Abstract
The trafficking of cytotoxic CD8+ T lymphocytes across the lining of the cerebral vasculature is key to the onset of the chronic neuro-inflammatory disorder multiple sclerosis. However, the mechanisms controlling their final transmigration across the brain endothelium remain unknown. Here, we describe that CD8+ T lymphocyte trafficking into the brain is dependent on the activity of the brain endothelial adenosine triphosphate-binding cassette transporter P-glycoprotein. Silencing P-glycoprotein activity selectively reduced the trafficking of CD8+ T cells across the brain endothelium in vitro as well as in vivo. In response to formation of the T cell–endothelial synapse, P-glycoprotein was found to regulate secretion of endothelial (C–C motif) ligand 2 (CCL2), a chemokine that mediates CD8+ T cell migration in vitro. Notably, CCL2 levels were significantly enhanced in microvessels isolated from human multiple sclerosis lesions in comparison with non-neurological controls. Endothelial cell-specific elimination of CCL2 in mice subjected to experimental autoimmune encephalomyelitis also significantly diminished the accumulation of CD8+ T cells compared to wild-type animals. Collectively, these results highlight a novel (patho)physiological role for P-glycoprotein in CD8+ T cell trafficking into the central nervous system during neuro-inflammation and illustrate CCL2 secretion as a potential link in this mechanism.
Similar content being viewed by others
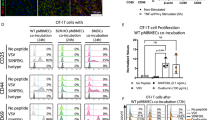
Avoid common mistakes on your manuscript.
Introduction
Leukocyte migration across the endothelium of the brain vasculature plays a key role in the onset of the chronic inflammatory disorder multiple sclerosis. Upon the formation of perivascular infiltrates, clinical symptoms arise leading to severe neurological deficits. Autoreactive T lymphocytes play a critical role in the disease process [12], both in multiple sclerosis as well as its animal model experimental autoimmune encephalomyelitis (EAE) [15]. CD4+ T cells are thought to be essential for the initiation and progression of the disease, whereas CD8+ T cells, which clonally expand within the inflamed CNS, are believed to play a key role in the progression of multiple sclerosis [42].
While the molecular interactions that underlie the migration of CD4+ T cells across the brain endothelium are well-defined, the mechanisms regulating CD8+ T cells entry into the central nervous system remain poorly described. In general, the sequential steps in the diapedesis of lymphocytes include tethering, rolling, capture, firm adhesion and finally migration across the endothelial layer [38]. The continuous cross-talk between immune cells and brain endothelium is an essential element in mediating immune cell migration into the central nervous system parenchyma [14]. Endothelial cells actively participate in this process by expressing adhesion molecules and producing chemokines [2, 10]. Upon the adhesion of lymphocytes, endothelial cells form ring-like membrane structures, so-called migratory cups, which stabilize the leukocytes onto the endothelial cell surface [3] and facilitate their migration. Although great progress has been made in the identification of the molecular interactions that mediate leukocyte transmigration [25, 31], it is becoming increasingly clear that in order to finalize this process, T lymphocytes require additional activation through chemokines presented on or present within the endothelium [35]. However, the precise mechanism that underlies this signaling of the endothelium to the adhered T cell remains to be elucidated.
We here provide evidence that the brain endothelial ATP-binding cassette transporter P-glycoprotein is critical for the release of brain endothelial chemokines, thereby providing a means to control the migration of CD8+ T cells in particular. ATP-binding cassette transporters are highly expressed at the cerebral endothelium, where they actively remove toxic compounds from the brain at the cost of ATP hydrolysis [26]. Although P-glycoprotein was originally described as a prototypic transporter that mediates multi-drug resistance of tumor cells [17], most recent work suggests that P-glycoprotein may additionally mediate immune responses possibly through its capacity to mediate secretion of cytokines, chemokines and bioactive lipids [18–21, 39].
