Abstract
Acute kidney injury (AKI) is a common complication of neonatal encephalopathy (NE). The accurate diagnosis of neonatal AKI, irrespective of the cause, relies on suboptimal methods such as identification of rising serum creatinine, decreased urinary output and glomerular filtration rate. Studies of AKI biomarkers in adults and children have shown that biomarkers can improve the early diagnosis of AKI. Hypoxia–ischaemia is the proposed aetiological basis of AKI in both NE and cardiopulmonary bypass (CPB). However, there is a paucity of studies examining the role of AKI biomarkers specifically in NE. Urinary cystatin C (CysC), neutrophil gelatinase-associated lipocalin (NGAL), interleukin-18, kidney injury molecule-1, liver-type fatty acid-binding protein, serum CysC and serum NGAL all show good ability to predict early AKI in a heterogeneous critically ill neonatal population including infants post-CPB. Moreover, serum and urinary NGAL and urinary CysC are early predictors of AKI secondary to NE. These findings are promising and open up the possibility of biomarkers playing a significant role in the early diagnosis and treatment of NE-related AKI. There is an urgent need to explore the role of AKI biomarkers in infants with NE as establishing the diagnosis of AKI earlier may allow more timely intervention with potential for improving long-term outcome.
Similar content being viewed by others
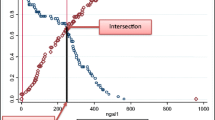
Avoid common mistakes on your manuscript.
Introduction
Acute kidney injury (AKI) is a common occurrence in infants with neonatal encephalopathy (NE), with a reported incidence of 50–72 % [1, 18, 23, 31, 40]. Most of these studies are small and employ varying definitions for AKI based on elevation of serum creatinine (SCr), oliguria, decreased glomerular filtration rate (GFR) and presence of haematuria and proteinuria. Nevertheless, these studies emphasise the frequency of AKI complicating NE and underline the uncertainty surrounding the precise and early diagnosis of AKI in critically ill neonates. All of the markers of AKI currently in use have disadvantages, probably the most important being the lag between the timing of renal injury and abnormalities in SCr and urinary output arising [5]. Significant renal damage has already occurred by the time the diagnosis of AKI has been made based on elevation of SCr and oliguria [57]. Studies have suggested that renal injury in NE is associated with more severe brain injury and adverse long-term neurodevelopmental outcome [55]; however, this correlation requires further study. It is therefore important that we develop techniques for early diagnosis of AKI.
Recently, there has been interest in developing biomarkers of AKI in adults and children, including urinary and serum neutrophil gelatinase-associated lipocalin (NGAL) and cystatin C (CysC), urinary interleukin-18 (IL-18), urinary kidney injury molecule-1 (KIM-1), urinary liver-type fatty acid-binding protein (L-FABP) and others. Paediatric studies indicate that AKI can be diagnosed earlier using serum and urinary AKI biomarkers [6, 19, 22, 33, 34, 37, 42, 54, 56, 57, 65, 68, 69]. Additionally, there has been a number of studies examining the role of AKI biomarkers in critically ill newborns [6, 33, 34, 37, 57]. In this review, we discuss the relevance of these biomarkers of AKI during NE and outline the important role these biomarkers may play in the clinical management of critically ill newborns.
There is considerable confusion in the literature regarding the correct terminology of infants with NE. As per consensus [10, 36, 50], we have used the term neonatal encephalopathy rather than hypoxic–ischaemic encephalopathy (HIE) except where authors have specifically defined their study group in terms of meeting criteria for perinatal asphyxia.
Limitations of serum creatinine
Elevated SCr (> 132.6 μmol/L) is a commonly used marker of AKI in neonates despite its numerous inherent flaws [5]. Firstly, SCr may not increase until about 25–50 % of renal function is lost [5] as the relationship between GFR and SCr relies on a steady state where the endogenous generation of creatinine from muscle is in equilibrium with renal clearance. In the first 48 h of life, neonatal SCr reflects maternal but not neonatal renal function. Neonatal GFR also steadily increases during the first few months of life [5, 13, 17], which may influence the interpretation of early changes in SCr. Additionally, the method of SCr quantification utilised can affect the result as the older Jaffe assay is influenced by medications and hyperbilirubinaemia [27]. Lastly, absolute cutoff values of rising SCr (e.g. > 132.6 μmol/L [1]) are employed to identify the presence or absence of AKI, which leaves no capacity for determining the precise degree of acute kidney injury sustained.
Limitations of AKI biomarker studies
Although AKI biomarkers are opening up an exciting new era in AKI diagnosis, it must be emphasised that all the studies of AKI biomarkers to date are limited by the use of a suboptimal gold-standard definition of AKI, i.e. elevated SCr [4]. The challenge of defining neonatal AKI accurately using novel biomarkers is significantly hampered by the reliance on historical gold-standard (but suboptimal) definitions which, as discussed previously, have many disadvantages. Furthermore, validation of AKI biomarkers is impaired by the lack of a high-quality, sensitive and specific definition of AKI.
