was recorded throughout the study; however, milk composition was evaluated weekly. Animals were hand-milked once daily (08:00 h) with two skillful milkers who have relatively the same efficiency (speed, gentle handling, and efficient evacuation of the udder). After hand milking, yield of each goat was determined by weighing the milk. Milk samples (approximately 100 ml) were collected, protected by means of Broad Spectrum Microtabs II, and preserved at 4 °C until analysis of the milk gross composition: protein, fat, and lactose contents (MilkoScan 6000; Foss Electric A/S, Hillerød, Denmark). Milk solids were determined according to Cipolat-Gotet et al. (2013).
Hematological and biochemical parameters
Biweekly, two blood samples were collected from each animal via the jugular vein puncture, before the morning feeding. The first blood sample (3 ml) was collected into an EDTA tube. In order to evaluate the hematological parameters, an automated analyzer (Autolyser AL 820, Swiss) was used to measure red blood cell (RBC) counts, hemoglobin (Hb) concentration, and total leucocyte count (WBC). The second blood sample was utilized to harvest serum samples and preserved at −20 °C. Serum total protein was measured by the biuret method (Armstrong and Carr 1964). Serum cholesterol concentration was estimated colorimetrically as mentioned by Watson (1960). Glucose was measured according to Barham and Trinder (1972). The RBC hemolysate was used to determine the activity of total antioxidant capacity (TAC) and was expressed as millimoles per deciliter (El-Deeb and Younis 2009). The activity of catalase was evaluated by colorimetric method using Catalase Assay Kit, Oxford Biochemical Research, Inc., USA (Asri-Rezaei and Dalir-Naghadeh 2006). Activities of the enzymes were expressed as units per gram of Hb. The concentrations of triiodothyronine (T3) in blood serum samples were measured by RIA using coated tubes kit; DSL, Inc. (Webster, Texas, USA). The lower limit of detection [95 % binding/0 binding (B/B°)] was 0.16 ng/ml of serum, and the intra-assay and interassay CVs were 6.1 and 5.3 %, respectively.
Statistical analysis
All statistical steps were conducted using the statistical system Package of SAS, V9.1 (SAS 2003). The Kolmogorov-Smirnov test was used to test the data for non-normality. The analytical procedures of data distribution indicated that all parameters analyzed matched the normal distribution (p > 0.05). The MIXED procedure of SAS was used to analyze the repeatedly measured variables (physiological traits, milk yield and composition, biochemical and hematological parameters). The statistical model included the fixed effect of THI and the random effects of DIM and the dairy animal. Proc COR procedures were used to estimate the Pearson’s correlations between THI values and the different hematological and biochemical parameters.
Results
Effect of THI on physiological traits in Baladi goats
Data summarizing significant results for the effect of THI on physiological traits are included in Table 2. Respiration rate (p = 0.016) and RT (p = 0.002) were significantly greater at the higher THI than at low and moderate THI. Furthermore, higher ST was reported at high THI in comparison with low and moderate THI (+2.75 and 1.67 °C, respectively; p = 0.001).
Effect of THI on milk yield and composition in Baladi goats
Data summarizing significant results for the effect of THI on milk production traits are included in Table 3. Baladi goats had decreased daily milk yield (MY) in a rate of 27.3 and 19.3 % at a high THI level, compared with low and moderate THI, respectively (p = 0.031). Furthermore, lactose (p = 0.001) and solids-not-fat (p = 0.003) percentages were significantly decreased at high THI in comparison with low and moderate THI. On the contrary, no significant differences have been reported in protein (p = 0.954), fat (p = 0.868), and total solid (p = 0.185) percentages at different THI levels.