Here, we show that silencing of P-glycoprotein activity in brain endothelial cells selectively impairs the adhesion of CD8+ T cells to intercellular adhesion molecule-1 as well as their transendothelial migration through the secretion of endothelial (C–C motif) ligand 2 (CCL2), a critical agent for CD8+ T cell migration. Notably, characterization of human brain microvessels freshly isolated from post-mortem tissue of multiple sclerosis patients revealed high levels of CCL2 transcripts, reinforcing a role for endothelial-derived CCL2 in human neuro-inflammatory disease. Finally, we were able to show that endothelial targeted CCL2 conditional knockout mice that were subjected to EAE contained significantly reduced levels of infiltrated CD8+ T cells, highlighting the importance of secreted CCL2 in regulating CD8+ T lymphocytes entry into the brain under pathological conditions.
Materials and methods
Cell isolation and culture
The immortalized human brain endothelial cell line hCMEC/D3, which establishes the key features of brain endothelium, was cultured as described [43]. Human CD4+ and CD8+ T cells were purified from peripheral blood mononuclear cells using MACS® magnetic cell sorting kit (Miltenyi Biotec) according to manufacturer’s instructions. CD4+ and CD8+ T cell purity was >96 % as assessed by flow cytometry performed (FACSCalibur™) using CELLQuestTM software (BD Biosciences). Isolated T cells were cultured for 48 h before experiments. Effector T cells were generated by adding IL-2 (10 ng/ml) and phytohemagglutinin (1 μg/ml) during 48 h.
Docking structure imaging
hCMEC/D3 cells were cultured in μSlide VI coated with collagen (Ibidi GmbH) to confluence. CD4+ or CD8+ T cells were labeled with a fluorescent membrane dye PKH26 (Sigma-Aldrich) according to manufacturer’s instructions and added to the endothelial slides for 30 min. Non-adherent cells were removed and slides were fixed with 4 % paraformaldehyde in phosphate buffered saline for 15 min and blocked with 5 % normal donkey serum in phosphate buffered saline with 0.3 % Triton X-100 for 15 min. Cells were incubated with anti ICAM-1 (Santa Cruz) and anti-P-glycoprotein (15D3, BD Biosciences) antibodies (Electronic Supplementary Material 1) and relevant secondary antibodies were used. After each step, the slides were washed three times with phosphate buffered saline. Coverslips were mounted with Vectashield (Vector Labs) or Mowiol 488 reagent (Calbiochem) on microscope slides. Imaging was performed using a confocal laser scanning microscope (Leica TCS SP2).
Silencing P-glycoprotein and qRT-PCR
Plasmids encoding specific P-glycoprotein shRNA or non-targeting control shRNA (NTC-shRNA) were purchased from Sigma. Messenger RNA was isolated from hCMEC/D3 cells using an mRNA capture kit (Roche). cDNA was synthesized with the Reverse Transcription System kit (Promega) and real-time PCR was performed as described previously [19]. All primer sequences are listed in Electronic Supplementary Material 2 and expression levels of transcripts obtained with qRT-PCR were normalized to GAPDH expression levels.
Flow adhesion and migration assays
NTC-shRNA or P-glycoprotein shRNA-transduced hCMEC/D3 cells were cultured to confluence in collagen-coated μSlide VI flow chambers (Ibidi GmbH). Cells were treated with 5 ng/ml TNF-α (Preprotech) for 24 h. Cells were mounted onto the microscope stage using a POC-mini chamber system (LaCon) and connected to a perfusion pump. Using physiological flow conditions (flow speed was 500 μl/min, correlating to 0.8 dyne/cm2), 5 × 105 T cells/ml were perfused over the endothelial cells, followed by 20 min of fluid-flow. Transendothelial migration was characterized by the change in appearance of T cells from bright to dim. Migrated cells were quantified by counting per field-of-view. From one experiment, five fields were analyzed. Movies were generated using VirtualDub.
ICAM-1 binding assay
ICAM-1 binding assays were performed as described previously [4] and fluorescence was quantified using a cytofluorometer (Bio-Rad). Results are expressed as the mean percentage of adhesion of triplicate wells.