In order to develop novel definitions of neonatal AKI, normative values for AKI biomarkers in healthy term neonates and critically ill term newborns need to be established and compared to pre-defined and internationally recognised criteria for AKI. Such a definition of neonatal AKI needs to be categorical, including a combination of rising SCr and decreasing urinary output and avoiding the use of estimated creatinine clearance which is not a reliable indicator of a newborn’s true creatinine clearance [16]. Once biomarker cutoff values for the identification of AKI are established, they can be further validated and incorporated into a novel categorical definition of neonatal AKI for use in clinical practice. It is important that studies of long-term outcome following neonatal AKI would also incorporate these novel categorical definitions of AKI so that the predictive ability of AKI biomarkers can be estimated and possible impact on long-term outcomes can be assessed.
Effect of gestational age on AKI biomarkers
The GFR of a fetus increases steadily in parallel with gestational age over the course of pregnancy due to an increase in the number and size of nephrons [26]. Therefore, gestational age may theoretically affect biomarkers of acute kidney injury. Serum CysC values of premature infants appear to be independent of gestational age [3, 11, 15, 24, 46]. In contrast, urinary CysC in non-AKI control infants is significantly negatively associated with gestational age [37]. Urinary NGAL is significantly associated with gestational age in pre-term neonates, with concentrations of urinary NGAL declining significantly as gestational age increases [7, 28, 35]. Urinary IL-18 and urinary KIM-1 levels however do not appear to vary significantly across gestational age categories [7, 37]. Levels of urinary L-FABP in pre-term infants have been shown to be significantly negatively correlated with gestational age only on day 1 of life [61]. It is important that variations in AKI biomarkers associated with gestational age are incorporated into any future proposed definition of neonatal AKI.
Serum cystatin C
Cystatin C is a cysteine protease inhibitor that is synthesised and released into the blood at a relatively constant rate by all nucleated cells. It is freely filtered by the glomerulus, completely reabsorbed by the proximal tubule and not secreted [67] (Fig. 1). In newborn infants, serum cystatin C (sCysC) levels are independent of gender, height, gestational age, lean body mass and serum bilirubin level [8], all of which cause problems when SCr is used to estimate GFR in neonates. SCr is an inferior marker of kidney function in the first days of life of an infant compared to sCysC. Neonatal SCr levels in the first 24–48 h of life may not reflect the true neonatal renal function as the maternal kidneys clear any fetal creatinine that may cross the placenta [51]. In addition, fetal sCysC levels do not show any relationship to maternal sCysC levels [9]. Therefore, sCysC appears to avoid many of the problems associated with SCr as an estimate of renal function in neonates and may even be a superior marker of GFR in this particular population.
Serum CysC is undergoing investigation in the adult population as a potential early biomarker of AKI [25, 60]. Both NGAL and sCysC levels at 12 h are strong independent predictors of AKI post-paediatric cardiac surgery; however, NGAL outperforms sCysC at earlier time points [63]. In a larger, more recent paediatric study of AKI post-cardiopulmonary bypass (CPB) [33], sCysC concentrations were significantly increased in AKI patients at 12 h post-CPB and remained elevated at 24 h. The 12-h sCysC strongly correlated with severity and duration of AKI as well as with hospital stay. A systematic review and meta-analysis [69] found that across all settings including paediatrics, sCysC demonstrated excellent ability to predict AKI with best diagnostic value when performed early.
Sarafidis et al. [57] carried out a small study on AKI biomarkers in infants with perinatal asphyxia. Although other studies have looked at AKI biomarkers in critically ill neonates, this is the first study that has looked specifically at the predictive value of AKI biomarkers (namely, CysC, NGAL and KIM-1) in diagnosing AKI in asphyxiated term neonates. Acute perinatal asphyxia was defined as: (1) the presence of a sentinel hypoxic event immediately before or during delivery, (2) history of fetal distress (bradycardia, late decelerations, absence of heart rate variability), (3) need for advanced neonatal resuscitation at delivery, (4) 5-min Apgar score ≤ 6, (5) metabolic acidosis (base deficit ≥ 12 mmol/L) within the first hour of life and (6) clinical indications of HIE and multiple organ involvement. Of note, none of the 13 asphyxiated infants enrolled underwent therapeutic hypothermia. Serum CysC levels were significantly higher in asphyxiated infants compared to control infants on day of life (DOL) 1, while urinary cystatin C (uCysC) was significantly higher in asphyxiated infants at all time points (DOL 1, 3 and 10). However, sCysC levels did not differ between the group of asphyxiated infants with AKI compared to the asphyxiated group without AKI. The study group defined AKI as SCr ≥ 132.6 μmol/L for > 24 h or rising SCr values > 26.52 μmol/L from DOL 1. Urinary CysC was also significantly higher in asphyxiated neonates with AKI compared to controls at all time points and in the asphyxiated group without AKI compared to controls on DOL 1 and 3. Serum CysC and uCysC showed favourable diagnostic performances as predictors of AKI on DOL 1.
