Abstract
Gait disorders represent a therapeutic challenge in Parkinson’s disease (PD). This study investigated the efficacy of 4-week action observation training (AOT) on disease severity, freezing of gait and motor abilities in PD, and evaluated treatment-related brain functional changes. 25 PD patients with freezing of gait were randomized into two groups: AOT (action observation combined with practicing the observed actions) and “Landscape” (same physical training combined with landscape-videos observation). At baseline and 4-week, patients underwent clinical evaluation and fMRI. Clinical assessment was repeated at 8-week. At 4-week, both groups showed reduced freezing of gait severity, improved walking speed and quality of life. Moreover, AOT was associated with reduced motor disability and improved balance. AOT group showed a sustained positive effect on motor disability, walking speed, balance and quality of life at 8-week, with a trend toward a persisting reduced freezing of gait severity. At 4-week vs. baseline, AOT group showed increased recruitment of fronto-parietal areas during fMRI tasks, while the Landscape group showed a reduced fMRI activity of the left postcentral and inferior parietal gyri and right rolandic operculum and supramarginal gyrus. In AOT group, functional brain changes were associated with clinical improvements at 4-week and predicted clinical evolution at 8-week. AOT has a more lasting effect in improving motor function, gait and quality of life in PD patients relative to physical therapy alone. AOT-related performance gains are associated with an increased recruitment of motor regions and fronto-parietal mirror neuron and attentional control areas.
Similar content being viewed by others
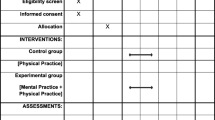
Avoid common mistakes on your manuscript.
Introduction
Evidence is accumulating that gait disorders in patients with Parkinson’s disease (PD), including freezing of gait (FoG), are caused by a complex interplay between motor, cognitive and affective factors, rather than being pure motor phenomena [1, 2]. FoG represents a major therapeutic challenge in Parkinson’s disease (PD), and non-invasive approaches such as physical activity have received much attention in the last decades [3]. Attentional strategies and cueing, aimed to provide compensatory methods for the production of efficient gait patterns, are used by patients successfully to overcome mild FoG [3]. Other rehabilitative strategies combine group exercise and treadmill training with auditory and visual cues [3].
Among attentional strategies, action observation training (AOT) (i.e., the systematic observation of actions followed by their imitation) is a top-down rehabilitation approach exploiting the “mirror” mechanism and its potential role in motor recovery through motor learning consolidation [4]. It is a well-known notion in neurophysiology that the observation of actions performed by others, after being processed in the visual system, are directly mapped onto observers’ mirror neuron system (MNS), which is an observation-execution matching system located in frontal and parietal lobes firing both when a person observes another individual performing a motor act and when he/she performs an identical or similar action [5]. As well as having a role in action understanding, the MNS also modulates the motor behaviour of the observer being involved in motor learning and the building of motor memory [6]. AOT has been successfully applied during stroke rehabilitation [4]. Only a few studies have successfully exploited AOT in PD improving autonomy in daily activity [7], increasing finger movement rate at spontaneous pace [8], and reducing the number of FoG episodes [9].
This prospective study aims at investigating the efficacy of 4 week (W4) AOT on disease severity, FoG and motor functional abilities in patients with PD-FoG. Importantly, we explored brain functional changes following AOT using functional MRI (fMRI) in combination with foot movement execution, motor imagery (MI), and action observation (AO) tasks. We hypothesized that AOT would positively influence the motor performance and gait of PD-FoG patients through a reorganization of motor representations at central level and fronto-parietal attentive networks.
Methods
Sample
Twenty-five consecutive, right-handed outpatients with idiopathic PD were recruited at the Movement Disorders Unit, Department of Neurology, San Raffaele Scientific Institute in Milan according to the following inclusion criteria: (1) occurrence of FoG [i.e., item 3 of the FoG Questionnaire (FoG-Q) [10] ≥2, and at least two of the following: observation of FoG by an experienced neurologist, the participant’s verbal account of whether he/she had experienced FoG, the recognition of typical FoG in the patient’s experience when this was identified and described to him/her by a physician]; (2) disease duration ≥5 years; (3) Hoehn and Yahr scale (H&Y) [11] <4; (4) stable dopaminergic medication regimen for at least 4 weeks; (5) no long-acting medications such as sustained-release dopamine agonists; (6) no levodopa-induced FoG; (7) no dementia (Mini-Mental Status Examination score [MMSE] [12] >24); (8) no depressive symptoms (Beck Depression Inventory [13] <9); and (9) no significant head tremor. At study entry, patients underwent clinical, motor functional and neuropsychological evaluations and MRI scan. Nineteen age- and sex-matched, right-handed, healthy controls (10 women/9 men; age 66 ± 8 years, range 55–81 years) were recruited among non-consanguineous relatives, institute personnel and by word of mouth, and performed neuropsychological and MRI assessments at baseline. Patients and controls were excluded if they had: medical illnesses or substance abuse that could interfere with cognition; any (other) major systemic, psychiatric or neurological illnesses (including musculoskeletal and visual disturbances); (other) causes of gait impairment such as severe arthrosis or neuropathy; brain damage at routine MRI, including lacunae and extensive cerebrovascular disorders. Local ethical standards committee on human experimentation approved the study protocol and all subjects provided written informed consent prior to study participation.