Electron microscopy
hCMEC/D3 cells were grown to confluence on fibronectin-coated Thermanox slides (Nunc) and fixed with 1 % paraformaldehyde overnight, then embedded in gelatine, infiltrated with sucrose and frozen in liquid nitrogen as described [36]. Ultra-thin sections (60–70 nm) were prepared using a cryotome (UC6; Leica) and labeled with an anti-P-glycoprotein antibody (C219, Alexis) in 1 % bovine serum albumin (Aurion) in phosphate buffered saline for 1 h. Visualization was performed with Protein A gold 10 nm (UMC). Sections were post-stained with uranyl acetate and uranyl oxalate. The sections were examined at 80 keV using a Technai 12 EM (FEI Co), equipped with a side-mounted Megaview II camera (SIS). The specificity of the electron microscopy was controlled by using an isotype control as well as leaving out the primary antibody, which in both cases did not show any gold grains.
Isolation of microvessels from control or multiple sclerosis brains
Brain tissue (eight non-neurological controls and eight multiple sclerosis patients) was obtained from The Netherlands Brain Bank from donors with written consent. Brain microvessels were isolated from non-neurological patient tissue, normal-appearing white matter (NAWM) and multiple sclerosis lesions from post-mortem multiple sclerosis patients and validated by qRT-PCR as described before [34].
EAE induction in mice
Female mice with CCL2-deficient endothelial cells were developed as described previously [13] and together with their littermate controls (C57BL/6 background) were used at the age of 8–10 weeks. All mice were kept under specific pathogen-free conditions and used in accordance with local animal experimentation guidelines. EAE was induced in C57BL/6 mice as described previously [22].
Histology and immunohistochemistry
Brains of the killed animals were dissected, snap-frozen in liquid nitrogen, and stored at −80 °C. For immunohistochemistry, cryosections were fixed in ice-cold acetone for 10 min and blocked with normal mouse serum prior to antibody staining. Immunofluorescence staining was performed in phosphate buffered saline, supplemented with 0.1 % bovine serum albumin as previously described [18], and antibodies are described in Electronic Supplementary Material 1. Immunofluorescent sections were enclosed in Vinol (Air Products) supplemented with DAPI (Invitrogen) and analyzed on a Leica DM6000 fluorescence microscope (Leica Microsystems), equipped with LAS AF (Leica) software.
3-D rendering of extravasating CD8+ T cells during EAE
Tissue processing was performed as described recently [32]. In brief, at day 16 of EAE, spinal cords were harvested and cryoprotected in 30 % sucrose overnight prior to cryomatrix embedding. Serial cryosections of 60 μm thickness were obtained from the thoraco-lumbar spinal cord, and incubated with rabbit anti-mouse Laminin 1 (CLPR245679; Cedarlane) antibody to highlight the microvascular basement membrane and with CD8β-FITC antibody for CD8+ T cells. DRAQ5 (DR51000; Biostatus Ltd.) was utilized for nuclear staining. For visualization of the CD8+ T cells in inflamed venules, confocal z-stacks were acquired as described [32] and imported into Bitplane IMARIS suite version 7.1x 64 software (Bitplane Inc.) and a volume-rendered image was obtained in maximum-intensity projection mode. Individual color channels in the volume-rendered image were subjected to iso-surface rendering to enhance visualization of spatial architecture around venules and relative localization of the CD8+ T cells.
Relative quantification of immunohistochemistry and 3-D rendering
Volume-rendered images were fed into ImageJ software (NIH) and a microvascular contour was created manually by cursoring out the inflamed venules of interest. Respective areas representing the microvessel segment (# of pixels occupied by the microvessel) and associated CD8+ T cell population (# of green pixels) were determined and the percentage vessel area occupied by CD8+ T cell aggregates calculated as follows: (# of green pixels)/(# of pixels occupied by the microvessel) × 100. For immunohistochemistry, a similar approach (using ImageJ) was applied on multiple EAE lesions from 4 WT and 4 mdr1a/1b−/− animals by separating the green (CD4+ or CD8+ T cells) from the blue (CD45+ cells) channel. These images were also subjected to intensity-threshold adjustment and the percentage of CD45+CD4+ or CD45+CD8+ cells was determined.
Statistical analysis
All data were analyzed statistically by means of analysis of variance (ANOVA) and Student’s t test. Statistical significance was defined as *p < 0.05, **p < 0.01, ***p < 0.001.