Two other studies [6, 37] examined the role of AKI biomarkers in critically ill newborns (term and pre-term), although infants with congenital anomalies or sepsis were excluded. Li et al. included eight term infants with NE. Urinary CysC was predictive of AKI even after controlling for gestational age, post-natal age, birth weight, gender, Apgar score and score for neonatal acute physiology (SNAP) in non-septic critically ill neonates [37]. Askenazi et al. recruited 58 infants born > 34 weeks with birth weight > 2,000 g and 5-min Apgar score ≤ 7 and AKI developed in nine infants. The number of infants who developed NE in this cohort is not specified, but five of nine were intubated in the delivery room, six of nine were ventilated and one of nine received phenobarbital. Infants with AKI had higher levels of uCysC compared to infants without AKI [6].
Caution is warranted however when evaluating CysC as a potential early AKI biomarker as it is not a direct marker of renal tubular damage but simply an alternative marker (to SCr) of GFR [2]. Further studies are vital to elucidate the role of CysC in the prediction of AKI in NE (Table 1).
Neutrophil gelatinase-associated lipocalin
NGAL is a 25-kDa lipocalin secreted by activated neutrophils and expressed in many cells (e.g. kidney, lungs, stomach and colon). NGAL is upregulated in several injury settings, including infection, cancer and renal tubular injury [42–44, 47, 58, 64], via induction of NGAL mRNA (Fig. 1). In the setting of renal epithelial injury, human serum NGAL (sNGAL) levels are increased by seven- to 16-fold [58] and urinary NGAL (uNGAL) levels increased by 25–100-fold [58]. NGAL was recently identified by microarray analysis using genomic and proteomic technologies as one of the earliest and most robustly induced genes and proteins in the kidney after ischaemic or nephrotoxic injury in animal models. NGAL protein was easily detected in the blood and urine soon after AKI [44, 45, 47, 58, 59].
In many studies on children (for example, [12, 42, 48, 68]) and adults (for example, [64]), NGAL rises significantly in patients with AKI but not in controls. Furthermore, this rise in NGAL occurs at 24 to 48 h before the rise in SCr is observed. NGAL both in urine and plasma is an excellent early marker of AKI in children undergoing CPB, showing good diagnostic performance with receiver operating characteristic curve analysis at 2 h post-operatively [42]. A recent meta-analysis (including both paediatric and adult studies) [19] revealed an overall area under the receiver operating characteristic (AUROC) curve of NGAL of 0.815 for prediction of AKI with better predictive ability in children compared to adults.
Zappitelli et al. [68] prospectively assessed the ability of uNGAL to predict AKI development and severity of AKI in a heterogeneous critically ill paediatric population (1 month to 21 years) admitted to PICU who received mechanical ventilation and underwent indwelling bladder catheterisation. Patients with end-stage renal disease and who had undergone renal transplantation were excluded. Mean and peak uNGAL concentrations increased with worsening paediatric Risk Injury Failure Loss End-stage renal failure (pRIFLE) maximal scores (p < 0.05). In AKI, uNGAL concentrations rose by sixfold versus controls at 2 days before a ≥ 50 % rise in SCr, without change in control uNGAL. However, uNGAL did not predict AKI severity after a rise in SCr had already occurred. Still taking into account that the timing of the renal insult was unknown and the population was heterogeneous, uNGAL performed reasonably well with regard to prediction of AKI occurrence and severity before AKI development.
The temporal relationship of urinary AKI biomarkers following paediatric CPB was established by Krawczeski et al., who described uNGAL rising first at 2 h post-CPB, followed by IL-18 and L-FABP at 6 h and finally KIM-1 at 12 h. Significant correlations were found between elevated urinary AKI biomarkers and severity of AKI and clinical outcomes. Krawczeski et al. reinforces the theory that a panel of biomarkers will most accurately predict AKI compared to a single biomarker. A clinical model to predict AKI alone showed an AUROC = 0.74, while with the addition of uNGAL at 2 h this increased to 0.85. At 6 h, the addition of NGAL, IL-18 and L-FABP each improved the AUROC from 0.72 to 0.91, 0.84 and 0.77, respectively.
There have been few studies looking at uNGAL specifically in term neonates. Krawczeski et al. [34] included 35 neonates (age <30 days) in the 374 children enrolled in their study of urinary and sNGAL concentrations after CPB. Eight newborns developed AKI, and uNGAL and sNGAL levels increased significantly in neonates with AKI at 2 h after CPB and remained elevated at all time points. No variable, except urinary and sNGAL thresholds, was predictive of AKI. AUROC curves for uNGAL and sNGAL indicated that both urinary and sNGAL are outstanding predictors of AKI in neonates at all time points following CPB. This population of neonates was a relatively homogeneous cohort, where the precise timing of injury was known. The ability of NGAL to predict neonatal AKI does not appear to be limited to such a homogeneous group however.