Neuropsychological assessment
The neuropsychological assessment was performed by an experienced neuropsychologist blinded to participants’ allocation and other evaluation findings. Global cognitive functioning was evaluated with the MMSE [12]; memory function with verbal and spatial span [14], Rey auditory verbal learning test [15], and Rey’s Figure Delayed Recall Test [16]; executive functions with the Phonemic and Semantic Fluency [17], and the fluency indices (controlling for individual variations in motor disabilities) [18], Ten Point Clock Drawing Test [19], and Modified Card Sorting Test [20]; attention and working memory functions with digit span backward [21], attentive matrices [22], and Trail Making Test [23]; language with the confrontation naming subtests of the battery for the assessment of aphasic disorders (BADA) [24] and the Token Test [25]; visuospatial abilities with the Rey’s Figure Copy Test [16] and the visual spatial subtests of the Addenbrooke’s Cognitive Examination Revised [26] Mood was evaluated with the Beck Depression Inventory [13]. Scores on neuropsychological tests were age, sex, and education corrected using normative values.
Treatment
At the time of enrolment, patients were randomly assigned to the experimental (labelled “AOT”) or control (labelled “Landscape”) groups by an allocation concealment procedure. The allocations were reported in sheets put in numbered and closed envelopes, opened by a blinded researcher. The list of assignation was generated by a computerized random-number generator. All patients underwent a 60-min physical therapy training (24 min of observation and 36 of imitation), during their ON time, for 3 sessions a week for 4 weeks (12 sessions), as previously described [9]. During each training session, two video clips showing strategies useful in circumventing FoG episodes [9] and lasting 6 min, were presented twice. Overall, subjects in the AOT group were presented six video clips, repeated each week. The complexity of actions progressively increased and auditory cues were associated to the movements. The specific content of each video clip was reported previously [9]. To ensure proper attention during the video clip presentation, patients were explicitly asked to concentrate on how the actions were performed and were not allowed to practice any movement. After each video clip observation, patients were asked to imitate for 8 min the observed actions repetitively and accurately at the beat of the auditory cues. PD patients in the Landscape group matched the experimental protocol, with the only exception that during each training session they watched video clips containing sequences of static pictures of landscapes without any living representations for the same time length [9]. During training sessions, patients in the Landscape group performed the same movements/actions used for the AOT group in the same order and amount of time, following the physical therapist’s instructions. Patients in both groups were continuously encouraged and corrected by the therapist during action performance. The treatment of both groups was performed by two physiotherapists with expertise in AOT.
Clinical outcomes
Patients underwent clinical, motor functional and neuropsychological evaluations during ON time before the physical therapy program (T0), and clinical and motor functional visits at the end of the 12 training sessions (W4) and 4 weeks later (W8). Clinical evaluation was performed by an experienced neurologist, blinded to participants’ allocation and motor functional and MRI findings, and assessed disease severity with the Unified Parkinson’s Disease Rating Scale (UPDRS) III [27] and the HY scale [11], FoG severity with the FoG-Q [10] and UPDRS II FoG score, and quality of life with the 39-item PD questionnaire (PDQ-39) [28]. UPDRS scores and H&Y scales were also obtained during OFF time, before MRI. A motor functional evaluation was performed by a blinded, experienced physical therapist and included the Berg Balance Scale (BBS) [29], and the 10 m walking test (10 M-WT) [30]. During the training period and for the subsequent 4 weeks, participants were allowed to continue their ordinary motor activities; however, they were asked not to practice or undertake any specific physical therapy and no change in medication was permitted.
MRI acquisition
Using a 3.0 Tesla Philips Intera scanner, MRI scans were obtained between 12 AM and 1 PM during OFF time, i.e., at least 12 h after their regular evening dopaminergic therapy administration, to mitigate the pharmacological effects on neural activity. fMRI was obtained using a T2* weighted echo planar imaging sequence with the following parameters: echo time (TE) = 30 ms, repetition time (TR) = 3700 ms (foot movement execution task) or 2500 ms (motor imagery and action observation tasks), flip angle = 85°, field of view (FOV) = 240 × 240 mm, matrix = 128 × 128, 168 sets of 30 axial slices, slice thickness = 4 mm, acquisition time = 7 min. Subjects were scanned during three conditions: (1) foot movement execution, (2) MI; and (3) AO. All the stimuli were built using Presentation 13.0 Build 01.23.09 (http://www.neurobs.com).