Effect of THI on hematological and biochemical parameters in Baladi goats
Data summarizing significant results for the effect of THI on hematological and biochemical parameters in Baladi goats are presented in Table 4. Total leucocyte count (p = 0.043), serum glucose (p = 0.001), and total protein (p = 0.001) were significantly reduced at high THI in comparison with low and moderate THI levels. However, no significant differences were observed in total leucocyte count and serum glucose level among low and moderate THI. Furthermore, no significant differences have been reported in erythrocyte count (p = 0.442), hemoglobin (p = 0.248), serum triglycerides (p = 0.528), cholesterol (p = 0.635), catalase (p = 0.953), total antioxidant capacity (p = 0.115), and triiodothyronine (p = 0.483) at different THI levels.
Significant correlation estimates between THI values and the different hematological and biochemical parameters were summarized in Table 5. There were significant positive correlations between the THI values and total leucocyte count (r = 0.25, p = 0.012) and total antioxidant capacity (r = 0.21, p = 0.037). In contrast, THI values were negatively correlated with serum glucose (r = −0.37, p = 0.013) and total protein (r = −0.66, p = 0.0101).
Discussion
The primary objectives of this study were to explore the effect of THI on physiological responses and lactation performance of dairy Baladi goats under stressful subtropical conditions. Climate condition may reveal negative impacts on livestock health, performance, reproduction, and welfare (Nardone et al. 2006; El-Tarabany and El-Bayoumi 2015). Furthermore, dairy goats in tropical and subtropical areas are exposed to high ambient temperatures and relative humidity for long periods. However, THI is considered as sensitive indicator for the stressful climatic conditions (Silanikove 2000). The exposure of dairy goats to moderate heat stress conditions (THI = 79) depressed milk yield in Alpine but not in Nubian goats, indicating that the response to heat stress varies according to breed (Brown et al. 1988).
Respiration rate in the low and moderate THI conditions was comparable to the reference RR of adult goats, which fluctuated between 15 and 30 breaths per minute (Pugh and Baird 2012). However, RR at the higher THI in our trial was lower than that reported by Srikandakumar et al. (2003), who reported a value of 128 ± 2.5 RR for animals exposed to high temperatures, probably due to higher THI rates (93 ± 3.1). Similarly, Darcan and Güney (2002) showed that RR increased when the ambient temperature rose up to 30 °C. Under heat stress conditions, increased RR is an established mechanism for dissipating thermal load by evaporation (Hamzaoui et al. 2013). However, Devendra (1987) reported that changes in the respiration rate of heat-stressed goats could be attributed to the changes in metabolism and muscle activity. Rectal temperature is an important index of thermal balance and could be used to assess the influence of heat stress (Spiers et al. 2004). Elevated RT is a natural mechanism by which animals dissipate heat from their bodies to keep thermoregulation in hot environment (Yousef 1985), or it is an indicator of increased body temperature (Avendano-Reyes et al. 2006). In the present experiment, RTs at the low and moderate THI levels were comparable to the normal estimates reported previously in dairy goats (Devendra 1987; Avendano-Reyes et al. 2006). Those former trials concluded that goats kept in a comfort zone can maintain their rectal temperatures below 38.5 °C. However, the increment of RT at the high THI was in accordance with observations of Phulia et al. (2010), who reported increment in RT from 38.97 to 39.35 °C when goats are exposed to a hot ambient temperature for 6 h.
Increasing the environmental temperature is associated with production losses in livestock enterprises which is a part of the ongoing and predicted climate change (Gaughan et al. 2009; El-Tarabany and Nasr 2015). Our results clearly state that Baladi goats can tolerate at a moderate THI level; however, they revealed a reduction in daily MY at a THI level higher than 80 (severe stress zone). Avendano-Reyes et al. (2006) demonstrated that the homoeothermic ability of lactating goats starts to be deteriorated when the THI value exceeds 80. According to Sevi et al. (2001), milk yield only reduces in lactating ewes when they are subjected to a THI greater than 80. Saanen goats subjected to moderate or high heat stress, at THI 81 and 89, lost their daily milk yield by 3 or 13 %, respectively (Sano et al. 1985). Brown et al. (1988) reported that Alpine goats exposed to moderate level of heat stress for 5 weeks (THI = 79) have had lowered milk yield but not in Nubian goats, denoting that the response to thermal stress varies according to breed.
