Abstract
The main aim of this review is to discuss some evidence-based physiological and methodological considerations for optimal use of neuromuscular electrical stimulation (NMES) in healthy and impaired skeletal muscles. After a quick overview of the main applications, interests and limits of NMES use, the first section concentrates on two crucial aspects of NMES physiology: the differences in motor unit recruitment pattern between NMES and voluntary contractions, and the involvement of the nervous system during peripheral NMES. The second section of the article focuses on the most common NMES parameters, which entail the characteristics of both the electrical current (the input) and the evoked contraction (the output).
Similar content being viewed by others
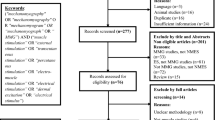
Avoid common mistakes on your manuscript.
Introduction
Definition of NMES
Neuromuscular electrical stimulation (NMES) involves the application of a series of intermittent stimuli to superficial skeletal muscles, with the main objective to trigger visible muscle contractions due to the activation of the intramuscular nerve branches (Hultman et al. 1983). Electrical stimuli are generally delivered using one or more active electrodes positioned in proximity to the muscle motor points (Fig. 1), and pre-programmed stimulation units. An intact motor nerve is a prerequisite for eliciting muscle contractions with NMES.
Typical settings of NMES exercise for the quadriceps muscle: a frontal view, b lateral view. Two active electrodes are positioned over the motor points of vastus medialis and vastus lateralis muscles, with one dispersive electrode closing the stimulation loop. The stimulated limb is maintained in isometric conditions so as to record NMES force; this latter is then expressed relative to MVC force to provide NMES training intensity (see Fig. 5). Current intensity is consistently increased by the subject themselves to the maximal tolerated level throughout the session
Main applications of NMES
NMES is used as a valid research tool for in vivo assessment of the neuromuscular function of healthy and impaired muscles, in both fresh and fatigued conditions (Horstman et al. 2008; Martin et al. 2004; Wust et al. 2008). As an example, with this technique it is possible to evaluate the contractile function of intact muscle in a standardized way (e.g., force–frequency relationship, fatigability during constant stimulation) as well as the level of voluntary activation using the twitch interpolation technique (Gandevia 2001).
More importantly, NMES is largely adopted in both research and clinical settings as a rehabilitation/training method [henceforth referred to as (re)training]. Depending on the status of the muscle being stimulated, NMES can be used (1) for the preservation of muscle mass and function during prolonged periods of disuse or immobilization (Gibson et al. 1988), (2) for the recovery of muscle mass and function following prolonged periods of disuse or immobilization (Snyder-Mackler et al. 1995), (3) for the improvement of muscle function in different healthy populations: elderly subjects (Caggiano et al. 1994), adult subjects (Currier and Mann 1983), recreational and competitive athletes (Babault et al. 2007; Delitto et al. 1989; Maffiuletti et al. 2002a; Pichon et al. 1995), and also as a preoperative strengthening modality (Walls et al., personal communications), i.e. “prehabilitation” (Fig. 2).
Thus, NMES (re)training programs have been implemented in the following fields:
-
Cardiovascular medicine Patients with chronic or refractory heart failure (Harris et al. 2003; Quittan et al. 2001), cardiac transplant (Vaquero et al. 1998), chronic obstructive pulmonary disease (Roig and Reid 2009; Vivodtzev et al. 2008);
-
Orthopedic medicine Patients with anterior cruciate ligament reconstruction (Delitto et al. 1988; Eriksson and Haggmark 1979; Fitzgerald et al. 2003; Lieber et al. 1996; Snyder-Mackler et al. 1991), bone fracture (Gibson et al. 1988), knee osteoarthritis (Gibson et al. 1989; Zizic et al. 1995), rheumatoid arthritis (Piva et al. 2007), total knee arthroplasty (Petterson and Snyder-Mackler 2006; Stevens et al. 2004), total hip arthroplasty (Suetta et al. 2004), meniscectomy (Gould et al. 1983), patellofemoral pain (Callaghan et al. 2001);
-
Neurological medicine Patients following stroke (Glinsky et al. 2007; Newsam and Baker 2004), spinal cord injury (Belanger et al. 2000; Crameri et al. 2000; Dudley et al. 1999), cerebral palsy (Merrill 2009; Stackhouse et al. 2007);
-
General medicine patients with hemophilia (Querol et al. 2006), cancer (Crevenna et al. 2006) and critically ill patients (Gerovasili et al. 2009);
-
Geriatric medicine Healthy (Amiridis et al. 2005; Caggiano et al. 1994) and unhealthy (Stevens et al. 2004) elderly subjects;
-
Space medicine Astronauts (Convertino 1996; Mayr et al. 1999), simulated microgravity (Duvoisin et al. 1989);
-
Sports medicine Healthy and injured athletes of individual and team sports (Delitto et al. 1989; Maffiuletti 2006).
Surprisingly, such an extensive use of NMES has been mainly restricted to the quadriceps femoris muscle (Bax et al. 2005; Hortobagyi et al. 1992).
Interests of NMES
The most unique aspect of NMES is the activation order of motor units, which is quite different from the physiological recruitment pattern (“size principle” of Henneman et al. 1965) and would favor the activation of fast motor units in addition to the slow ones (i.e., “disorderly” recruitment), even at relatively low levels of evoked force (Gregory and Bickel 2005) (see “Physiological considerations for the use of NMES”). This unique feature has important implications for the use of NMES in the context of rehabilitation and sport training, e.g., for those patients presenting with atrophy of fast muscle fibers (Gosker et al. 2002) or for athletes requiring high levels of muscle strength and power (Babault et al. 2007; Delitto et al. 1989).
The use of NMES as a (re)training modality allows preservation (Gibson et al. 1988) or recovery (Eriksson and Haggmark 1979) of muscle mass and function in patients, and improves muscle strength in healthy subjects (Bax et al. 2005; Gondin et al. 2005) and sportsmen (Maffiuletti 2006). Compared to voluntary training and conventional rehabilitation procedures, NMES (combined or not with voluntary exercise) could be (1) more effective to preserve muscle function during a phase of reduced activity/immobilization (Bax et al. 2005; Glinsky et al. 2007; Vivodtzev et al. 2006), (2) equally effective to recover muscle function after an immobilization period (Bax et al. 2005), also when treatment intensities are matched (Lieber et al. 1996), (3) equally or less effective to improve healthy muscle function (Bax et al. 2005; Hainaut and Duchateau 1992) (Fig. 2). That is to say, NMES (re)training may provide better results than volitional training only for partially or totally immobilized subjects (to substitute for voluntary activation of muscle), when they are unable to perform volitional exercise or to sustain an adequate volitional training intensity and duration to gain benefit from the intervention, e.g., because of breathlessness in cardiovascular patients (Vivodtzev et al. 2008), limited or no cooperation in neurological (Glinsky et al. 2007) and critically ill patients (Gerovasili et al. 2009), reflex inhibition (Morrissey 1988) and limited effectiveness of voluntary isometric training in immobilized orthopedic patients (Eriksson and Haggmark 1979; Gould et al. 1983). It seems that more impaired patients would even respond more effectively to NMES (Morrissey 1988; Roig and Reid 2009).