The following structural brain sequences were also acquired: T2-weighted spin echo (TR = 3500 ms; echo time TE = 85 ms; echo train length = 15; flip angle = 90°; 22 contiguous, 5-mm-thick, axial slices; matrix size = 512 × 512; FOV = 230 × 184 mm2); fluid-attenuated inversion recovery (TR = 11 s; TE = 120 ms; flip angle = 90°; 22 contiguous, 5-mm thick, axial slices; matrix size = 512 × 512; FOV = 230 mm2); and 3D T1-weighted fast field echo (TR = 25 ms, TE = 4.6 ms, flip angle = 30°, 220 contiguous axial slices with voxel size = 0.89 × 0.89 × 0.8 mm, matrix size = 256 × 256, FOV = 230 × 182 mm2). All slices were positioned to run parallel to a line that joins the most inferoanterior and inferoposterior parts of the corpus callosum.
fMRI tasks
During the foot movement execution task, participants were asked to move their feet alternately in dorsal and plantar flexion at a frequency of 0.5 Hz according to an auditory stimulus and with their eyes closed. The task was performed with the knees supported by a soft wedge and flexed of about 30°, and to avoid any bias due to different amplitude of the movement the subjects were asked to reach with their insteps a fixed wood bar. In this way, the dorsal flexion was about 90° for all the subjects. A block design (ABAB) was used, in which the activation A (lasting 18.5 s) corresponded to the dorsal and plantar flexion, while during the resting period B (lasting 18.5 s) subjects were asked to maintain their eyes closed. To ensure correct task performance, subjects were monitored visually by an observer inside the scanner room during scanning. Tasks were performed equally well by all the subjects.
During the MI task, subjects were asked to imagine themselves performing three actions exacerbating FoG, i.e., starting and stopping walking in a narrow hallway, turning around 360° in a small radius, and going through a doorway. MI task was performed with eyes open and while subjects were asked to navigate first-person videos of realistic environments such as a narrow corridor, a small room and a sliding door, respectively. Participants were instructed to focus their attention on their body and to imagine moving the body parts involved in the task. During the AO task, subjects were asked to observe third-person videos representing a person performing the same three actions, in the same environments, presented during the MI task. A block design (ABAB) was used, in which activation periods (A) alternated with resting-state periods (B). Each block consisted of a video which lasted 20 s followed by a 15 s rest period where the frame of the subsequent video was displayed. The start of a new trial was indicated by a green arrow, while the stop was indicated by a red hand. The order of presentation of videos was fully randomized within both MI and AO runs. Cushions were used to avoid head motion.
Before scanning, participants were familiarized with the experimental conditions and the various different videos. During the rest period, an operator anticipated to the subject the task he/she should perform.
fMRI analysis
Changes in blood oxygenation level dependent (BOLD) contrast associated with the performance of the tasks were assessed on a pixel-by-pixel basis, using the general linear model and the theory of Gaussian fields [31]. FMRI data were analysed using the statistical parametric mapping (SPM8) software. Prior to statistical analysis, all images were realigned to the first one to correct for subject motion, spatially normalized into the standard space of SPM, and smoothed with a 10-mm, 3D-Gaussian filter. None of the study participants were excluded from analysis because of motion artefacts. Linear mixed effects models showed no significant group effect at T0 (p = 0.40) and W4 (p = 0.45) for the maximal translational component (mm) of motion (controls = 1.51 ± 0.55, max 3.14; AOT group = 1.48 ± 0.68, max 3.78; Landscape group = 1.53 ± 0.65, max 3,57). Similarly, no significant group effect at T0 (p = 0.16) and W4 (p = 0.13) was found for the maximal rotational (degree) component (controls = 0.02 ± 0.02, max 0.12; AOT group = 0.02 ± 0.02, max 0.10; Landscape group = 0.02 ± 0.01, max 0.06). In each subject, a first-level design matrix, where motion parameters were used as regressors of non-interest, was built. Then, specific effects were tested applying appropriate linear contrasts (i.e., BOLD changes occurring during MI, AO, and the feet movement were assessed in each subject). Significant hemodynamic changes for each contrast were assessed using t statistical parametric maps (SPMt).
Statistical analysis
Demographic, clinical and cognitive variables at T0 were compared between groups with ANOVA models. In each group, longitudinal linear generalized models for repeated measures were used to assess the change of clinical data and motor functional performance over time. Differential effect of training over time was assessed including into the models the group × time interaction term. SAS Release 9.3, SAS Institute, Cary, NC, USA, was used.
One-sample t tests [p < 0.05, family wise error (FWE) corrected for multiple comparisons] in SPM were used to assess the average fMRI activity during each task in each group. A second-level random effect analysis was performed to assess differences between groups at the T0 visit (controls vs. PD-FoG groups, and Landscape vs. AOT). Intra-group changes over time were evaluated using paired t tests in the ‘Landscape’ group and ‘AOT’ group separately. Multiple linear regression models were used to assess the correlation between fMRI changes and those clinical outcomes showing significant changes at W4 and W8. A mask including sensorimotor, basal ganglia, MNS/fronto-parietal networks was created from the AAL brain atlas [32] and applied to the SPM dataset using WFU Pickatlas [33]; in particular the brainstem, precentral, postcentral and paracentral gyri, supplementary motor area (SMA), inferior frontal gyrus, inferior parietal lobule (angular and supramarginal gyri), superior parietal gyrus, lingual gyrus, fusiform gyrus, basal ganglia (putamen, caudate, globus pallidus, thalamus) and cerebellum bilaterally were included. Significant results within the mask were recognized at p < 0.001 uncorrected at the voxel level but only clusters passing a small volume correction (SVC) for multiple comparisons (10 mm radius, cut off value for significance p < 0.05) were reported.