The heat-stressed goats experience increased maintenance requirements necessary for extra activities such as muscle movements for panting, greater sweating, increased chemical reactions in the body, and the production of heat shock proteins that consumes large amounts of ATP (Salama et al. 2014). According to NRC (2007), maintenance requirements of heat-stressed animals have increased by 30 %. Consequently, energy intake fails to cover the daily requirements for milk production, especially in early lactating goats. Feed intake reduces in heat-stressed ewes due to both the effort exerted for reduction of heat production and the relatively slower transition of feed through the digestive tract (Christopherson 1985). Under these circumstances, body reserves of fat and nitrogen are utilized to furnish energy through gluconeogenesis process at the expense of the mammary tissues (Amaral-Phillips et al. 1993). In another systemic mechanism, stress activates the HPA axis and stimulates the secretion process of adrenocorticotropic hormone (ACTH) by the anterior pituitary. The ACTH enhances the synthesis and emission of glucocorticoids from the cortex of the adrenal gland. Cortisol causes a decrease in milk synthesis by blocking the uptake of glucose by the mammary gland (Dwyer and Bornett 2004). A secondary effect of stress is the inhibition of prolactin synthesis by the pituitary gland due to the hypothalamic release of dopamine (Kandemir et al. 2013).
In the current study, milk of the dairy Baladi goats had steady and symmetric protein and fat percentages at different THI levels. These results support other short-term trials achieved using climate-controlled heat stress, where milk fat was not changed in cows (Rhoads et al. 2009) despite the established negative impact of summer temperature on milk fat (Kadzere et al. 2002). On the contrary, protein percentage showed a linear decline of −0.03 % per unit increase of THI over the entire scale (Finocchiaro et al. 2005). The heat-stressed goats produced milk with lesser protein percentage (−6.3 %) compared with the thermoneutral group (Brasil et al. 2000). Decreased protein intake and increased sweat secretion that contains protein and urea might have limited the availability of amino acids for milk protein synthesis (Joshi et al. 1968). However, Bernabucci et al. (2002) stated that decreased mammary synthesis of milk protein is the reason for the low milk protein during the hot season rather than the reduction in amino acid intake. Lactose is one of the most critical components in milk that displays disturbances in secretory tissues (Gajdusek 1996). Our results revealed that lactose percentage in dairy goats has been reduced dramatically as the THI value increased. This was in agreement with previous report which suggested that the heat-stressed goats produced milk with lesser lactose (−1 to −5 %) contents (Salama et al. 2014).
The current results revealed stability in the most hematological and biochemical parameters of Baladi goats at different THI levels. However, total leucocytes, serum glucose, and total protein were reduced at a high THI level (severe heat stress). The reduced leucocyte count at high THI level was in accordance with previous reports (Wojtas et al. 2014). This may be attributed to the increment in cortisol level, which probably is responsible for the impairment of the cellular immune response (Caroprese et al. 2012). On the contrary, Uwayjan and Bahattacharya (1975) showed an increase in leucocyte count in animals exposed to thermal stress. The reduced serum total protein at the high THI level was supported by other trials conducted on dairy goats (Dangi et al. 2012). The total plasma protein reduced from 6.56 to 5.88 g/dl in Baladi goats exposed to short-term thermal stress for 2 days (Helal et al. 2010). This may be attributed to the increment in plasma volume as a sequel of heat shock which results in decreased protein concentration. On the contrary, More et al. (1980) reported a considerable increase in the serum protein of sheep exposed to thermal stress. The increment in serum protein could be a physiological trial to maintain extended plasma volume.