Contrary to voluntary training procedures, NMES can be used to selectively (re)train specific muscles, e.g., erector spinae for low-back pain prevention (Kahanovitz et al. 1987), or to alter the relative magnitude of recruitment of certain heads within a muscle group (Lake 1992), e.g., selective stimulation of the vastus medialis in subjects with patellofemoral problems (Morrissey 1988). Additionally, the acute application of NMES has been found to promote unique facilitatory effects of the contralateral homologous muscle (Howard and Enoka 1991), and NMES (re)training has been shown to result in greater cross-education compared to voluntary training (Hortobagyi et al. 1999). Such cross-limb effects of NMES are likely to be especially important in patients with disorders predominantly affecting one side of the body.
Limits of NMES
The two main limitations of NMES are the strong discomfort associated with the peripheral stimulation (Delitto et al. 1992; Lake 1992) and the limited spatial recruitment of muscle fibers, which is quite superficial (Vanderthommen et al. 2003) and largely incomplete (see “Physiological considerations for the use of NMES”). Both of these factors, which are strictly related to the current dose, inevitably limit the use of NMES in frail subjects as well as its effectiveness as a valid treatment intervention. In order to improve NMES acceptability, researchers have long attempted to minimize the discomfort and maximize the spatial recruitment by altering the characteristics of NMES parameters (see “Methodological considerations for the use of NMES”), but only with limited success.
Peripherally, NMES exercise imposes a great metabolic demand and thus hastens the onset of muscle fatigue, mainly because of the repeated contractile activity within the same muscle fibers (i.e., fixed recruitment). Moreover, as stimulations are generally delivered at a fixed joint angle (Lieber and Kelly 1993), the effects of NMES (re)training programs are considered to be poorly related to functional activities of daily living or to sporting activities (Enoka 2002b). Methodological precautions may nevertheless be taken to minimize the impact of these limitations on NMES application (Maffiuletti et al. 2002a).
Aims of the review
As discussed above, the use of NMES (re)training could be particularly appropriate during a period of reduced activity/immobilization. Paradoxically, however, the large majority of the NMES (re)training studies have focused on healthy rather than on impaired muscles (Bax et al. 2005), so that the real potential of NMES remains largely unexplored. Additionally, several clinicians are still reluctant to apply NMES in patient populations because of the unclear benefits compared to volitional training, and because of the scarce knowledge of the physiological (Lieber 1986) and methodological issues (Reed 1997; Vanderthommen and Duchateau 2007) related to its use. As an example, there is still a lack of objective evidence on how motor units are activated by the electrical current (see Gregory and Bickel 2005), as well as on the central mechanisms that mediate acute adjustments and chronic adaptations in response to NMES (Collins 2007; Vanderthommen and Duchateau 2007). In the same way, since manufacturers’ claims and personal experience generally prevail over scientific evidence, NMES stimulation parameters are often determined on an empirical basis (Reed 1997; Vanderthommen and Duchateau 2007).
Therefore, the main aim of this review is to discuss some evidence-based physiological and methodological considerations for optimal use of NMES in healthy and impaired skeletal muscles. The intention is to provide practical information for clinicians, physical trainers and researchers working with NMES.
Physiological considerations for the use of NMES
This section will focus on two aspects of NMES physiology that have been the subject of numerous discussions in the last 20 years: the differences in motor unit recruitment pattern between NMES and voluntary contractions, and the involvement of the nervous system during peripheral NMES.
Motor unit recruitment
The involvement of motor units during NMES contractions is considerably different from that underlying voluntary activation (Table 1). The first logical difference between the two modalities of activation deals with the temporal recruitment of motor units, which is quite asynchronous during voluntary actions, while it is imposed by the stimulator in a synchronous manner during NMES (Adams et al. 1993).
With regard to spatial recruitment, constant-intensity NMES imposes a continuous contractile activity to the same population of superficial muscle fibers (i.e., those with the axonal branches in proximity to the stimulating electrodes), and such fixed recruitment diminishes proportionally with increasing distance from the electrode (Vanderthommen et al. 2000). On the other hand, if current intensity is progressively increased throughout the (re)training session (Theurel et al. 2007), new fibers located at a greater distance from the electrode (i.e., deeper) could be depolarized, while the superficial ones maintain their contractile activity despite the occurrence of neuromuscular transmission-propagation failure (Zory et al. 2005). Using magnetic resonance imaging to map the muscle activity elicited by very high-intensity NMES [up to 75% of maximal voluntary contraction (MVC) force], Adams et al. (1993) indicated that the recruitment pattern was not exclusively superficial, but rather dispersed and different among subjects. Despite a relatively small sample size (N = 7 healthy subjects), they also proposed a formula to predict the activated muscle cross-sectional area as a function of NMES training intensity (Fig. 3). This formula substantiates the main limitation of NMES-induced contractions that is the limited spatial recruitment of muscle fibers. As an example, for the normal range of NMES training intensities previously discussed (40–60% MVC), the amount of activated cross-sectional area would only be 29–43% of the total muscle, which indirectly suggests that only a limited portion of the targeted muscle could be (re)trained by NMES.
Quadriceps muscle cross-sectional area (CSA) activated by NMES can be predicted using NMES training intensity (% of MVC), according to the equation proposed by Adams et al. (1993)
As already discussed, superficial, fixed and incomplete muscle recruitment with NMES does not represent good reasons for the use of this modality in the context of muscle (re)training. However, there are at least three strategies that would maximize spatial recruitment during NMES exercise. First, stimulation intensity should be increased whenever possible by the subject themselves, ideally after each contraction, in order to depolarize new and deeper muscle fibers at each evoked contraction. Secondly, stimulation electrodes should be moved after a series of contractions (within the same session and between training sessions), so as to change the population of superficial fibers preferentially activated by NMES current. Thirdly, it is also recommended to alter muscle length by manipulating joint angle, to vary the position of muscle fibers with respect to the electrode, but also to modify the likely contribution of cutaneous (Garnett and Stephens 1981) and joint receptors to the evoked contraction.