Results
Baseline characteristics
Patients were randomized into the Landscape (N = 13) and AOT (N = 12) groups (Table 1). Both patient groups were in the moderate stage of the disease and presented with a dopamine-responsive FoG. Compared to healthy controls, PD-FoG patients performed worse on tests assessing visuospatial abilities, problem solving, shifting attention and verbal comprehension (Table 2). Fourteen PD-FoG patients had mild cognitive impairment [34] (6 patients in the Landscape group: 3 single-domain, 3 multi-domain; 8 patients in the AOT group: 6 single-domain, 2 multi-domain). At T0, no difference in demographic, clinical, motor functional and neuropsychological characteristics was detected between PD groups (Tables 1, 2). All participants of the Landscape group completed the study. In the AOT group, one patient was not able to perform W4 and W8 visits, and another subject did not complete the W8 evaluation for personal reasons unrelated to the AOT treatment.
Clinical outcomes
After training (W4), the AOT group showed reduced FoG severity (i.e., FoG-Q and UPDRS II FoG score OFF) and improved UPDRS III ON, and PDQ39, BBS and 10 M-WT scores (Table 1), while the ‘Landscape’ group showed a reduced FoG severity (FoG-Q), decreased PDQ39 score, improved walking abilities and a trend toward an improved BBS score (Table 1). At W8, 10 M-WT improvement was confirmed in both groups, while positive effects on UPDRS III ON, PDQ39 and balance scores were maintained in AOT group only. At W8, AOT group also showed a trend toward a reduced FoG severity. The treatment x time interaction analysis showed an effect on the UPDRS III ON score in the AOT vs. the Landscape group at W4 (p = 0.03), with a trend toward a significant improvement at W8 (p = 0.07).
fMRI results
PD-FoG vs. controls at T0 During the performance of all the three tasks (foot movement, MI, AO), healthy controls showed activation of the primary motor cortex, SMA, inferior dorsolateral frontal cortex, inferior and superior parietal gyri and cerebellum bilaterally (Fig. 1). During the execution of the foot movement task, healthy controls also showed an activity of the postcentral gyrus bilaterally, while during the MI and AO tasks there was also a recruitment of the visual cortex (Fig. 1). PD patients showed a broadly similar but less distributed topographical patterns of activation (Fig. 1). During the foot movement execution task, PD patients showed a reduced activity of the right superior parietal and left supramarginal gyri and an increased activity of the lingual gyrus bilaterally and right cerebellum (crus I) relative to healthy controls (Fig. 2a; Table 3). During MI, PD-FoG patients relative to controls showed a reduced activity of the SMA bilaterally, right paracentral lobule, precentral gyrus [Brodmann area (BA) 6], and supramarginal gyrus, and an enhanced activity of the rolandic operculum bilaterally and left precentral gyrus (BA4) (Fig. 2b; Table 3). During AO, PD-FoG patients showed reduced activity relative to controls of the SMA, precentral gyrus (BA6), and head of the caudate nucleus bilaterally, and left putamen and rolandic operculum, while they showed an enhanced activity of the lingual gyrus bilaterally (Fig. 2c; Table 3).
fMRI patterns of activations on a rendered brain in healthy controls (HC) and Parkinson’s disease patients with freezing of gait (PD-FoG) during the execution of the foot movement task (a), the motor imagery task (b), and the action observation task (c) at baseline (T0) (one-sample t tests, p < 0.05 family wise error corrected for multiple comparisons). Colour bars denote T values
fMRI differences in Parkinson’s disease patients with freezing of gait (PD-FoG) relative to healthy controls (HC) at baseline (T0) during the foot movement task (a), the motor imagery task (b), and the action observation task (c). Results are shown on axial sections of the Montreal Neurological Institute standard brain. Colour bars denote T values
fMRI patterns of changes at Week 4 versus baseline (T0) in the ‘Landscape’ and ‘Action observation training’ (AOT) groups of Parkinson’s disease patients with freezing of gait (PD-FoG) during the foot movement task (a), the motor imagery task (b), and the action observation task (c). Results are shown on axial and sagittal sections of the Montreal Neurological Institute standard brain. Colour bars denote T values
The figure shows the regions where fMRI changes at week 4 (W4) relative to baseline (T0) correlated with clinical changes at W4 and week 8 (W8) in the ‘Action observation training’ (AOT) group of Parkinson’s disease patients with freezing of gait. a Correlation between the increased recruitment of the left inferior frontal gyrus (IFG) during the motor imagery task and the decreased Unified Parkinson’s Disease Rating scale (UPDRS) III score at W4 relative to T0. b Correlation between the increased recruitment of the right precentral gyrus during the foot movement task at W4 relative to T0 and the decreased UPDRS III score at W8 relative to T0. Results are shown on axial sections of the Montreal Neurological Institute standard brain (colour bar denotes T values). L left
AOT vs. Landscape patients at T0. No differences were observed.