Sarafidis et al. explored the role of NGAL in the context of post-asphyxial AKI [57]. Serum and uNGAL levels were significantly higher in asphyxiated neonates compared to controls on DOL 1, 3 and 10. The levels of sNGAL and uNGAL were significantly elevated compared to controls irrespective of whether the asphyxiated infant had AKI or not based on elevation of SCr, which hints at the spectrum of AKI that is being missed when diagnosis is based solely on rising SCr . Within the subgroup of asphyxiated infants, sNGAL was significantly elevated in the “asphyxia with AKI group” compared to the “asphyxia without AKI group” (defined by SCr) on DOL 1 and 3 (p = 0.016 and 0.001, respectively). This underlines the fact that defining AKI by SCr alone is inadequate and that there is a spectrum of acute tubular injury occuring post-asphyxial injury, only the more severe of which qualifies as AKI when defined by SCr. Serum and uNGAL showed good diagnostic peformance as predictors of AKI on DOL 1 (Table 2).
Urinary interleukin-18
Urinary interleukin-18 is also being promoted as an early non-invasive biomarker of AKI [41]. Interleukin-18 is an 18-kDa pro-inflammatory cytokine initially synthesised in its inactive form (24-kDa) and subsequently cleaved by caspase-1 in the epithelial cells of the proximal tubules into its active form (Fig. 1). It is easily detected in the urine following ischaemic AKI in mice models [41].
IL-18 urine levels are elevated in adults with ischaemic AKI compared with other causes of renal injury, including urinary tract infection, nephrotic syndrome and chronic renal failure [53], and levels appear to rise significantly earlier than SCr in adults with acute respiratory distress syndrome who develop AKI [52]. However, there have been contradictory reports on the ability of IL-18 to predict AKI in adults following CPB [20].
Urinary NGAL and urinary IL-18 (uIL-18) were recently shown to represent early, predictive, sequential AKI biomarkers in children undergoing cardiac surgery [54]. In post-operative AKI, uNGAL was induced within 2 h (25-fold increase) and peaked at 6 h. Urinary IL-18 levels increased at around 6 h, peaked at over 25-fold at 12 h and remained elevated up to 48 h after CPB. Urinary IL-18 performed best as a predictor of AKI at 12 h compared to other time points. Both uNGAL and uIL-18 were independently associated with duration of AKI [54]. The combination of these two biomarkers may facilitate the early diagnosis of AKI as compared to SCr which, as a marker of renal function, does not expose the real-time tubular injury that is occuring.
Washburn et al. [65] determined that peak uIL-18 levels increased with worsening AKI severity (measured by pRIFLE score) in critically ill children (non-cardiac, PICU patients aged 1 month to 21 years), i.e. more heterogeneous group. However, uIL-18 did not perform effectively as an early predictor of AKI. There was a particular peak in uIL-18 in those with sepsis. Elevated uIL-18 levels were found to be an independent predictor of mortality. The better predictive ability of uIL-18 for the diagnosis of AKI in children compared to adults may be due to the release of IL-18 primarily through the kidney in children rather than the immune cells in adults [20].
A group of 62 critically ill neonates including eight term neonates with NE were recently evaluated for urinary biomarkers of AKI by Li et al. Urinary IL-18 was significantly higher in non-septic critically ill neonates with AKI compared to controls even when adjusted for gestational age, post-natal age, birth weight, 5-min Apgar score, gender and SNAP score. IL-18 demonstrated reasonably good ability to predict AKI within 48 h (Table 1). The authors did not divide the patients into clinical groups; therefore, it is not possible to extrapolate these findings to infants with NE (Table 3).
Kidney injury molecule-1
Kidney injury molecule-1 is being studied as a potential early biomarker of AKI in adults [38, 39]. KIM-1 is a 104-kDa type-1 trans-membrane glycoprotein that is highly overexpressed in dedifferentiated proximal tubule cells (Fig. 1) after ischaemic or nephrotoxic AKI in animal models [21, 29], and a proteolytically processed domain is easily detected in the urine [62]. In a small human cross-sectional study, KIM-1 was found to be markedly induced in proximal tubules in kidney biopsies from patients with established AKI (primarily ischaemic), and urinary KIM-1 (uKIM-1) distinguished ischaemic AKI from pre-renal azotaemia and chronic renal disease [21].
A case–control study of 40 children undergoing cardiac surgery found increased uKIM-1 levels in the 20 patients with AKI [22]. Pre-operative investigation of the urinary biomarker profile of children with congenital hydronephrosis found that median uKIM-1/SCr levels were significantly greater in children with severe congenital hydronephrosis compared to controls [66].
In contrast to the aforementioned paediatric studies, uKIM-1 levels were comparable between asphyxiated neonates (n = 13) and healthy controls (n = 22) on DOL 1, 3 and 10 and did not discriminate the AKI group from the non-AKI group within the subgroup of asphyxiated neonates [57] (Table 4).