Results
P-glycoprotein regulates CD8+ T cell migration across an in vitro model of the blood–brain barrier
To determine the potential role for P-glycoprotein in the transendothelial migration of CD4+ and CD8+ T cells under flow conditions, we generated human brain endothelial cells that lack P-glycoprotein activity using a lentiviral approach, resulting in over 80 % reduction of P-glycoprotein expression (Electronic Supplementary Material 3a) and function (Electronic Supplementary Material 3b) compared to non-targeting control (NTC) shRNA-transfected cells. Silencing P-glycoprotein activity significantly reduced transendothelial migration (Fig. 1a) and adhesion (Fig. 1b) of both T cell subsets across inflamed (TNF-α-stimulated) brain endothelium (Fig. 1a–c). The percentage of CD8+ T cell that adhere to the endothelial cells and subsequently migrate was significantly impaired up to 50 % under P-glycoprotein-silenced conditions (Fig. 1c), whereas the migration capacity of CD4+ T cells after firm adhesion was independent of P-glycoprotein function (Fig. 1c). Upon adhesion, CD8+ T cells appeared immobilized on the endothelial cells (Electronic Supplementary Material 4), whereas CD4+ T cells still displayed crawling behavior followed by transmigration (Electronic Supplementary Material 5). Importantly, silencing P-glycoprotein did not affect the mRNA and protein expression levels of intercellular adhesion molecule-1 (Electronic Supplementary Material 3c, d) and vascular cell adhesion molecule-1 (Electronic Supplementary Material 3d, e). Collectively, these results suggest that P-glycoprotein activity is particularly required to direct CD8+ T cell trafficking upon their firm adhesion to brain endothelial cells.
P-glycoprotein mediates CD4+ and CD8+ T cell adhesion and migration under flow conditions Non-targeting control (NTC; black bars) or P-glycoprotein shRNA (grey bars) transfected cells were grown in IBIDI slides and were incubated after 2 days with TNF-α for 24 h. Next, freshly isolated primary human CD4+ and CD8+ T cells were added to visualize and quantify their migration (a) and adhesion (b) capacity. Moreover, the number of CD4+ and CD8+ T cells that started to migrate after their initial arrest on the endothelial cells was quantified (c). Experiments were performed in triplicate using three different human T cell donors and were presented as the percentage of migration/adhesion compared to NTC-transfected cells ± SEM. **p < 0.01, ***p < 0.001 as determined by Student’s t test
mdr1a/1b−/− mice lack CD8+ T cell infiltrates during EAE
As reported earlier, mdr1a/b−/− mice showed significantly reduced clinical signs of EAE [18], which coincided with reduced CD45+CD3+ T cell infiltrates in EAE lesions. To extrapolate our in vitro results (Fig. 1) to an in vivo situation, we analyzed and quantified the presence of disease causing parenchymal CD45+CD4+ as well as CD8+ T cells in wild type and mdr1a/b−/− mice during EAE. Notably, CD45+CD8+ T cell infiltrates were almost completely absent from EAE lesions in mdr1a/1b−/− compared to their wild-type littermates, whereas the percentage of CD45+CD4+ T cell infiltrates was comparable between wild type and mdr1a/1b−/− EAE mice (Fig. 2a–d, quantification 2e). No changes were found in the percentage of circulating peripheral CD4+ T cells or CD8+ T cells in control (CFA: complete Freund’s adjuvant) and EAE animals from mdr1a/1b−/− or wild-type mice (Fig. 2f, g) and both T cell subsets respond equally well to polyclonal stimuli [18], indicating that P-glycoprotein predominantly affects CD8+ T cell trafficking across brain endothelial cells in vitro but also in vivo during neuro-inflammation.