Physiologically, blood glucose level is an adaptation mechanism that can be influenced by high ambient temperatures. Blood glucose level shows greater variations in a hot climate than in the comfort zone. Dairy goats in this experiment maintained a relatively stable level of serum glucose at thermoneutral and moderate THI levels; however, significant reduction was reported at a high THI level. There have been conflicting results on serum glucose level with different environmental condition. Some researchers demonstrated that hot environmental conditions reduce blood glucose level (Ocak and Guney 2010), and other results are contrary to these (Webster 1976). Ocak and Guney (2010) reported that the level of blood glucose has been decreased during summer season in dairy goats. On the contrary, Hamzaoui et al. (2013) reported no significant decrease in milk yield in heat-stressed lactating goats, probably because glucose was sufficient for the milk yield level at late lactation. Also, this may be attributed to some degree of body muscle degradation under heat stress and the resultant amino acids could be utilized for gluconeogenesis (Wheelock et al. 2010).
Thyroid hormones stimulate oxygen consumption and cellular ability for heat production, which elevate basal metabolic rate (Ocak et al. 2009). Therefore, it is logical that the concentration of these hormones is decreased under thermal stress conditions to reduce animal heat production as previously observed by Helal et al. (2010) in Damascus and Baladi goats. In the current trial, Baladi goats able are to maintain a relatively stable level of T3 at different THI values. Such stability plays an important role in regulating energy balance (Hefnawy et al. 2011) and maintaining feed intake (Todini 2007) at different thermal zones. The seasonal pattern of blood T3 levels often showed maximal values during cold months and minimal during hot months (Menegatos et al. 2006). However, contrasting results reported that T3 level has been increased in hot seasons either in dry or lactating ewes (Ashutosh et al. 2001). Thyroid function is reduced as an adaptation response to mitigate thermal stress. This reduction could be attributed to the influence of heat on the hypothalamo-pituitary-adrenal cortical axis to minimize the release of thyrotropin hormone which enables an animal to reduce basal metabolic rate (Johnson 1987).
Conclusion
Dairy Baladi goats can relatively cope with the adverse subtropical climate in Egypt. Furthermore, they were able to maintain most physiological and production parameters at THI up to 80; however, variable reduction in milk yield and few biochemical (serum total protein and glucose) and hematological (leucocytes count) indices have been reported at a THI level higher than 80. The results from the study point towards developing appropriate strategies to alleviate negative impacts of thermal stress on dairy Baladi goats, mostly at a THI level greater than 80.
References
Amaral-Phillips DM, McGilliarg AD, Lindberg GL, Veenhuizen JJ, Yang JW (1993) Effects of decreased availability of glucose for dairy cows. J Dairy Sci 76:752–761
AOAC (1990) Official methods of analysis, 15th edn. AOAC, Arlington
Armstrong WD, Carr CW (1964) Physiological chemistry laboratory directions, 3rd edn. Burges Publishing Co., Minneapolis
Ashutosh OP, Dhanda OP, Kundu RL (2001) Effect of climate on the seasonal endocrine profile of native and crossbred sheep under semi-arid conditions. Trop Anim Health Prod 33:241–252
Asri-Rezaei S, Dalir-Naghadeh B (2006) Evaluation of antioxidant status and oxidative stress in cattle naturally infected with Theileria annulata. Vet Parasitol 142:179–186