The main argument supporting differences in motor unit recruitment order between voluntary and stimulated contractions is that large-diameter axons are more easily excited by electrical stimuli (i.e., they have the lowest threshold of activation), which would reverse the activation order during NMES (Enoka 2002a). This is even favored by anatomical factors, at least for the quadriceps muscle, since larger motor units are mainly located in superficial regions of the vastus lateralis muscle (Knight and Kamen 2005), which would inevitably reduce the distance between the larger axons and the active electrode. Moreover, input from cutaneous afferents via reflex inhibition may alter the recruitment threshold of motor units, as demonstrated by Garnett and Stephens (1981), and favor reversal of motor unit recruitment order. However, human studies have produced contradictory findings on motor unit recruitment order with some studies suggesting preferential or selective activation of fast motor units with NMES (Cabric et al. 1988; Heyters et al. 1994; Trimble and Enoka 1991), and others demonstrating minimal or no difference between voluntary and electrically elicited contractions (Binder-Macleod et al. 1995; Feiereisen et al. 1997; Knaflitz et al. 1990). In an excellent review paper, Gregory and Bickel (2005) suggested that motor unit recruitment during NMES is nonselective or random (see also Jubeau et al. 2007); that is motor units are activated without obvious sequencing related to unit types (i.e., “disorderly” recruitment). This implies that NMES can activate some fast motor units, in addition to slow units, even at relatively low force levels. Indirect evidence suggests that the relative proportion of fast and slow motor units in a muscle activated by NMES at different force levels would be quite constant, as twitch contractile speeds were not found to differ between NMES training intensities of 20, 40 and 80% of MVC (Binder-Macleod et al. 1995).
Such peculiarity of NMES recruitment inevitably entails some disadvantages (e.g., onset and extent of muscle fatigue, see below) but also several advantages, particularly for impaired muscles. For example, elderly individuals and patients presenting a selective atrophy of type II muscle fibers (e.g., chronic obstructive pulmonary disease, chronic steroid myopathy) (Gosker et al. 2002; Kanda et al. 2001), or orthopedic patients who cannot perform high-intensity voluntary contractions because of injury, recent surgery or impaired activation (Petterson and Snyder-Mackler 2006; Stevens et al. 2004), and also athletes requiring high levels of muscle strength and power (Babault et al. 2007; Delitto et al. 1989; Malatesta et al. 2003), would benefit from the use of NMES exercise—even at low intensity—to (re)train at least some of the fast fibers that otherwise can only be activated using high-force voluntary efforts.
The main consequence of such a unique motor unit recruitment pattern for NMES is the exaggerated metabolic cost of an electrically evoked contraction (Vanderthommen et al. 2003), which, compared to a voluntary action of the same intensity, provokes greater and earlier muscle fatigue (Deley et al. 2006; Jubeau et al. 2008; Theurel et al. 2007). According to Vanderthommen and Duchateau (2007), these differences, in motor unit recruitment and thus in metabolic demand between NMES and voluntary contractions, constitute an argument in favor of the non-concomitant combination of these two techniques in the context of muscle (re)training. Differences in spatial recruitment between these two activation modalities, would also contribute, at least in part, to the significant muscle damage produced by NMES but not by voluntary isometric contractions of the same intensity (Jubeau et al. 2008).
Involvement of the nervous system during NMES
Although NMES is commonly viewed as a technique to induce muscle contractions with a negligible contribution of the central nervous system, several lines of evidence indicate a considerable involvement of different neural structures during NMES. These central effects of NMES have been increasingly acknowledged in the last few years (Enoka 2002a; Vanderthommen and Duchateau 2007). In accordance with this contention, it has even been suggested that NMES would provide a multimodal bombardment of the central nervous system (Baker et al. 2000), which results in increased cortical activity (Smith et al. 2003) as well as in spinal motoneuron recruitment (Collins 2007).
Quadriceps NMES, as it is used in clinical settings, has been shown to acutely increase the hemodynamic response in sensorimotor cortex regions (Smith et al. 2003). Importantly, this previous MRI study showed a dose–response relationship between the current intensity and cortical activity, which allows speculation that high current doses (see “Methodological considerations for the use of NMES”) would maximize these supraspinal effects of NMES exercise. On the other hand, the use of wide-pulse (1 ms) high-frequency (50–100 Hz) NMES would favor the recruitment of spinal motoneurons by the electrically evoked sensory volley, leading to the development of central torque (shaded area in Fig. 4), in addition to the peripheral depolarization of motor axons (Collins 2007). Such reflexive recruitment, which activates motor units in the normal physiological recruitment order, is consistent with the development of persistent inward currents in spinal motoneurons or interneurons (Collins 2007) that lead to sustained depolarizations of sensory axons (plateau potentials). Interestingly, central torque can be further maximized by the use of frequencies higher than 80 Hz, long stimulation trains (approximately 20 s) and low contraction intensities (approximately 10% of MVC) (Dean et al. 2007). Even though this spinal contribution remains to be demonstrated for commonly stimulated muscles such as the quadriceps femoris, some of the neural mechanisms that mediate the acute adjustments in response to NMES exercise are now better identified due to these recent findings.
Representative elbow flexor force traces recorded during conventional NMES (grey line) and wide-pulse high-frequency NMES (black line) of the biceps brachii muscle in a healthy subject. The shaded area indicates the central torque due to the reflexive recruitment of spinal motoneurons by the electrically evoked sensory volley (Collins 2007)
The central and peripheral mechanisms of muscle fatigue induced by a conventional session of NMES have been investigated in our laboratory (Boerio et al. 2005), in an attempt to substantiate the acute neural adjustments to NMES exercise. Interestingly, after only 13 min of intermittent NMES of the calf muscles, the exercise-induced reduction in plantar flexor MVC torque (i.e., muscle fatigue) was accompanied by both peripheral (neuromuscular transmission-propagation failure) and central (voluntary activation failure) fatigue, as witnessed by M wave and interpolated twitch findings, respectively (Boerio et al. 2005). Failure of voluntary activation after a single bout of calf NMES has been confirmed and debated recently by Papaiordanidou et al. (2010). As no depression in spinal reflex excitability was reported (Boerio et al. 2005; Papaiordanidou et al. 2010; Tanino et al. 2003), central fatigue induced by NMES could originate from supraspinal rather than from spinal mechanisms. However, we failed to observe a similar neural impairment immediately after application of the same NMES protocol to the quadriceps muscle (Zory et al. 2005), while others have reported a tendency toward a decrease in voluntary activation (Deley et al. 2006), and a significant decline in quadriceps voluntary activation 2 days after the NMES bout (Laurin et al., personal communications).