Landscape group: W4 vs. T0. After 4 weeks of training, the Landscape group showed a reduced activity of the left postcentral gyrus and right rolandic operculum during the foot movement execution task (Fig. 3a; Table 3), and a reduced recruitment of the left inferior parietal lobule (IPL) and right supramarginal gyrus during the MI task (Fig. 3b; Table 3). No fMRI changes occurred during the AO task.
AOT group: W4 vs. T0. After 4 weeks of training, the AOT group showed: an increased activity of the left superior/inferior parietal and right precentral (BA6) gyri during the foot movement execution task (Fig. 3a; Table 3); an increased recruitment of the left inferior frontal gyrus during the MI task (Fig. 3b); and an increased activity of the right rolandic operculum during the AO task (Fig. 3c; Table 3).
PD-FoG at W4 vs. controls During the foot movement execution task, the Landscape group showed a reduced activity of the cerebellar vermis and an increased activity of the right cerebellar lobule 6 relative to healthy controls, while the AOT group compared with controls showed a reduced activity of the cerebellar vermis and cerebellar lobule 4–5 bilaterally (Supplementary Figure A; Table 4). During MI, PD Landscape patients relative to controls showed a reduced activity of the precentral gyrus bilaterally, left SMA, and right IPL, and an enhanced activity of the right middle occipital gyrus (Supplementary Figure B; Table 4). During MI, PD AOT patients compared with controls showed a reduced activity of the right IPL, and an increased recruitment of the left middle occipital gyrus (Supplementary Figure B; Table 4). No differences were observed between patients and controls during the AO task.
Clinical outcomes vs. fMRI changes (Table 5) In the AOT group, a decreased UPDRS III score at W4 was associated with an increased recruitment of the left inferior frontal gyrus performing the MI task (r = −0.91) (Fig. 4a) at W4 relative to T0. A decreased UPDRS III at W8 in the AOT group correlated with an increased recruitment of the right precentral gyrus during the foot movement task at W4 relative to T0 (r = 0.97) (Fig. 4b). No correlations were found in the Landscape group.
Discussion
The purpose of this study was to test whether AOT is more effective than physical therapy alone in alleviating disease severity and disabling symptoms, including FoG, in PD-FoG patients and to investigate the effects of AOT on brain fMRI activations. The current findings indicate that both the Landscape and AOT groups showed decreased FoG severity, improved walking speed and quality of life after 4 weeks of treatment, but the AOT group showed a further effect on motor (UPDRS III) and balance deficits after training. More importantly, only the AOT group showed a positive effect on motor impairment, walking speed, balance and quality of life after a short-term follow-up (W8), with a trend toward a persisting reduced FoG severity.
The major strength of our study is the use of fMRI to explore the functional correlates of training. PD-FoG patients compared to controls at baseline showed a pattern of altered functional recruitment involving basal ganglia, motor and premotor cortices, MNS and visual cortex during foot movement, MI and AO tasks [35, 36]. After 4 weeks of AOT, PD-FoG patients showed an increased recruitment of premotor and inferior parietal regions (fronto-parietal MNS) during the foot movement execution as well as an increased activation of the inferior frontal cortex during both MI and AO tasks. These findings are confirmed by the comparison between fMRI activity at W4 in PD patients and healthy controls, showing a partial “normalization” of baseline findings. Our findings are in line with those previously obtained during an object manipulation task in patients with chronic stroke after AOT [37]. Importantly, the increased recruitment of the left inferior frontal gyrus during MI correlated with clinical improvement after treatment and the increased activity of motor and premotor areas during the foot movement task correlated with an improvement of UPDRS III score at the short-term follow-up (W8). Taken together, these findings suggest that the enhanced recruitment of the motor network and MNS following AOT is likely to be the functional correlate of clinical and motor improvement in PD-FoG patients.
Several studies have consistently shown that AOT is an effective way to learn or enhance the performance of specific motor skills (observational learning). MNS plays a role in action understanding, imitation learning of novel complex actions, and internal rehearsal of actions [5]. Our fMRI findings showed that the AOT increased the activation of areas of motor network and MNS, such as the premotor cortex, inferior frontal gyrus and IPL, which are known to be engaged both during the observation of actions that are part of the motor repertoire of the observer [38] and also in acquiring new motor skills [6]. Therefore, AOT potentially enhanced motor learning and facilitates the building of a motor memory in our PD-FoG patients [39].
Additionally, we found that AOT is associated with an increased recruitment of regions closely overlapping with the fronto-parietal control network involved in controlled attention and goal-directed processing in response to shifting environmental contingencies [40], suggesting that training intervention enhanced the engagement of externally focused attention. We also observed an increased recruitment of the SPG, a region that is reliably activated during attention-demanding task [40]. Clinically, it is well known that focused attention and external stimuli can help PD patients overcome FoG episodes [3]. A previous fMRI study assessing the neural basis of attention to action compared with simple execution in PD showed that these patients failed to enhance the attention-related activation of SMA and parietal cortex, and that there was a functional disconnection between the prefrontal cortex and the SMA and premotor cortex relative to controls [41]. AOT, cueing actions, may have reinforced the connections between fronto-parietal cortices, thus allowing subjects to better perform actions in context [4]. Another possibility is that FoG is a conflict-resolution deficit evident in situations requiring a response decision and exacerbated by FoG-related executive dysfunction (i.e., the “cognitive model” of FoG) [1, 2]. Executive function deficits have also been shown to worsen implicit motor learning in PD-FoG, suggesting an imbalance between automatic and controlled response selection processes [2, 42]. Previous neuroimaging findings confirmed these models [43]. Using virtual reality and MI paradigms, previous task-based fMRI studies of PD-FoG patients suggested that FoG is associated with a functional decoupling between coordinated motor and cognitive neural networks [36, 44]. In addition, two studies investigated resting state fMRI networks in PD-FoG patients [45, 46] showing an altered functional connectivity in locomotor [46], attention-related fronto-parietal [45], and visual occipito-temporal [45] systems. Enhancing fronto-parietal cortical activations, AOT may have contributed to favour the external and attention-demanding control thus alleviating the severity of gait disturbances in our patients.