Avendano-Reyes L, Alvarez-Valenzuela FD, Correa-Clederon A, Saucedo-Quintero JS, Robinson PH (2006) Effect of cooling Holstein cows during the dry period on postpartum performance under heat stress conditions. Livest Sci 105:198–206
Barham D, Trinder P (1972) Improved colour reagent for determination of blood glucose by oxidase system. Analyst 44:223–231
Bernabucci U, Lacetera N, Ronchi B (2002) Effects of the hot season on milk protein fractions in Holstein cows. Anim Res 51:25–33
Brasil LHA, Wechesler FS, Junior F, Goncalves HC, Bonassi IA (2000) Efeitos do Estresse Termico Sobre a Produc, ao, Composic, ao Quimica do Leite e Respostas Termorreguladoras de Cabras da Rac, a Alpina. Rev Bras Zootec 29:1632–1641
Brown DL, Morrison SR, Bradford GE (1988) Effects of ambient temperature on milk production of Nubian and Alpine goats. J Dairy Sci 71:2486–2490
Caroprese M, Albenzio M, Bruno A, Annicchiarico G, Marino R, Sevi A (2012) Effects of shade and flaxseed supplementation on the welfare of lactating ewes under high ambient temperatures. Small Rumin Res 102:177–185
Christopherson RJ (1985) The thermal environment and the ruminant digestive system. In: Stress physiology in Livestock. Vol. I. Basic principles. M. K. Yousef, CRC Press, Boca Raton, p 163–177
Cipolat-Gotet C, Cecchinato A, De Marchi M, Bittante G (2013) Factors affecting variation of different measures of cheese yield and milk nutrient recovery from an individual model cheese manufacturing process. J Dairy Sci 96:7966–7979
Collier RJ, Stiening CM, Pollard BC, VanBaale MJ, Baumgard LH, Gentry PC, Coussens PM (2006) Use of gene expression microarrays for evaluating environmental stress tolerance at the cellular level in cattle. J Anim Sci 84 (E. Suppl.): E1–E13
Dangi SS, Gupta M, Maurya D, Yadav VP, Panda RP, Singh G, Mohan NH, Bhure SK, Das BC, Bag S, Mahapatra RK, Sarkar M (2012) Expression profile of HSP genes during different seasons in goats (Capra hircus). Trop Anim Health Prod 44:1905–1912
Darcan N, Güney O (2002) Effect of spraying on growth and feed efficiency of kids under subtropical climate. Small Rumin Res 43:189–190
David K, Murgo AJ, Faith RE (1990) Effects of stress on the immune system. Immunol Today 11:170–179
Devendra C (1987) Goats. In: Johnson HD (ed) Bioclimatology and the adaptation of livestock. Elsevier, Amsterdam, pp 157–167
Dwyer CM, Bornett HL (2004) Chronic stress in sheep: assessment tools and their use in different management conditions. Anim Welf 13:293–304
Edelstein S (2014) Food science—an ecological approach. Jones & Bartlett, Burlington
El-Deeb WM, Younis EE (2009) Clinical and biochemical studies on Theileria annulata in Egyptian buffaloes (Bubalus bubalis) with particular orientation to oxidative stress and ketosis relationship. Vet Parasitol 164:301–305
El-Tarabany MS, El-Bayoumi KM (2015) Reproductive performance of backcross Holstein x Brown Swiss and their Holstein contemporaries under subtropical environmental conditions. Theriogenology 83:444–448
El-Tarabany MS, El-Tarabany AA (2015) Impact of maternal heat stress at insemination on the subsequent reproductive performance of Holstein, Brown Swiss, and their crosses. Theriogenology 84:1523–1529
El-Tarabany MS, Nasr MAF (2015) Reproductive performance of Brown Swiss, Holstein and their crosses under subtropical environmental conditions. Theriogenology 84:559–565
FAO (2013) FAOSTAT, faostat.fao.org/default.aspx
Finocchiaro R, Van Kaam JBCHM, Portolano B, Misztal I (2005) Effect of heat stress on production of Mediterranean dairy sheep. J Dairy Sci 88:1855–1864