Besides fatigue experiments, a series of NMES (re)training studies have provided compelling evidence of neural adaptations induced by short-term programs on healthy and impaired muscles. These adaptations include: significant increases in MVC strength after only a few sessions of NMES (Brocherie et al. 2005; Pichon et al. 1995), when there is no reason to believe that increased protein synthesis could have induced significant muscle hypertrophy; strength increases without any significant changes in muscle enzyme activity, fiber size, or mitochondrial properties (Eriksson et al. 1981) (for a contrary view see (Cabric et al. 1988); voluntary strength gains of the contralateral homologous muscle after unilateral (re)training (i.e., cross-education effect) (Hortobagyi et al. 1999; Lai et al. 1988); and increases in voluntary muscle activation, as witnessed by surface electromyography (Gondin et al. 2006; Maffiuletti et al. 2002b), twitch interpolation (Maffiuletti et al. 2002b; Stevens et al. 2004), and volitional-wave (Gondin et al. 2006) recordings. Surprisingly, NMES (re)training does not seem to influence H reflex excitability (Gondin et al. 2006; Maffiuletti et al. 2003), both at rest and during an actual contraction, so it has been inferred that NMES (re)training-induced adaptations would be mainly located at the supraspinal level.
Interestingly, the time course of neuromuscular adaptations to NMES (re)training appears very similar to the classical model proposed by Sale (1988) for voluntary strength training. In their well-designed, prospective study, Gondin et al. (2005) demonstrated that 4 weeks of NMES significantly increased MVC strength and voluntary activation, while muscle cross-sectional area was not significantly altered. After 8 weeks of NMES (re)training, both neural and muscular adaptations mediated the strength improvement, therefore demonstrating that NMES could elicit morphological changes in the muscle, but only for programs longer than 4 weeks. There is, however, a lack of knowledge on the effects of NMES at the single muscle fiber level, and further studies are required to confirm that NMES (re)training actually induces changes in myosin heavy chain isoforms relative content, single-fiber cross-sectional area and specific tension (Maffiuletti et al. 2006).
In light of the above considerations, one can conjecture that conventional NMES (re)training using high training intensities would mainly promote supraspinal adaptations, while wide-pulse high-frequency NMES would likely result in changes at the spinal level. In the same way, the former NMES modality would probably train populations of slow and fast muscle fibers, while the latter would preferentially target slow fibers. These speculation, however, remain to be confirmed by controlled studies in both healthy and patient populations.
In conclusion, the application of peripheral NMES could evoke widespread activity within the central nervous system that is capable of mediating a range of neural adjustments and adaptations. Such central contributions to electrically elicited contractions cannot be ignored any longer.
Methodological considerations for the use of NMES
This section will concentrate on the most common NMES parameters, which entail the characteristics of both the electrical current (the input) and the evoked contraction (the output), and try to provide some clear indications on their appropriate application.
As a foreword, it is important to point out that the effectiveness of a NMES (re)training program does not rely, for the most part, on NMES parameters. Indeed, there is considerable inter-individual variation in response to NMES exercise, and optimization may relate more to the characteristics of the subject than to NMES parameters themselves (Lloyd et al. 1986). I strongly concur with Lieber and Kelly (1991) on the suggestion that NMES effectiveness would not depend on external controllable factors (e.g., current or electrode characteristics), but rather on some intrinsic anatomical properties, such as individual motor nerve branching, which determines the response of the muscle to the application of electrical current over the skin. It is tempting to suggest that NMES success is essentially determined by features that can only be partly controlled by the end user.
In general, NMES current parameters are poorly reported and there is a considerable heterogeneity between the different studies. In the same way, the methods used by researchers to evaluate NMES (re)training effectiveness are quite heterogeneous, so that it is difficult to compare the outcomes of the different NMES studies. Unfortunately, researchers and clinicians tend to consider the different forms of electrical stimulation as a whole, irrespective of the species (human vs. animal), of the model (chronic low frequency stimulation, functional electrical stimulation, transcutaneous electrical nerve stimulation, NMES), of the type of electrode (implanted vs. surface), of the stimulus parameters (subsensory, sensory, motor or supramotor current levels) and of the muscle being stimulated. This inevitably creates confusion and a lack of general consensus regarding the main stimulus parameters for NMES of human skeletal muscles (Maffiuletti 2008; Vanderthommen and Duchateau 2007).
There is nevertheless a sort of agreement on some NMES current characteristics (Vanderthommen and Duchateau 2007). The key factor for optimizing NMES effectiveness has been suggested to be muscle tension, that is the level of evoked force with respect to maximal voluntary force (Lieber and Kelly 1991), which should be maximized, whenever possible, via an appropriate manipulation of the two main NMES current parameters: frequency and intensity. In order to maximize muscle tension, it is strongly recommended to use biphasic rectangular pulses of 100–400 μs delivered at a stimulation frequency of 50–100 Hz (Vanderthommen and Duchateau 2007) and at the highest tolerated current intensity (Lake 1992), and to apply NMES in a static loading condition, so as to strictly control the level of evoked force. It is a common procedure to quantify isometric MVC force at the beginning of a NMES session, and subsequently express the level of each electrically elicited contraction as a percentage of the MVC force (Fig. 5). This important variable, which provides an indication of the intensity of the NMES training achieved, also called NMES training intensity (Selkowitz 1985) or NMES dose (Stevens et al. 2004), is not commonly controlled in both research and clinical settings, therefore invalidating comparisons between studies and between subjects.
There are several lines of evidence indicating that the higher the NMES training intensity, the higher the effectiveness of NMES (re)training, for both healthy (Lai et al. 1988; Miller and Thepaut-Mathieu 1993; Selkowitz 1985) and impaired muscles (Snyder-Mackler et al. 1994; Stevens et al. 2004). Although few NMES studies actually measured the force generated during the stimulation, typical NMES training intensities for the healthy quadriceps range from 40 to 60% of MVC force, while tension levels greater than 70% of MVC are extremely rare to obtain (Lieber and Kelly 1991). As a unique finding, NMES training intensity was found to exceed the individual’s MVC (110% of MVC) in a competitive power lifter (Delitto et al. 1989). Despite low NMES training intensities during the first few training sessions (in subjects non-familiarized with NMES), very large increases in current dose and therefore in training intensity are naturally observed during the course of conventional NMES (re)training programs (Lieber et al. 1996; Maffiuletti et al. 2009), due to improved tolerance or accommodation to NMES. It is therefore recommended to adequately familiarize subjects with the NMES current before initiating a (re)training program, in order to maximize training intensity throughout the intervention. Two or three sessions distributed over 7–10 days seem appropriate for this aim.