Lack of CD8+ T cell infiltrates in EAE lesions of mdr1a/1b−/− mice. Brains were isolated from EAE mice and the cerebellum white matter was analyzed for the infiltration of CD4+ T cells (green; a, b) or CD8+ T cells (green; c, d). Slides were counterstained for the presence of CD45+ infiltrating cells (blue) and laminin positive (red) basement membranes around vessels in WT (a, c) or mdr1a/1b−/− (b, d) mice. Images represent representative tissues from four mice per group. Magnification ×200. e Quantification of immunohistochemistry using ImageJ software on various EAE lesions of 4 mice per group. Data are presented as the percentage of CD4+ and CD8+ T cells of the total number of CD45+ infiltrates per EAE lesion and indicate a lack of CD8+ T cells in mdr1a/1b−/− animals compared to WT during EAE. f Peripheral blood mononuclear cell analysis by fluorescence-activated cell sorting revealed no differences in the number of circulating CD45+ CD4+ or g CD45+ CD8+ T cells in wild type (WT; black bars) or mdr1a/1b−/− (grey bars) mice under control (CFA complete Freund’s adjuvant) or EAE conditions. Experiments were performed in triplicate using four mice per group in two independent experiments and were presented as the mean ± SEM. **p < 0.01, ***p < 0.001 as determined by Student’s t test
P-glycoprotein localizes in endothelial migratory cups upon T lymphocyte attachment
Binding of both effector CD4+ (Fig. 3a) and CD8+ (Fig. 3b) T lymphocytes to inflamed brain endothelial cells resulted in the recruitment of P-glycoprotein into the migratory cups, as indicated by the partly overlapping expression with an established marker intercellular adhesion molecule-1. However, silencing P-glycoprotein on the endothelium revealed no differences in docking structure formation upon T cell attachment (Electronic Supplementary Material 6), thereby excluding a potential role for P-glycoprotein in migratory cup formation.
Co-localization of P-glycoprotein and ICAM-1 in endothelial migratory cups upon T cell attachment. hCMEC/D3 cells were stimulated with TNF-α for 24 h. After that, freshly isolated primary human CD4+ (a) or CD8+ (b) T cells labeled with the membrane dye PKH (in red) were added and subsequently co-cultured with endothelial cells for 1 h. Next, co-cultures were fixed with paraformaldehyde and stained for P-glycoprotein (in green) and intercellular cell adhesion molecule-1 (ICAM-1 in blue), indicating a co-localization of P-glycoprotein with ICAM-1 in endothelial migratory cups upon CD4+ or CD8+ T cell attachment. Scale bar 10 μm
Further analysis using electron microscopy revealed that P-glycoprotein localized at the plasma membrane of the endothelial cells at the contact sites of CD8+ T lymphocytes (Fig. 4b; quantification Fig. 4c) and CD4+ T lymphocytes (data not shown), whereas in control endothelium its plasma membrane localization is less predominant (Fig. 4a). Notably, P-glycoprotein expression is also observed in T cells (Fig. 4b), although P-glycoprotein activity is low in either CD4+ or CD8+ T lymphocytes compared to brain endothelial cells (Electronic Supplementary Material 7). Together, these results indicate that P-glycoprotein becomes enriched in endothelial migratory cups upon T cell attachment, where it may locally exert its regulatory role in CD8+ T cell transendothelial migration.
Redistribution of P-glycoprotein to endothelial plasma membrane upon T cell adhesion. hCMEC/D3 cells were grown on transwell polyester membrane inserts. Upon confluency, freshly isolated primary human CD8+ T cells were added and subsequently co-cultured with endothelial cells for 1 h. Next, co-cultures were fixed and prepared as described in “Materials and methods”. Ultra-thin sections were immune gold labeled for P-glycoprotein and subsequently the labeled sections were analyzed by electron microscopy, indicating a predominant cytoplasmic localization of P-glycoprotein (as indicated by arrows) in endothelial cells (a; insert). Upon T cell attachment (b; insert), P-glycoprotein redistributes to the plasma membrane (as indicated by arrows). (c) Quantification of P-glycoprotein localization on endothelial cells (EC) in the presence or absence of T cells. For this, P-glycoprotein-positive particles in 20 endothelial cells and 20 endothelial cells that contain adhering T cells were counted by two independent researchers, *p < 0.05 as determined by Student’s t test. N nucleus
P-glycoprotein regulates CD8+ T cell migration via CCL2 secretion
To study if mediators effluxed by P-glycoprotein induce the migration of CD8+ T cells, we harvested supernatants from control or P-glycoprotein-silenced endothelial cells under inflammatory or control conditions, and determined their ability to affect CD4+ and CD8+ T cell adhesion to intercellular adhesion molecule-1, a key player in transendothelial migration. As shown in Fig. 5a, b, conditioned media from TNF-α-stimulated endothelial cells significantly induced the adhesion of both CD4+ and CD8+ T lymphocytes to ICAM-1 compared to conditioned media from untreated endothelial cells. Induced lymphocyte adhesion to intercellular adhesion molecule-1 was inhibited in the presence of pertussis toxin (data not shown), indicating an important role for G-protein-coupled receptors, such as chemokines receptors, herein. Conditioned medium from TNF-α-treated endothelial cells lacking P-glycoprotein activity failed to induce CD8+ T cell adhesion to intercellular adhesion molecule-1, whereas the induction of CD4+ T cell adhesion to intercellular adhesion molecule-1 was largely unaffected (Fig. 5a, b). These results indicate that P-glycoprotein is involved in the secretion of soluble factors from inflamed endothelium that selectively affect CD8+ T cell adhesion to intercellular adhesion molecule-1.