Gajdusek S (1996) Impact of mastitis infection on production, quality, composition, and technological parameters of milk. In: Control of mastitis in milk production. Proceedings of the seminar. Rapotin, 05/16, p 27
Gaughan JB, Lacetera N, Valtorta SE, Khalifa HH, Hahn L, Mader T (2009) Response of domestic animals to climate challenges. Chapter 7. In: Ebi KL, Burton I, McGregor GR (eds) Biometeorology for adaptation to climate variability and change. Springer Verlag, Heidelberg, pp 131–170
Hamzaoui S, Salama AAK, Albanell E, Such X, Caja G (2013) Physiological responses and lactational performances of late-lactation dairy goats under heat stress conditions. J Dairy Sci 96:6355–6365
Hefnawy EA, Shousha S, Youssef S (2011) Hematobiochemical profile of pregnant and experimentally pregnancy toxemic goats. J Basic Appl Chem 1:65–69
Helal A, Hashem ALS, Abdel-Fattah MS, El-Shaer HM (2010) Effect of heat stress on coat characteristics and physiological responses of Balady and Damascus goats in Sinai, Egypt. Am Eurasian J Agric Environ Sci 7:60–69
Johnson HD (1987) Bioclimate and livestock. In: Johnson HD (ed) Bioclimatology and the adaptation of livestock. Elsevier Science Publisher, Amsterdam, pp 3–16
Joshi BC, McDowell RE, Sadhu DP (1968) Effect of drugs on sweating rates in Hariana cattle. J Dairy Sci 51:905–909
Kadzere CT, Murphy MR, Silanikove N, Maltz E (2002) Heat stress in lactating dairy cows: a review. Livest Prod Sci 77:59–91
Kandemir Ç, Koşum N, Taşkin T (2013) Effects of heat stress on physiological traits in sheep. Maced J Anim Sci 3:25–29
Kendall PE, Webster JR (2009) Season and physiological status affects the circadian body temperature rhythm of dairy cows. Livest Sci 125:155–160
Lough DS, Beede DK, Wilcox CJ (1990) Effects of feed intake and thermal stress on mammary blood flow and other physiological measurements in lactating dairy cows. J Dairy Sci 73:325–332
Marai IFM, Haeeb AAM (2010) Buffalo’s biological functions as affected by heat stress. A review. Livest Sci 127:89–109
Menegatos J, Goulas C, Kalogiannis D (2006) The productivity, ovarian and thyroid activity of ewes in an accelerated lambing system in Greece. Small Rumin Res 65:209–216
Menéndez-Buxadera A, Molina A, Arrebola F, Clemente I, Serradilla JM (2012) Genetic variation of adaptation to heat stress in two Spanish dairy goat breeds. J Anim Breed Genet 129:306–315
More T, Singh M, Rai AK (1980) Observation on excretory pattern of sodium, potassium and water at different temperature. Indian J Anim Sci 50:182–186
Nardone A, Ronchi B, Lacetera N, Bernabucci U (2006) Climate effects on productive traits in livestock. Vet Res Commun 30:75–81
Nardone A, Ronchi B, Lacetera N, Ranieri MS, Bernabucci U (2010) Effects of climate changes on animal production and sustainability of livestock systems. Livest Sci 130:57–69
NRC (2007) Nutrient requirements of small ruminants. Natl. Acad. of Sci, Washington
Ocak S, Guney O (2010) Physiological responses and some blood parameters of bucks under Mediterranean climate conditions. Anadolu J Agric Sci 25:113–119
Ocak S, Darcan N, Cankaya S, Inal TC (2009) Physiological and biochemical responses in German fawn kids subjected to cooling treatments under Mediterranean climate conditions. Turk J Vet Anim Sci 33:455–461
Phulia SK, Upadhyay RC, Jindal SK, Misra RP (2010) Alteration in surface body temperature and physiological responses in Sirohi goats during day time in summer season. Indian J Anim Sci 80:340–342
Pugh DG, Baird N (2012) Sheep and goat medicine. Elsevier Saunders, Maryland Heights
Rhoads ML, Rhoads RP, VanBaale MJ, Collier RJ, Sanders SR, Weber WJ, Crooker BA, Baumgard LH (2009) Effects of heat stress and plane of nutrition on lactating Holstein cows: I. Production, metabolism, and aspects of circulating somatotropin. J Dairy Sci 92:1986–1997