However, high NMES training intensities cannot always be used with frail populations, mainly because of the discomfort associated with high current doses. The same is true for obese-overweight subjects and for women, who present with large amounts of subcutaneous fat thickness that inevitably limit current diffusion to the targeted muscle (Maffiuletti et al. 2008). Surprisingly, however, NMES (re)training intensities as low as 5% MVC have been shown to be effective for the preservation of muscle mass during limb immobilization (Gibson et al. 1988) and also for the improvement of muscle strength in healthy individuals (Stefanovska and Vodovnik 1985). Lai et al. (1988) clearly demonstrated that high training intensities (50% MVC) produced greater strength gains than low training intensities (25% MVC) in healthy subjects. Nevertheless, a single investigation having compared the effect of low versus high NMES training intensity on mass and function of impaired muscles is still lacking and only a randomized controlled trial will definitely answer this question.
As a practical recommendation, the level of force evoked by NMES should not necessarily be measured during each training session, because of the linear relationship existing between current intensity and NMES force (Fig. 6) (i.e., the latter can be predicted from the former). However, individual current intensity should be consistently measured and, whenever possible, related to the evoked force to give an estimate of stimulation efficiency (in Newtons or Newton-meters per milliamperes) (Lieber and Kelly 1991). It is also recommended to stimulate the muscle under resting conditions in order to facilitate the quantification of pure NMES evoked force. Nevertheless, frail or current-sensitive subjects could be asked to voluntarily contract their muscle during the first few evoked contractions of a session and/or during the first training sessions of a program so as to decrease the sensation of discomfort associated with NMES. In terms of training effectiveness, whether NMES is performed alone or superimposed with voluntary contraction does not seem to have an influence on the training-induced strength gains (Bax et al. 2005; Currier and Mann 1983; Lieber 1986; Malatesta et al. 2003).
When presenting NMES parameters, it is important to clearly differentiate the characteristics of the electrical current from the characteristics of the evoked contraction (Fig. 7), because the former do not necessarily allow prediction of the latter on an individual basis. Moreover, hardware features and (re)training program parameters, including subject compliance with and tolerance to the NMES intervention, should always be carefully reported in scientific studies. It is also recommended to follow a linear progression in the main NMES training parameters such as current intensity, evoked force and training volume (Maffiuletti et al. 2009), to respect the basic principles of progressive strength training.
Concluding remarks
A good knowledge of the physiological and methodological features of NMES discussed here would allow optimization of NMES use in the clinic and for research purposes. The end user would then turn limitations and unique aspects of NMES to their advantage, so as to ensure effectiveness and safety. As an example, the “disorderly” recruitment of motor units with NMES could be minimized by using nerve instead of muscle stimulation (which would reduce the “confusion”), and wide-pulse high-frequency stimulations to favor the physiological motor unit recruitment order (Collins 2007). In the same way, central effects of NMES could be maximized using wide pulses, high frequencies, long stimulations and low NMES training intensities (Dean et al. 2007). Finally, spatial recruitment of motor units should be maximized by adopting different subterfuges during the training session such as a progressive increase in current intensity, displacement of active electrodes and alteration in muscle length.
References
Adams GR, Harris RT, Woodard D, Dudley GA (1993) Mapping of electrical muscle stimulation using MRI. J Appl Physiol 74:532–537
Amiridis I, Arabatzi F, Violaris P, Stavropoulos E, Hatzitaki V (2005) Static balance improvement in elderly after dorsiflexors electrostimulation training. Eur J Appl Physiol 94:424–433
Babault N, Cometti G, Bernardin M, Pousson M, Chatard JC (2007) Effects of electromyostimulation training on muscle strength and power of elite rugby players. J Strength Cond Res 21:431–437
Baker LL, Wederich C, McNeal D, Newsam CJ, Waters RL (2000) Neuromuscular electrical stimulation: a practical guide. Los Amigos Research and Educational Institute, Downey, CA
Bax L, Staes F, Verhagen A (2005) Does neuromuscular electrical stimulation strengthen the quadriceps femoris? A systematic review of randomised controlled trials. Sports Med 35:191–212
Belanger M, Stein RB, Wheeler GD, Gordon T, Leduc B (2000) Electrical stimulation: can it increase muscle strength and reverse osteopenia in spinal cord injured individuals? Arch Phys Med Rehabil 81:1090–1098
Binder-Macleod SA, Halden EE, Jungles KA (1995) Effects of stimulation intensity on the physiological responses of human motor units. Med Sci Sports Exerc 27:556–565
Boerio D, Jubeau M, Zory R, Maffiuletti NA (2005) Central and peripheral fatigue after electrostimulation-induced resistance exercise. Med Sci Sports Exerc 37:973–978
Brocherie F, Babault N, Cometti G, Maffiuletti N, Chatard JC (2005) Electrostimulation training effects on the physical performance of ice hockey players. Med Sci Sports Exerc 37:455–460
Cabric M, Appell HJ, Resic A (1988) Fine structural changes in electrostimulated human skeletal muscle. Evidence for predominant effects on fast muscle fibres. Eur J Appl Physiol Occup Physiol 57:1–5
Caggiano E, Emrey T, Shirley S, Craik RL (1994) Effects of electrical stimulation or voluntary contraction for strengthening the quadriceps femoris muscles in an aged male population. J Orthop Sports Phys Ther 20:22–28
Callaghan MJ, Oldham JA, Winstanley J (2001) A comparison of two types of electrical stimulation of the quadriceps in the treatment of patellofemoral pain syndrome. A pilot study. Clin Rehabil 15:637–646