In the Landscape group, fMRI showed a different pattern of functional changes after training. These patients showed a practice-related decrease of activation of brain regions implicated in somatosensory and attentional control. One may speculate that this pattern of changes reflects a shift from more controlled to automatic forms of task performance [47]. However, as discussed previously, there are indications that this may be not efficacious over time in PD-FoG patients, as they took advantage of controlled rather than automatic motor behavior in overcoming the FoG episodes [1, 2]. Clinical findings in the Landscape group are in line with this hypothesis since we found some benefits after 4 weeks which are not sustained at the short-term follow-up.
Our study is not without limitations. First, the sample is relatively small. Second, one might question the use of AOT which requires high level cognitive processing in PD-FoG patients as the link between frontal lobe dysfunction and gait disorders is established [1, 2]. However, our patients showed only mild executive deficits relative to controls and were able to follow the training protocol. Certainly, an important gap in our experiment is whether the benefits of AOT are still evident in patients with dementia and later stages of PD. Third, we cannot conclude that AOT is specific for FoG as PD patients without FoG were not included. Fourth, participants’ ability for MI was not tested at study entry using appropriate questionnaire. Fifth, since walking is not feasible during fMRI, we assessed brain activity while subjects observe or image walking and during foot movement execution task. However, walking requires an integrated cortical, subcortical, and spinal sensorimotor network, which can be missed, at least partially, by our tasks. Novel computer paradigms, including virtual reality, can improve the capacity of fMRI to identify the neural correlates of gait rehabilitation. Finally, in the present study the training was applied for a relatively short time period (4 weeks) with a relatively short-term follow up (additional 4 weeks). Future studies should investigate the effects of longer therapy in larger patient populations.
In conclusion, this study provides evidence that 4-week AOT is a non-invasive, non-pharmacological and cost-effective treatment with a more lasting effect in improving motor function, gait (including FoG) and quality of life in PD-FoG patients relative to physical therapy alone. Using fMRI, we also showed that AOT-related performance gains are associated with an increased recruitment of motor regions and fronto-parietal MNS. It is likely that future development of AOT will benefit from knowing how the brain changes with therapy. Further investigations are needed to identify the long term effects of the AOT in PD.
References
Nieuwboer A, Giladi N (2013) Characterizing freezing of gait in Parkinson’s disease: models of an episodic phenomenon. Mov Disord 28:1509–1519
Vandenbossche J, Deroost N, Soetens E, Coomans D, Spildooren J, Vercruysse S, Nieuwboer A, Kerckhofs E (2012) Freezing of gait in Parkinson’s disease: disturbances in automaticity and control. Front Hum Neurosci 6:356
Nonnekes J, Snijders AH, Nutt JG, Deuschl G, Giladi N, Bloem BR (2015) Freezing of gait: a practical approach to management. Lancet Neurol 14:768–778
Buccino G (2014) Action observation treatment: a novel tool in neurorehabilitation. Philos Trans R Soc Lond B Biol Sci 369:20130185
Rizzolatti G, Craighero L (2004) The mirror-neuron system. Annu Rev Neurosci 27:169–192
Stefan K, Cohen LG, Duque J, Mazzocchio R, Celnik P, Sawaki L, Ungerleider L, Classen J (2005) Formation of a motor memory by action observation. J Neurosci 25:9339–9346
Buccino G, Gatti R, Giusti MC, Negrotti A, Rossi A, Calzetti S, Cappa SF (2011) Action observation treatment improves autonomy in daily activities in Parkinson’s disease patients: results from a pilot study. Mov Disord 26:1963–1964
Pelosin E, Bove M, Ruggeri P, Avanzino L, Abbruzzese G (2013) Reduction of bradykinesia of finger movements by a single session of action observation in Parkinson disease. Neurorehabil Neural Repair 27:552–560
Pelosin E, Avanzino L, Bove M, Stramesi P, Nieuwboer A, Abbruzzese G (2010) Action observation improves freezing of gait in patients with Parkinson’s disease. Neurorehabil Neural Repair 24:746–752
Giladi N, Shabtai H, Simon ES, Biran S, Tal J, Korczyn AD (2000) Construction of freezing of gait questionnaire for patients with Parkinsonism. Parkinsonism Relat Disord 6:165–170