P-glycoprotein regulates CD8+ T cell migration via CCL2 secretion. Supernatants from control or TNF-α-treated non-targeting control or P-glycoprotein shRNA-transfected cells were harvested and subsequently exposed to naïve CD4+ (a) and CD8+ (b) T cells for 30 min after which their adhesive capacity to intercellular adhesion molecule-1 was tested. Phorbol 12-myristate 13-acetate (PMA) was used as a positive control. Next, the presence of CCL2 was determined in cell supernatants by enzyme-linked immunosorbent assay (c) and CCL2 transcripts (d) were determined by real-time quantitative PCR (qRT-PCR) and presented as relative expression (FI fold induction) compared to GAPDH. The capacity of the above-mentioned conditioned media to attract T cells was tested in transwell migration experiments (e). Conditioned media was added to the lower chamber in the presence or absence of exogenous CCL2 (10 ng/ml). f Identification of endothelial purity of isolated human brain microvessels from non-neurological control brain tissues (n = 6) was performed by analyzing GFAPa (astrocytes), PDGFRβ (pericytes), zona-occludens-1 (ZO-1), claudin-5 and P-glycoprotein transcripts by qRT-PCR and presented them as relative expression compared to GAPDH. g CCL2 transcripts were detected by qRT-PCR on human brain microvessel fractions of control, normal-appearing white matter (NAWM) or multiple sclerosis (MS) lesion brain tissue (n = 6, respectively) and presented as relative expression (FI fold induction) compared to GAPDH. Experiments were performed in triplicate using three different human T cell donors (a, b, e) and were presented as the number of adhered T cells (a, b) or as the percentage of CD4+ T cell migration across NTC-transfected cells ± SEM. *p < 0.05, **p < 0.01, ***p < 0.001 as determined by Student’s t test
A potent inducer of leukocyte migration into the brain is the chemokine (C–C motif) ligand 2 (CCL2) [27]. Activated CD4+ and CD8+ T cells express a differential expression pattern of chemokine receptors, of which CCR2 is predominantly expressed by effector CD8+ T cells [5]. In that way, CD8+ T cells may more selectively respond to CCL2 compared to CD4+ T cells. To assess whether endothelial P-glycoprotein is able to mediate the efflux of CCL2 by inflamed brain endothelial cells, we determined the presence of CCL2 in the above-described conditioned media. TNF-α treatment highly induced CCL2 secretion by brain endothelial cells, which was abrogated upon P-glycoprotein silencing (Fig. 5c). In contrast, expression of mRNA encoding for CCL2 in both endothelial cell populations remained unaffected, indicating that CCL2 secretion but not its synthesis is mediated by P-glycoprotein (Fig. 5d). P-glycoprotein preferentially affected the secretion of CCL2 by inflamed endothelium, since the secretion of the pro-inflammatory cytokine IL-1β was highly induced in the absence of P-glycoprotein (Electronic Supplementary Material 8). To demonstrate that CCL2 affects CD8+ T cell migration and to dissect the role of P-glycoprotein in this process, in vitro transwell migration assays were performed using conditioned media from inflammatory endothelial cells that lack P-glycoprotein activity in the presence or absence of CCL2. Using this setup, we found that both CD4+ and CD8+ T cell migration towards the conditioned media was significantly inhibited upon brain endothelial P-glycoprotein silencing (Fig. 5e). However, the addition of CCL2 to the conditioned media restored the migratory capacity of CD8+ T cells, whereas CD4+ T cell migration was unresponsive to CCL2 (Fig. 5e). These data collectively show that expression of P-glycoprotein by TNF-α-activated brain endothelial cells is required for release of chemotactic factors that stimulate migration of both CD4+ and CD8+ T cells. Moreover, the release of CCL2 appears responsible for the stimulated migration specifically of CD8+ T cells.