Collins DF (2007) Central contributions to contractions evoked by tetanic neuromuscular electrical stimulation. Exerc Sport Sci Rev 35:102–109
Convertino VA (1996) Exercise as a countermeasure for physiological adaptation to prolonged spaceflight. Med Sci Sports Exerc 28:999–1014
Crameri RM, Weston AR, Rutkowski S, Middleton JW, Davis GM, Sutton JR (2000) Effects of electrical stimulation leg training during the acute phase of spinal cord injury: a pilot study. Eur J Appl Physiol 83:409–415
Crevenna R, Marosi C, Schmidinger M, Fialka-Moser V (2006) Neuromuscular electrical stimulation for a patient with metastatic lung cancer—a case report. Support Care Cancer 14:970–973
Currier DP, Mann R (1983) Muscular strength development by electrical stimulation in healthy individuals. Phys Ther 63:915–921
Dean JC, Yates LM, Collins DF (2007) Turning on the central contribution to contractions evoked by neuromuscular electrical stimulation. J Appl Physiol 103:170–176
Deley G, Millet GY, Borrani F, Lattier G, Brondel L (2006) Effects of two types of fatigue on the VO(2) slow component. Int J Sports Med 27:475–482
Delitto A, Rose SJ, McKowen JM, Lehman RC, Thomas JA, Shively RA (1988) Electrical stimulation versus voluntary exercise in strengthening thigh musculature after anterior cruciate ligament surgery. Phys Ther 68:660–663
Delitto A, Brown M, Strube MJ, Rose SJ, Lehman RC (1989) Electrical stimulation of quadriceps femoris in an elite weight lifter: a single subject experiment. Int J Sports Med 10:187–191
Delitto A, Strube MJ, Shulman AD, Minor SD (1992) A study of discomfort with electrical stimulation. Phys Ther 72:410–421 (discussion on 421–424)
Dudley GA, Castro MJ, Rogers S, Apple DF Jr (1999) A simple means of increasing muscle size after spinal cord injury: a pilot study. Eur J Appl Physiol Occup Physiol 80:394–396
Duvoisin MR, Convertino VA, Buchanan P, Gollnick PD, Dudley GA (1989) Characteristics and preliminary observations of the influence of electromyostimulation on the size and function of human skeletal muscle during 30 days of simulated microgravity. Aviat Space Environ Med 60:671–678
Enoka RM (2002a) Activation order of motor axons in electrically evoked contractions. Muscle Nerve 25:763–764
Enoka RM (2002b) Neuromechanics of human movement. Human Kinetics, Champaign, IL
Eriksson E, Haggmark T (1979) Comparison of isometric muscle training and electrical stimulation supplementing isometric muscle training in the recovery after major knee ligament surgery. A preliminary report. Am J Sports Med 7:169–171
Eriksson E, Haggmark T, Kiessling KH, Karlsson J (1981) Effect of electrical stimulation on human skeletal muscle. Int J Sports Med 2:18–22
Feiereisen P, Duchateau J, Hainaut K (1997) Motor unit recruitment order during voluntary and electrically induced contractions in the tibialis anterior. Exp Brain Res 114:117–123
Fitzgerald GK, Piva SR, Irrgang JJ (2003) A modified neuromuscular electrical stimulation protocol for quadriceps strength training following anterior cruciate ligament reconstruction. J Orthop Sports Phys Ther 33:492–501
Gandevia SC (2001) Spinal and supraspinal factors in human muscle fatigue. Physiol Rev 81:1725–1789
Garnett R, Stephens JA (1981) Changes in the recruitment threshold of motor units produced by cutaneous stimulation in man. J Physiol 311:463–473
Gerovasili V, Stefanidis K, Vitzilaios K, Karatzanos E, Politis P, Koroneos A, Chatzimichail A, Routsi C, Roussos C, Nanas S (2009) Electrical muscle stimulation preserves the muscle mass of critically ill patients: a randomized study. Crit Care 13:R161
Gibson JN, Smith K, Rennie MJ (1988) Prevention of disuse muscle atrophy by means of electrical stimulation: maintenance of protein synthesis. Lancet 2:767–770
Gibson JN, Morrison WL, Scrimgeour CM, Smith K, Stoward PJ, Rennie MJ (1989) Effects of therapeutic percutaneous electrical stimulation of atrophic human quadriceps on muscle composition, protein synthesis and contractile properties. Eur J Clin Invest 19:206–212
Glinsky J, Harvey L, Van Es P (2007) Efficacy of electrical stimulation to increase muscle strength in people with neurological conditions: a systematic review. Physiother Res Int 12:175–194
Gondin J, Guette M, Ballay Y, Martin A (2005) Electromyostimulation training effects on neural drive and muscle architecture. Med Sci Sports Exerc 37:1291–1299
Gondin J, Duclay J, Martin A (2006) Soleus- and gastrocnemii-evoked V-wave responses increase after neuromuscular electrical stimulation training. J Neurophysiol 95:3328–3335
Gosker HR, Engelen MP, van Mameren H, van Dijk PJ, van der Vusse GJ, Wouters EF, Schols AM (2002) Muscle fiber type IIX atrophy is involved in the loss of fat-free mass in chronic obstructive pulmonary disease. Am J Clin Nutr 76:113–119
Gould N, Donnermeyer D, Gammon GG, Pope M, Ashikaga T (1983) Transcutaneous muscle stimulation to retard disuse atrophy after open meniscectomy. Clin Orthop Relat Res:190–197
Gregory CM, Bickel CS (2005) Recruitment patterns in human skeletal muscle during electrical stimulation. Phys Ther 85:358–364
Hainaut K, Duchateau J (1992) Neuromuscular electrical stimulation and voluntary exercise. Sports Med 14:100–113
Harris S, LeMaitre JP, Mackenzie G, Fox KA, Denvir MA (2003) A randomised study of home-based electrical stimulation of the legs and conventional bicycle exercise training for patients with chronic heart failure. Eur Heart J 24:871–878
Henneman E, Somjen G, Carpenter DO (1965) Functional significance of cell size in spinal motoneurons. J Neurophysiol 28:560–580
Heyters M, Carpentier A, Duchateau J, Hainaut K (1994) Twitch analysis as an approach to motor unit activation during electrical stimulation. Can J Appl Physiol 19:451–461
Horstman AM, Beltman MJ, Gerrits KH, Koppe P, Janssen TW, Elich P, de Haan A (2008) Intrinsic muscle strength and voluntary activation of both lower limbs and functional performance after stroke. Clin Physiol Funct Imaging 28:251–261
Hortobagyi T, Lambert NJ, Tracy C, Shinebarger M (1992) Voluntary and electromyostimulation forces in trained and untrained men. Med Sci Sports Exerc 24:702–707