Hoehn MM, Yahr MD (1967) Parkinsonism: onset, progression and mortality. Neurology 17:427–442
Folstein MF, Folstein SE, McHugh PR (1975) “Mini-mental state”. A practical method for grading the cognitive state of patients for the clinician. J Psychiatr Res 12:189–198
Beck AT, Ward CH, Mendelson M, Mock J, Erbaugh J (1961) An inventory for measuring depression. Arch Gen Psychiatry 4:561–571
Orsini A, Grossi D, Capitani E, Laiacona M, Papagno C, Vallar G (1987) Verbal and spatial immediate memory span: normative data from 1355 adults and 1112 children. Ital J Neurol Sci 8:539–548
Carlesimo GA, Caltagirone C, Gainotti G (1996) The Mental Deterioration Battery: normative data, diagnostic reliability and qualitative analyses of cognitive impairment. The Group for the Standardization of the Mental Deterioration Battery. Eur Neurol 36:378–384
Caffarra P, Vezzadini G, Dieci F, Zonato F, Venneri A (2002) Rey-Osterrieth complex figure: normative values in an Italian population sample. Neurol Sci 22:443–447
Novelli G, Papagno C, Capitani E, Laiacona N, Vallar G, Cappa SF (1986) Tre test clinici di ricerca e produzione lessicale. Taratura su soggetti normali. Arch Psicol Neurol Psichiatr 47:477–506
Abrahams S, Leigh PN, Harvey A, Vythelingum GN, Grise D, Goldstein LH (2000) Verbal fluency and executive dysfunction in amyotrophic lateral sclerosis (ALS). Neuropsychologia 38:734–747
Manos PJ (1999) Ten-point clock test sensitivity for Alzheimer’s disease in patients with MMSE scores greater than 23. Int J Geriatr Psychiatry 14:454–458
Caffarra P, Vezzadini G, Dieci F, Zonato F, Venneri A (2004) Modified card sorting test: normative data. J Clin Exp Neuropsychol 26:246–250
Monaco M, Costa A, Caltagirone C, Carlesimo GA (2013) Forward and backward span for verbal and visuo-spatial data: standardization and normative data from an Italian adult population. Neurol Sci 34:749–754
Spinnler H, Tognoni G (1987) Standardizzazione e taratura italiana di test neuropsicologici. Ital J Neurol Sci 6:1–120
Giovagnoli AR, Del Pesce M, Mascheroni S, Simoncelli M, Laiacona M, Capitani E (1996) Trail making test: normative values from 287 normal adult controls. Ital J Neurol Sci 17:305–309
Miceli G, Laudanna A, Burani C, Capasso R (1994) Batteria per l’Analisi del Deficit Afasico. B.A.D.A. [B.A.D.A. A battery for the assessment of aphasic disorders]. CEPSAG, Roma
De Renzi E, Faglioni P (1978) Normative data and screening power of a shortened version of the Token Test. Cortex 14:41–49
Mioshi E, Dawson K, Mitchell J, Arnold R, Hodges JR (2006) The Addenbrooke’s Cognitive Examination Revised (ACE-R): a brief cognitive test battery for dementia screening. Int J Geriatr Psychiatry 21:1078–1085
Fahn S, Elton RL, Committee motUD (1987) Unified Parkinson’s disease rating scale. In: Fahn S, Marsden CD, Goldstein M, Calne DB (eds) Recent developments in Parkinson’s disease II. MacMillan, New York, pp 153–163
Peto V, Jenkinson C, Fitzpatrick R, Greenhall R (1995) The development and validation of a short measure of functioning and well being for individuals with Parkinson’s disease. Qual Life Res 4:241–248
Franchignoni F, Velozo CA (2005) Use of the Berg Balance Scale in rehabilitation evaluation of patients with Parkinson’s disease. Arch Phys Med Rehabil 86:2225–2226 (author reply 2226)
Johnston M, de Morton N, Harding K, Taylor N (2013) Measuring mobility in patients living in the community with Parkinson disease. NeuroRehabilitation 32:957–966
Worsley KJ, Friston KJ (1995) Analysis of fMRI time-series revisited–again. Neuroimage 2:173–181
Tzourio-Mazoyer N, Landeau B, Papathanassiou D, Crivello F, Etard O, Delcroix N, Mazoyer B, Joliot M (2002) Automated anatomical labeling of activations in SPM using a macroscopic anatomical parcellation of the MNI MRI single-subject brain. Neuroimage 15:273–289
Maldjian JA, Laurienti PJ, Kraft RA, Burdette JH (2003) An automated method for neuroanatomic and cytoarchitectonic atlas-based interrogation of fMRI data sets. Neuroimage 19:1233–1239
Litvan I, Goldman JG, Troster AI, Schmand BA, Weintraub D, Petersen RC, Mollenhauer B, Adler CH, Marder K, Williams-Gray CH, Aarsland D, Kulisevsky J, Rodriguez-Oroz MC, Burn DJ, Barker RA, Emre M (2012) Diagnostic criteria for mild cognitive impairment in Parkinson’s disease: Movement Disorder Society Task Force guidelines. Mov Disord 27:349–356
Herz DM, Eickhoff SB, Lokkegaard A, Siebner HR (2014) Functional neuroimaging of motor control in Parkinson’s disease: a meta-analysis. Hum Brain Mapp 35:3227–3237