Robertshaw D (1982) Concepts in animal adaptation. Thermoregulation in the goat. Proc. III Int. Conf. Goat Production and Disease, Tucson. Dairy Goat Journal Publ. Co., Scottsdale, AZ
Salama AAK, Caja G, Hamzaoui S, Badaoui B, Castro-Costa A, Façanha DAE, Guilhermino MM, Bozzi R (2014) Different levels of response to heat stress in dairy goats. Small Rumin Res 121:73–79
Sano H, Ambo K, Tsuda T (1985) Blood glucose kinetics in whole body and mammary gland of lactating goats exposed to heat stress. J Dairy Sci 68:2557–2564
SAS (2003) SAS/STAT users guide. SAS Institute INC, Cary
Sevi A, Annicchiarico G, Albenzio M, Taibi L, Muscio A, Dell’Aquila S (2001) Effects of solar radiation and feeding time on behavior, immune response and production of lactating ewes under high ambient temperature. J Dairy Sci 84:629–640
Silanikove N (1997) Why goats raised on harsh environment perform better than other domesticated animals. Options Méditérr 34(Ser. A):185–194
Silanikove N (2000) Effects of heat stress on the welfare of extensively managed domestic ruminants. Livest Prod Sci 67:1–18
Silanikove N, Koluman N (2015) Impact of climate change on the dairy industry in temperate zones: predications on the overall negative impact and on the positive role of dairy goats in adaptation to earth warming. Small Rumin Res 123:27–34
Spiers DE, Spain JN, Sampson RP, Rhoads RP (2004) Use of physiological parameters to predict milk yield and feed intake in heatstressed dairy cows. J Therm Biol 29:759–764
Srikandakumar A, Johnson EH, Mahgoub O (2003) Effect of heat stress on respiratory rate, rectal temperature and blood chemistry in Omani and Australian Merino sheep. Small Rumin Res 49:193–198
Todini L (2007) Thyroid hormones in small ruminants: effects of endogenous, environmental and nutritional factors. Animal 1:997–1008
Uwayjan M, Bahattacharya AN (1975) Effect of high ambient temperature and low humidity on nutrient utilization and on some physiological responses in Awasi sheep fed different levels of roughage. J Anim Sci 40:320–328
Watson D (1960) A simple method for the determination of serum cholesterol. Clin Chem Acta 5:637–638
Webster AJF (1976) The influence of the climatic environment on metabolism on cattle. In: Broster WH, Swan H (eds) Principles of cattle production. Butterworths, London
Wheelock JB, Rhoads RP, VanBaale MJ, Sanders SR, Baumgard LH (2010) Effects of heat stress on energetic metabolism in lactating Holstein cows. J Dairy Sci 93:644–655
Wojtas K, Cwynar P, Kołacz R (2014) Effect of thermal stress on physiological and blood parameters in merino sheep. Bull Vet Inst Pulawy 58:283–288
Yousef MK (1985) Stress physiology in livestock: vol. 1. In: Basic principles. CRC Press, Boca Raton
Author information
Authors and Affiliations
Corresponding author
Ethics declarations
The current work was approved by the Committee of Animal Care and Welfare, Zagazig University, Egypt (ANWD-206). The experiment was carried out at the experimental farm of the Nuclear Research Center, Atomic Energy Authority, Inshas, Egypt.
Conflict of interest
The authors declare that they have no conflict of interest.
Rights and permissions
About this article
Cite this article
El-Tarabany, M.S., El-Tarabany, A.A. & Atta, M.A. Physiological and lactation responses of Egyptian dairy Baladi goats to natural thermal stress under subtropical environmental conditions. Int J Biometeorol 61, 61–68 (2017). https://doi.org/10.1007/s00484-016-1191-2
Received:
Revised:
Accepted:
Published:
Issue Date:
DOI: https://doi.org/10.1007/s00484-016-1191-2