Liver-type fatty acid-binding protein
Proximal tubule cells express the 14-kDa protein, liver-type fatty acid-binding protein (L-FABP), which binds, helps transport and facilitates metabolism of urinary filtered free fatty acids (Fig. 1). In mouse models exposed to nephrotoxic cisplatin and to renal ischaemia, L-FABP increased within minutes to hours of AKI exposure and correlated with the severity of injury on histological examination [56]. Serum L-FABP levels do not have an influence on urinary levels, and urinary L-FABP (uL-FABP) levels in patients with liver disease are not significantly higher than those in healthy subjects [30, 49]. The role of uL-FABP in AKI in children was explored by Portilla et al. [56]. Urinary L-FABP levels rose significantly at 4 and 12 h in both patients with and without AKI following congenital heart surgery (n = 40), but this rise was significantly higher in children who subsequently developed AKI. Urinary L-FABP levels rose at least 1 day prior to SCr levels and displayed good predictive ability for the development of subsequent AKI. Urinary L-FABP also correlated with a longer length of hospital stay. In adult patients, uL-FABP compared favourably to uNGAL and uKIM-1 in the prediction of AKI and was statistically better than uIL-18 [14]. To date, there are no published studies examining the role of uL-FABP in neonatal AKI (Table 5).
Conclusion
Although AKI is common in infants with NE, current practice relies on suboptimal definitions and biomarkers. Adult studies suggest that urinary and sNGAL and uL-FABP have potential roles in a panel of biomarkers to predict AKI at earlier time points. uKIM-1 and uIL-18 show promise at later time points and sCysC is a validated and accurate alternative marker of GFR to SCr. There is a dearth of studies examining the role of AKI biomarkers in NE. Therefore, in this review, we included articles on biomarkers of AKI in neonates in general to explore the possibility of, in the future, expanding the remit of AKI biomarkers to detect perinatal hypoxic–ischaemic renal injury. There is evidence that measurement of AKI biomarkers such as CysC and NGAL on DOL 1 following acute neonatal asphyxia has a significant predictive value for the identification of AKI. A panel of AKI biomarkers that would differentiate the type of renal injury that has occurred, i.e. glomerular or tubular injury, and that would also have the ability to predict AKI and outcome would be extremely useful clinically in the acute period and also may help in the long-term follow-up of these infants.
There are also many uncertainties regarding fluid and electrolyte balance and cerebral oedema in infants with NE. In theory, it is possible that AKI combined with tubular dysfunction worsens cerebral oedema. More precise quantification of AKI using biomarkers may, in the future, be used to tailor fluid management so that cerebral oedema is minimised without compromising renal function. There is consequently an urgent need to look more closely at the role of novel AKI biomarkers in the diagnosis of renal injury in term infants with NE.
References
Aggarwal A, Kumar P, Chowdhary G, Majumdar S, Narang A (2005) Evaluation of renal functions in asphyxiated newborns. J Trop Pediatr 51(5):295–299
Al-Ismaili Z, Palijan A, Zappitelli M (2011) Biomarkers of acute kidney injury in children: discovery, evaluation, and clinical application. Pediatr Nephrol 26(1):29–40
Armangil D, Yurdakok M, Canpolat FE, Korkmaz A, Yigit S, Tekinalp G (2008) Determination of reference values for plasma cystatin C and comparison with creatinine in premature infants. Pediatr Nephrol 23(11):2081–2083
Askenazi D (2012) Are we ready for the clinical use of novel acute kidney injury biomarkers? Pediatr Nephrol 27(9):1423–1425
Askenazi DJ, Ambalavanan N, Goldstein SL (2009) Acute kidney injury in critically ill newborns: what do we know? What do we need to learn? Pediatr Nephrol 24(2):265–274
Askenazi DJ, Koralkar R, Hundley HE, Montesanti A, Parwar P, Sonjara S, Ambalavanan N (2012) Urine biomarkers predict acute kidney injury in newborns. J Pediatr 161(2):270–5