Snijders AH, Leunissen I, Bakker M, Overeem S, Helmich RC, Bloem BR, Toni I (2011) Gait-related cerebral alterations in patients with Parkinson’s disease with freezing of gait. Brain 134:59–72
Ertelt D, Small S, Solodkin A, Dettmers C, McNamara A, Binkofski F, Buccino G (2007) Action observation has a positive impact on rehabilitation of motor deficits after stroke. Neuroimage 36(Suppl 2):T164–173
Buccino G, Vogt S, Ritzl A, Fink GR, Zilles K, Freund HJ, Rizzolatti G (2004) Neural circuits underlying imitation learning of hand actions: an event-related fMRI study. Neuron 42:323–334
Gatti R, Tettamanti A, Gough PM, Riboldi E, Marinoni L, Buccino G (2013) Action observation versus motor imagery in learning a complex motor task: a short review of literature and a kinematics study. Neurosci Lett 540:37–42
Fox MD, Snyder AZ, Vincent JL, Corbetta M, Van Essen DC, Raichle ME (2005) The human brain is intrinsically organized into dynamic, anticorrelated functional networks. Proc Natl Acad Sci USA 102:9673–9678
Rowe J, Stephan KE, Friston K, Frackowiak R, Lees A, Passingham R (2002) Attention to action in Parkinson’s disease: impaired effective connectivity among frontal cortical regions. Brain 125:276–289
Vercruysse S, Gilat M, Shine JM, Heremans E, Lewis S, Nieuwboer A (2014) Freezing beyond gait in Parkinson’s disease: a review of current neurobehavioral evidence. Neurosci Biobehav Rev 43:213–227
Fasano A, Herman T, Tessitore A, Strafella AP, Bohnen NI (2015) Neuroimaging of Freezing of Gait. J Parkinsons Dis 5:241–254
Shine JM, Matar E, Ward PB, Frank MJ, Moustafa AA, Pearson M, Naismith SL, Lewis SJ (2013) Freezing of gait in Parkinson’s disease is associated with functional decoupling between the cognitive control network and the basal ganglia. Brain 136:3671–3681
Tessitore A, Amboni M, Esposito F, Russo A, Picillo M, Marcuccio L, Pellecchia MT, Vitale C, Cirillo M, Tedeschi G, Barone P (2012) Resting-state brain connectivity in patients with Parkinson’s disease and freezing of gait. Parkinsonism Relat Disord 18:781–787
Fling BW, Cohen RG, Mancini M, Carpenter SD, Fair DA, Nutt JG, Horak FB (2014) Functional reorganization of the locomotor network in Parkinson patients with freezing of gait. PLoS One 9:e100291
Wu T, Hallett M (2005) A functional MRI study of automatic movements in patients with Parkinson’s disease. Brain 128:2250–2259
Acknowledgments
We thank Dr. Elisa Pelosin for providing us with the video clips used during the training sessions. This study was partially supported by a grant from the Jacques and Gloria Gossweiler Foundation.
Author information
Authors and Affiliations
Corresponding author
Ethics declarations
Conflicts of interest
F Agosta serves on the editorial board of the Journal of Neurology; has received speaker honoraria from EXCEMED– Excellence in Medical Education; and receives research supports from the Italian Ministry of Health, AriSLA (Fondazione Italiana di Ricerca per la SLA), and the European Research Council. R. Gatti, E. Sarasso, M.A. Volonté, E. Canu, A. Meani, L. Sarro, M. Copetti, E. Cattrysse, E. Kerckhofs, and A. Falini report no disclosures. G. Comi has received compensation for consulting services and/or speaking activities from Novartis, Teva Pharmaceutical Ind., Sanofi, Genzyme, Merck Serono, Biogen, Bayer, Actelion, Serono Symposia International Foundation, Almirall, Chugai and Receptos. M. Filippi is Editor-in-Chief of Journal of Neurology; serves on the scientific advisory board of Teva Pharmaceutical Industries; has received compensation for consulting services and/or speaking activities from Biogen Idec, Excemed, Novartis, and Teva Pharmaceutical Industries; and receives research support from Biogen Idec, Teva Pharmaceutical Industries, Novartis, Italian Ministry of Health, Fondazione Italiana Sclerosi Multipla, Cure PSP, Alzheimer’s Drug Discovery Foundation (ADDF), the Jacques and Gloria Gossweiler Foundation (Switzerland), and ARiSLA (Fondazione Italiana di Ricerca per la SLA).
Additional information
F. Agosta and R. Gatti contributed equally to this work.
Electronic supplementary material
Below is the link to the electronic supplementary material.
Rights and permissions
About this article
Cite this article
Agosta, F., Gatti, R., Sarasso, E. et al. Brain plasticity in Parkinson’s disease with freezing of gait induced by action observation training. J Neurol 264, 88–101 (2017). https://doi.org/10.1007/s00415-016-8309-7
Received:
Revised:
Accepted:
Published:
Issue Date:
DOI: https://doi.org/10.1007/s00415-016-8309-7