Hortobagyi T, Scott K, Lambert J, Hamilton G, Tracy J (1999) Cross-education of muscle strength is greater with stimulated than voluntary contractions. Mot Control 3:205–219
Howard JD, Enoka RM (1991) Maximum bilateral contractions are modified by neurally mediated interlimb effects. J Appl Physiol 70:306–316
Hultman E, Sjoholm H, Jaderholm-Ek I, Krynicki J (1983) Evaluation of methods for electrical stimulation of human skeletal muscle in situ. Pflugers Arch 398:139–141
Jubeau M, Gondin J, Martin A, Sartorio A, Maffiuletti NA (2007) Random motor unit activation by electrostimulation. Int J Sports Med 28:901–904
Jubeau M, Sartorio A, Marinone PG, Agosti F, Van Hoecke J, Nosaka K, Maffiuletti NA (2008) Comparison between voluntary and stimulated contractions of the quadriceps femoris for growth hormone response and muscle damage. J Appl Physiol 104:75–81
Kahanovitz N, Nordin M, Verderame R, Yabut S, Parnianpour M, Viola K, Mulvihill M (1987) Normal trunk muscle strength and endurance in women and the effect of exercises and electrical stimulation. Part 2: comparative analysis of electrical stimulation and exercises to increase trunk muscle strength and endurance. Spine (Phila Pa 1976) 12:112–118
Kanda F, Okuda S, Matsushita T, Takatani K, Kimura KI, Chihara K (2001) Steroid myopathy: pathogenesis and effects of growth hormone and insulin-like growth factor-I administration. Horm Res 56(Suppl 1):24–28
Knaflitz M, Merletti R, De Luca CJ (1990) Inference of motor unit recruitment order in voluntary and electrically elicited contractions. J Appl Physiol 68:1657–1667
Knight CA, Kamen G (2005) Superficial motor units are larger than deeper motor units in human vastus lateralis muscle. Muscle Nerve 31:475–480
Lai HS, De Domenico G, Strauss GR (1988) The effect of different electro-motor stimulation training intensities on strength improvement. Aust J Physiother 34:151–164
Lake DA (1992) Neuromuscular electrical stimulation. An overview and its application in the treatment of sports injuries. Sports Med 13:320–336
Lieber RL (1986) Skeletal muscle adaptability III: muscle properties following chronic electrical stimulation. Dev Med Child Neurol 28:662–670
Lieber RL, Kelly MJ (1991) Factors influencing quadriceps femoris muscle torque using transcutaneous neuromuscular electrical stimulation. Phys Ther 71:715–721 (discussion 722–723)
Lieber RL, Kelly MJ (1993) Torque history of electrically stimulated human quadriceps: implications for stimulation therapy. J Orthop Res 11:131–141
Lieber RL, Silva PD, Daniel DM (1996) Equal effectiveness of electrical and volitional strength training for quadriceps femoris muscles after anterior cruciate ligament surgery. J Orthop Res 14:131–138
Lloyd T, De Domenico G, Strauss GR, Singer K (1986) A review of the use of electro-motor stimulation in human muscles. Aust J Physiother 32:18–30
Maffiuletti NA (2006) The use of electrostimulation exercise in competitive sport. Int J Sports Physiol Perform 1:406–407
Maffiuletti NA (2008) Caution is required when comparing the effectiveness of voluntary versus stimulated versus combined strength training modalities. Sports Med 38:437–438 (author reply 438–440)
Maffiuletti NA, Dugnani S, Folz M, Di Pierno E, Mauro F (2002a) Effect of combined electrostimulation and plyometric training on vertical jump height. Med Sci Sports Exerc 34:1638–1644
Maffiuletti NA, Pensini M, Martin A (2002b) Activation of human plantar flexor muscles increases after electromyostimulation training. J Appl Physiol 92:1383–1392
Maffiuletti NA, Pensini M, Scaglioni G, Ferri A, Ballay Y, Martin A (2003) Effect of electromyostimulation training on soleus and gastrocnemii H- and T-reflex properties. Eur J Appl Physiol 90:601–607
Maffiuletti NA, Zory R, Miotti D, Pellegrino MA, Jubeau M, Bottinelli R (2006) Neuromuscular adaptations to electrostimulation resistance training. Am J Phys Med Rehabil 85:167–175
Maffiuletti NA, Herrero AJ, Jubeau M, Impellizzeri FM, Bizzini M (2008) Differences in electrical stimulation thresholds between men and women. Ann Neurol 63:507–512
Maffiuletti NA, Bramanti J, Jubeau M, Bizzini M, Deley G, Cometti G (2009) Feasibility and efficacy of progressive electrostimulation strength training for competitive tennis players. J Strength Cond Res 23:677–682
Malatesta D, Cattaneo F, Dugnani S, Maffiuletti NA (2003) Effects of electromyostimulation training and volleyball practice on jumping ability. J Strength Cond Res 17:573–579
Martin V, Millet GY, Martin A, Deley G, Lattier G (2004) Assessment of low-frequency fatigue with two methods of electrical stimulation. J Appl Physiol 97:1923–1929
Mayr W, Bijak M, Girsch W, Hofer C, Lanmuller H, Rafolt D, Rakos M, Sauermann S, Schmutterer C, Schnetz G, Unger E, Freilinger G (1999) MYOSTIM-FES to prevent muscle atrophy in microgravity and bed rest: preliminary report. Artif Organs 23:428–431
Merrill DR (2009) Review of electrical stimulation in cerebral palsy and recommendations for future directions. Dev Med Child Neurol 51(Suppl 4):154–165
Miller C, Thepaut-Mathieu C (1993) Strength training by electrostimulation conditions for efficacy. Int J Sports Med 14:20–28
Morrissey MC (1988) Electromyostimulation from a clinical perspective. A review. Sports Med 6:29–41
Newsam CJ, Baker LL (2004) Effect of an electric stimulation facilitation program on quadriceps motor unit recruitment after stroke. Arch Phys Med Rehabil 85:2040–2045
Papaiordanidou M, Guiraud D, Varray A (2010) Kinetics of neuromuscular changes during low-frequency electrical stimulation. Muscle Nerve 41:54–62
Petterson S, Snyder-Mackler L (2006) The use of neuromuscular electrical stimulation to improve activation deficits in a patient with chronic quadriceps strength impairments following total knee arthroplasty. J Orthop Sports Phys Ther 36:678–685
Pichon F, Chatard JC, Martin A, Cometti G (1995) Electrical stimulation and swimming performance. Med Sci Sports Exerc 27:1671–1676
Piva SR, Goodnite EA, Azuma K, Woollard JD, Goodpaster BH, Wasko MC, Fitzgerald GK (2007) Neuromuscular electrical stimulation and volitional exercise for individuals with rheumatoid arthritis: a multiple-patient case report. Phys Ther 87:1064–1077