Askenazi DJ, Koralkar R, Levitan EB, Goldstein SL, Devarajan P, Khandrika S, Mehta RL, Ambalavanan N (2011) Baseline values of candidate urine acute kidney injury biomarkers vary by gestational age in premature infants. Pediatr Res 70(3):302–306
Bahar A, Yilmaz Y, Unver S, Gocmen I, Karademir F (2003) Reference values of umbilical cord and third-day cystatin C levels for determining glomerular filtration rates in newborns. J Int Med Res 31(3):231–235
Cataldi L, Mussap M, Bertelli L, Ruzzante N, Fanos V, Plebani M (1999) Cystatin C in healthy women at term pregnancy and in their infant newborns: relationship between maternal and neonatal serum levels and reference values. Am J Perinatol 16(6):287–295
Dammann O, Ferriero D, Gressens P (2011) Neonatal encephalopathy or hypoxic–ischemic encephalopathy? Appropriate terminology matters. Pediatr Res 70(1):1–2
Demirel G, Celik IH, Canpolat FE, Erdeve O, Biyikli Z, Dilmen U (2012) Reference values of serum cystatin C in very low birth weight premature infants. Acta Paediatr. Sept 27 [Epub ahead of print]
Devarajan P (2007) Emerging biomarkers of acute kidney injury. Contrib Nephrol 156:203–212
Durkan AM, Alexander RT (2011) Acute kidney injury post neonatal asphyxia. J Pediatr 158(2 Suppl):e29–33
Ferguson MA, Vaidya VS, Waikar SS, Collings FB, Sunderland KE, Gioules CJ, Bonventre JV (2010) Urinary liver-type fatty acid-binding protein predicts adverse outcomes in acute kidney injury. Kidney Int 77(8):708–714
Finney H, Newman DJ, Thakkar H, Fell JM, Price CP (2000) Reference ranges for plasma cystatin C and creatinine measurements in premature infants, neonates, and older children. Arch Dis Child 82(1):71–75
Gadepalli SK, Selewski DT, Drongowski RA, Mychaliska GB (2011) Acute kidney injury in congenital diaphragmatic hernia requiring extracorporeal life support: an insidious problem. J Pediatr Surg 46(4):630–635
Gouyon JB, Guignard JP (2000) Management of acute renal failure in newborns. Pediatr Nephrol 14(10–11):1037–1044
Gupta BD, Sharma P, Bagla J, Parakh M, Soni JP (2005) Renal failure in asphyxiated neonates. Indian Pediatr 42(9):928–934
Haase M, Bellomo R, Devarajan P, Schlattmann P, Haase-Fielitz A (2009) Accuracy of neutrophil gelatinase-associated lipocalin (NGAL) in diagnosis and prognosis in acute kidney injury: a systematic review and meta-analysis. Am J Kidney Dis 54(6):1012–1024
Haase M, Bellomo R, Story D, Davenport P, Haase-Fielitz A (2008) Urinary interleukin-18 does not predict acute kidney injury after adult cardiac surgery: a prospective observational cohort study. Crit Care 12(4):R96
Han WK, Bailly V, Abichandani R, Thadhani R, Bonventre JV (2002) Kidney injury molecule-1 (KIM-1): a novel biomarker for human renal proximal tubule injury. Kidney Int 62(1):237–244
Han WK, Waikar SS, Johnson A, Betensky RA, Dent CL, Devarajan P, Bonventre JV (2008) Urinary biomarkers in the early diagnosis of acute kidney injury. Kidney Int 73(7):863–869
Hankins GD, Koen S, Gei AF, Lopez SM, Van Hook JW, Anderson GD (2002) Neonatal organ system injury in acute birth asphyxia sufficient to result in neonatal encephalopathy. Obstet Gynecol 99(5 Pt 1):688–691
Harmoinen A, Ylinen E, Ala-Houhala M, Janas M, Kaila M, Kouri T (2000) Reference intervals for cystatin C in pre- and full-term infants and children. Pediatr Nephrol 15(1–2):105–108
Herget-Rosenthal S, Marggraf G, Husing J, Goring F, Pietruck F, Janssen O, Philipp T, Kribben A (2004) Early detection of acute renal failure by serum cystatin C. Kidney Int 66(3):1115–1122
Hoseini R, Otukesh H, Rahimzadeh N, Hoseini S (2012) Glomerular function in neonates. Iran J Kidney Dis 6(3):166–172
Hsu CW, Symons JM (2010) Acute kidney injury: can we improve prognosis? Pediatr Nephrol 25(12):2401–2412
Huynh TK, Bateman DA, Parravicini E, Lorenz JM, Nemerofsky SL, Sise ME, Bowman TM, Polesana E, Barasch JM (2009) Reference values of urinary neutrophil gelatinase-associated lipocalin in very low birth weight infants. Pediatr Res 66(5):528–532
Ichimura T, Hung CC, Yang SA, Stevens JL, Bonventre JV (2004) Kidney injury molecule-1: a tissue and urinary biomarker for nephrotoxicant-induced renal injury. Am J Physiol Renal Physiol 286(3):F552–563
Kamijo A, Sugaya T, Hikawa A, Yamanouchi M, Hirata Y, Ishimitsu T, Numabe A, Takagi M, Hayakawa H, Tabei F, Sugimoto T, Mise N, Omata M, Kimura K (2006) Urinary liver-type fatty acid binding protein as a useful biomarker in chronic kidney disease. Mol Cell Biochem 284(1–2):175–182
Karlowicz MG, Adelman RD (1995) Nonoliguric and oliguric acute renal failure in asphyxiated term neonates. Pediatr Nephrol 9(6):718–722
Krawczeski CD, Goldstein SL, Woo JG, Wang Y, Piyaphanee N, Ma Q, Bennett M, Devarajan P (2011) Temporal relationship and predictive value of urinary acute kidney injury biomarkers after pediatric cardiopulmonary bypass. J Am Coll Cardiol 58(22):2301–2309
Krawczeski CD, Vandevoorde RG, Kathman T, Bennett MR, Woo JG, Wang Y, Griffiths RE, Devarajan P (2010) Serum cystatin C is an early predictive biomarker of acute kidney injury after pediatric cardiopulmonary bypass. Clin J Am Soc Nephrol 5(9):1552–1557
Krawczeski CD, Woo JG, Wang Y, Bennett MR, Ma Q, Devarajan P (2011) Neutrophil gelatinase-associated lipocalin concentrations predict development of acute kidney injury in neonates and children after cardiopulmonary bypass. J Pediatr 158(6):1009–1015 e1001
Lavery AP, Meinzen-Derr JK, Anderson E, Ma Q, Bennett MR, Devarajan P, Schibler KR (2008) Urinary NGAL in premature infants. Pediatr Res 64(4):423–428
Leviton A, Nelson KB (1992) Problems with definitions and classifications of newborn encephalopathy. Pediatr Neurol 8(2):85–90
Li Y, Fu C, Zhou X, Xiao Z, Zhu X, Jin M, Li X, Feng X (2012) Urine interleukin-18 and cystatin-C as biomarkers of acute kidney injury in critically ill neonates. Pediatr Nephrol 27(5):851–860
Liang XL, Liu SX, Chen YH, Yan LJ, Li H, Xuan HJ, Liang YZ, Shi W (2010) Combination of urinary kidney injury molecule-1 and interleukin-18 as early biomarker for the diagnosis and progressive assessment of acute kidney injury following cardiopulmonary bypass surgery: a prospective nested case–control study. Biomarkers 15(4):332–339
Liangos O, Perianayagam MC, Vaidya VS, Han WK, Wald R, Tighiouart H, MacKinnon RW, Li L, Balakrishnan VS, Pereira BJ, Bonventre JV, Jaber BL (2007) Urinary N-acetyl-beta-(d)-glucosaminidase activity and kidney injury molecule-1 level are associated with adverse outcomes in acute renal failure. J Am Soc Nephrol 18(3):904–912
Martin-Ancel A, Garcia-Alix A, Gaya F, Cabanas F, Burgueros M, Quero J (1995) Multiple organ involvement in perinatal asphyxia. J Pediatr 127(5):786–793
Melnikov VY, Ecder T, Fantuzzi G, Siegmund B, Lucia MS, Dinarello CA, Schrier RW, Edelstein CL (2001) Impaired IL-18 processing protects caspase-1-deficient mice from ischemic acute renal failure. J Clin Invest 107(9):1145–1152
Mishra J, Dent C, Tarabishi R, Mitsnefes MM, Ma Q, Kelly C, Ruff SM, Zahedi K, Shao M, Bean J, Mori K, Barasch J, Devarajan P (2005) Neutrophil gelatinase-associated lipocalin (NGAL) as a biomarker for acute renal injury after cardiac surgery. Lancet 365(9466):1231–1238
Mishra J, Ma Q, Kelly C, Mitsnefes M, Mori K, Barasch J, Devarajan P (2006) Kidney NGAL is a novel early marker of acute injury following transplantation. Pediatr Nephrol 21(6):856–863
Mishra J, Ma Q, Prada A, Mitsnefes M, Zahedi K, Yang J, Barasch J, Devarajan P (2003) Identification of neutrophil gelatinase-associated lipocalin as a novel early urinary biomarker for ischemic renal injury. J Am Soc Nephrol 14(10):2534–2543
Mishra J, Mori K, Ma Q, Kelly C, Barasch J, Devarajan P (2004) Neutrophil gelatinase-associated lipocalin: a novel early urinary biomarker for cisplatin nephrotoxicity. Am J Nephrol 24(3):307–315
Montini G, Cosmo L, Amici G, Mussap M, Zacchello G (2001) Plasma cystatin C values and inulin clearances in premature neonates. Pediatr Nephrol 16(5):463–465
Mori K, Lee HT, Rapoport D, Drexler IR, Foster K, Yang J, Schmidt-Ott KM, Chen X, Li JY, Weiss S, Mishra J, Cheema FH, Markowitz G, Suganami T, Sawai K, Mukoyama M, Kunis C, D'Agati V, Devarajan P, Barasch J (2005) Endocytic delivery of lipocalin–siderophore–iron complex rescues the kidney from ischemia–reperfusion injury. J Clin Invest 115(3):610–621
Mussap M, Degrandi R, Fravega M, Fanos V (2010) Acute kidney injury in critically ill infants: the role of urine neutrophil gelatinase-associated lipocalin (NGAL). J Matern Fetal Neonatal Med 23(Suppl 3):70–72