Querol F, Gallach JE, Toca-Herrera JL, Gomis M, Gonzalez LM (2006) Surface electrical stimulation of the quadriceps femoris in patients affected by haemophilia A. Haemophilia 12:629–632
Quittan M, Wiesinger GF, Sturm B, Puig S, Mayr W, Sochor A, Paternostro T, Resch KL, Pacher R, Fialka-Moser V (2001) Improvement of thigh muscles by neuromuscular electrical stimulation in patients with refractory heart failure: a single-blind, randomized, controlled trial. Am J Phys Med Rehabil 80:206–214 (quiz 215–216, 224)
Reed B (1997) The physiology of neuromuscular electrical stimulation. Pediatr Phys Ther 9:96–102
Roig M, Reid WD (2009) Electrical stimulation and peripheral muscle function in COPD: a systematic review. Respir Med 103:485–495
Sale DG (1988) Neural adaptation to resistance training. Med Sci Sports Exerc 20:S135–S145
Selkowitz DM (1985) Improvement in isometric strength of the quadriceps femoris muscle after training with electrical stimulation. Phys Ther 65:186–196
Smith GV, Alon G, Roys SR, Gullapalli RP (2003) Functional MRI determination of a dose–response relationship to lower extremity neuromuscular electrical stimulation in healthy subjects. Exp Brain Res 150:33–39
Snyder-Mackler L, Ladin Z, Schepsis AA, Young JC (1991) Electrical stimulation of the thigh muscles after reconstruction of the anterior cruciate ligament. Effects of electrically elicited contraction of the quadriceps femoris and hamstring muscles on gait and on strength of the thigh muscles. J Bone Jt Surg Am 73:1025–1036
Snyder-Mackler L, Delitto A, Stralka SW, Bailey SL (1994) Use of electrical stimulation to enhance recovery of quadriceps femoris muscle force production in patients following anterior cruciate ligament reconstruction. Phys Ther 74:901–907
Snyder-Mackler L, Delitto A, Bailey SL, Stralka SW (1995) Strength of the quadriceps femoris muscle and functional recovery after reconstruction of the anterior cruciate ligament. A prospective, randomized clinical trial of electrical stimulation. J Bone Jt Surg Am 77:1166–1173
Stackhouse SK, Binder-Macleod SA, Stackhouse CA, McCarthy JJ, Prosser LA, Lee SC (2007) Neuromuscular electrical stimulation versus volitional isometric strength training in children with spastic diplegic cerebral palsy: a preliminary study. Neurorehabil Neural Repair 21:475–485
Stefanovska A, Vodovnik L (1985) Change in muscle force following electrical stimulation. Dependence on stimulation waveform and frequency. Scand J Rehabil Med 17:141–146
Stevens JE, Mizner RL, Snyder-Mackler L (2004) Neuromuscular electrical stimulation for quadriceps muscle strengthening after bilateral total knee arthroplasty: a case series. J Orthop Sports Phys Ther 34:21–29
Suetta C, Aagaard P, Rosted A, Jakobsen AK, Duus B, Kjaer M, Magnusson SP (2004) Training-induced changes in muscle CSA, muscle strength, EMG, and rate of force development in elderly subjects after long-term unilateral disuse. J Appl Physiol 97:1954–1961
Tanino Y, Daikuya S, Nishimori T, Takasaki K, Suzuki T (2003) M wave and H-reflex of soleus muscle before and after electrical muscle stimulation in healthy subjects. Electromyogr Clin Neurophysiol 43:381–384
Theurel J, Lepers R, Pardon L, Maffiuletti NA (2007) Differences in cardiorespiratory and neuromuscular responses between voluntary and stimulated contractions of the quadriceps femoris muscle. Respir Physiol Neurobiol 157:341–347
Trimble MH, Enoka RM (1991) Mechanisms underlying the training effects associated with neuromuscular electrical stimulation. Phys Ther 71:273–280 (discussion 280–272)
Vanderthommen M, Duchateau J (2007) Electrical stimulation as a modality to improve performance of the neuromuscular system. Exerc Sport Sci Rev 35:180–185
Vanderthommen M, Depresseux JC, Dauchat L, Degueldre C, Croisier JL, Crielaard JM (2000) Spatial distribution of blood flow in electrically stimulated human muscle: a positron emission tomography study. Muscle Nerve 23:482–489
Vanderthommen M, Duteil S, Wary C, Raynaud JS, Leroy-Willig A, Crielaard JM, Carlier PG (2003) A comparison of voluntary and electrically induced contractions by interleaved 1H- and 31P-NMRS in humans. J Appl Physiol 94:1012–1024
Vaquero AF, Chicharro JL, Gil L, Ruiz MP, Sanchez V, Lucia A, Urrea S, Gomez MA (1998) Effects of muscle electrical stimulation on peak VO2 in cardiac transplant patients. Int J Sports Med 19:317–322
Vivodtzev I, Pepin JL, Vottero G, Mayer V, Porsin B, Levy P, Wuyam B (2006) Improvement in quadriceps strength and dyspnea in daily tasks after 1 month of electrical stimulation in severely deconditioned and malnourished COPD. Chest 129:1540–1548
Vivodtzev I, Lacasse Y, Maltais F (2008) Neuromuscular electrical stimulation of the lower limbs in patients with chronic obstructive pulmonary disease. J Cardiopulm Rehabil Prev 28:79–91
Wust RC, Morse CI, de Haan A, Jones DA, Degens H (2008) Sex differences in contractile properties and fatigue resistance of human skeletal muscle. Exp Physiol 93:843–850
Zizic TM, Hoffman KC, Holt PA, Hungerford DS, O’Dell JR, Jacobs MA, Lewis CG, Deal CL, Caldwell JR, Cholewczynski JG et al (1995) The treatment of osteoarthritis of the knee with pulsed electrical stimulation. J Rheumatol 22:1757–1761
Zory R, Boerio D, Jubeau M, Maffiuletti NA (2005) Central and peripheral fatigue of the knee extensor muscles induced by electromyostimulation. Int J Sports Med 26:847–853
Acknowledgments
The author thanks: Marco A. Minetto for reading the manuscript and offering useful suggestions; Silvestro Roatta for providing the data presented in Fig. 4; Kirsten Dobson for checking English language; Gilles Cometti, Marc Jubeau, and Alain Martin for their continuous support and enthusiasm.
Author information
Authors and Affiliations
Corresponding author
Additional information
Communicated by Nigel Taylor.
Rights and permissions
About this article
Cite this article
Maffiuletti, N.A. Physiological and methodological considerations for the use of neuromuscular electrical stimulation. Eur J Appl Physiol 110, 223–234 (2010). https://doi.org/10.1007/s00421-010-1502-y
Accepted:
Published:
Issue Date:
DOI: https://doi.org/10.1007/s00421-010-1502-y