Nakamura T, Sugaya T, Koide H (2009) Urinary liver-type fatty acid-binding protein in septic shock: effect of polymyxin B-immobilized fiber hemoperfusion. Shock 31(5):454–459
Nelson KB (2007) Is it HIE? And why that matters. Acta Paediatr 96(8):1113–1114
Nolte S, Mueller B, Pringsheim W (1991) Serum alpha 1-microglobulin and beta 2-microglobulin for the estimation of fetal glomerular renal function. Pediatr Nephrol 5(5):573–577
Parikh CR, Abraham E, Ancukiewicz M, Edelstein CL (2005) Urine IL-18 is an early diagnostic marker for acute kidney injury and predicts mortality in the intensive care unit. J Am Soc Nephrol 16(10):3046–3052
Parikh CR, Jani A, Melnikov VY, Faubel S, Edelstein CL (2004) Urinary interleukin-18 is a marker of human acute tubular necrosis. Am J Kidney Dis 43(3):405–414
Parikh CR, Mishra J, Thiessen-Philbrook H, Dursun B, Ma Q, Kelly C, Dent C, Devarajan P, Edelstein CL (2006) Urinary IL-18 is an early predictive biomarker of acute kidney injury after cardiac surgery. Kidney Int 70(1):199–203
Perlman JM, Tack ED (1988) Renal injury in the asphyxiated newborn infant: relationship to neurologic outcome. J Pediatr 113(5):875–879
Portilla D, Dent C, Sugaya T, Nagothu KK, Kundi I, Moore P, Noiri E, Devarajan P (2008) Liver fatty acid-binding protein as a biomarker of acute kidney injury after cardiac surgery. Kidney Int 73(4):465–472
Sarafidis K, Tsepkentzi E, Agakidou E, Diamanti E, Taparkou A, Soubasi V, Papachristou F, Drossou V (2012) Serum and urine acute kidney injury biomarkers in asphyxiated neonates. Pediatr Nephrol 27(9):1575–82
Schmidt-Ott KM, Mori K, Kalandadze A, Li JY, Paragas N, Nicholas T, Devarajan P, Barasch J (2006) Neutrophil gelatinase-associated lipocalin-mediated iron traffic in kidney epithelia. Curr Opin Nephrol Hypertens 15(4):442–449
Supavekin S, Zhang W, Kucherlapati R, Kaskel FJ, Moore LC, Devarajan P (2003) Differential gene expression following early renal ischemia/reperfusion. Kidney Int 63(5):1714–1724
Trof RJ, Di Maggio F, Leemreis J, Groeneveld AB (2006) Biomarkers of acute renal injury and renal failure. Shock 26(3):245–253
Tsukahara H, Sugaya T, Hayakawa K, Mori Y, Hiraoka M, Hata A, Mayumi M (2005) Quantification of L-type fatty acid binding protein in the urine of preterm neonates. Early Hum Dev 81(7):643–646
Vaidya VS, Ramirez V, Ichimura T, Bobadilla NA, Bonventre JV (2006) Urinary kidney injury molecule-1: a sensitive quantitative biomarker for early detection of kidney tubular injury. Am J Physiol Renal Physiol 290(2):F517–529
VandeVoorde RG et al (2006) Serum NGAL and cystatin C as predictive biomarkers for acute kidney injury. J Am Soc Nephrol 17:404A
Wagener G, Jan M, Kim M, Mori K, Barasch JM, Sladen RN, Lee HT (2006) Association between increases in urinary neutrophil gelatinase-associated lipocalin and acute renal dysfunction after adult cardiac surgery. Anesthesiology 105(3):485–491
Washburn KK, Zappitelli M, Arikan AA, Loftis L, Yalavarthy R, Parikh CR, Edelstein CL, Goldstein SL (2008) Urinary interleukin-18 is an acute kidney injury biomarker in critically ill children. Nephrol Dial Transplant 23(2):566–572
Wasilewska A, Taranta-Janusz K, Debek W, Zoch-Zwierz W, Kuroczycka-Saniutycz E (2011) KIM-1 and NGAL: new markers of obstructive nephropathy. Pediatr Nephrol 26(4):579–586
Zaffanello M, Franchini M, Fanos V (2007) Is serum cystatin-C a suitable marker of renal function in children? Ann Clin Lab Sci 37(3):233–240
Zappitelli M, Washburn KK, Arikan AA, Loftis L, Ma Q, Devarajan P, Parikh CR, Goldstein SL (2007) Urine neutrophil gelatinase-associated lipocalin is an early marker of acute kidney injury in critically ill children: a prospective cohort study. Crit Care 11(4):R84
Zhang Z, Lu B, Sheng X, Jin N (2011) Cystatin C in prediction of acute kidney injury: a systemic review and meta-analysis. Am J Kidney Dis 58(3):356–365
Conflict of interest
The authors declare that they have no conflict of interest.
Author information
Authors and Affiliations
Corresponding author
Rights and permissions
About this article
Cite this article
Sweetman, D., Molloy, E. Biomarkers of acute kidney injury in neonatal encephalopathy. Eur J Pediatr 172, 305–316 (2013). https://doi.org/10.1007/s00431-012-1890-6
Received:
Accepted:
Published:
Issue Date:
DOI: https://doi.org/10.1007/s00431-012-1890-6