Increased expression levels of CCL2 in brain microvessels from multiple sclerosis patients
To determine endothelial CCL2 expression ex vivo, we isolated post-mortem brain microvessels from active demyelinating multiple sclerosis lesions, NAWM and non-neurological control brain tissue. Real-time PCR analysis revealed high endothelial purity as the expression levels of GAFP (astrocytes) and PDGFRβ (pericytes) were just above detection limit, whereas (brain) endothelial markers like ZO-1, claudin-5 and P-glycoprotein were highly expressed (Fig. 5f). CCL2 mRNA gene transcripts were significantly higher in isolated microvessels both in NAWM and active multiple sclerosis lesions compared to transcripts in brain microvessels isolated from non-neurological controls (Fig. 5g), whereas the expression levels of P-gp remained unaffected (Electronic Supplementary Material 9). These results indicate that in NAWM, inflammatory alterations in the brain endothelium are already present.
Endothelial CCL2 knockout mice display reduced CD8+ T cell infiltrates during EAE
To obtain proof of principle that during neuro-inflammation brain endothelial CCL2 drives CD8+ T cell trafficking into the CNS, we induced EAE in endothelial CCL2−/− mice [13] as well as their littermate controls. We evaluated CD8+ T cell influx using 3D rendering of spinal cord venules on day 16 of EAE (peak of the disease). As shown in Fig. 6a, b, high numbers of CD8+ T cells are associated with the vasculature of EAE animals. Vessel-associated CD8+ T cells in WT mice demonstrate extensive clumping with little-to-no obvious space between cell aggregates. By contrast, infiltrating CD8+ T cells in endothelial CCL2−/− mice form smaller aggregates that are clearly resolvable from each other. It thus appears that the density of vessel-associated CD8+ T cells is reduced in endothelial CCL2−/− mice with EAE, compared to WT mice at the same time-point during disease. As shown in Fig. 6c, we observed a significant (p < 0.016) reduction in vessel-associated CD8+ T cell density in endothelial CCL2−/− mice compared to WT mice. These results reinforce the notion that endothelial CCL2 is a crucial regulator of CD8+ T cell trafficking into the central nervous system under pathological conditions.
Reduced CD8+ T cell trafficking in endothelial CCL2−/− mice during EAE CD8+ T cell (in green) extravasation was determined using 3-D rendering of spinal cord venules (in red) of a wild type (WT) or b endothelial CCL2−/− mice on day 16 of EAE. c Quantification of CD8+ T cell extravasation by determining the ratio of # of green pixels/# of pixels occupied by the microvessel (in blue), as an index of vessel-associated CD8+ T cell density. p < 0.0159 as determined by Mann–Whitney U test, n = 5 mice per group
Discussion
In the pathogenesis of multiple sclerosis, CD8+ T cells are present within brains of patients and in animals with EAE, where they may clonally expand and subsequently induce tissue damage and, in particular, axonal damage. However, the mechanism by which CD8+ T cells gain access to the brain remains largely unknown. Here, we describe a novel regulatory role for multi-drug resistance transporter P-glycoprotein as well as the CCL2/CCR2 axis in this process. Our studies suggest that P-glycoprotein regulates the release of CCL2 by inflamed brain endothelium. In that way, the endothelial cells instruct adhered leukocytes, in particular CD8+ T lymphocytes, to migrate.
The prototypic ATP-binding cassette transporters at the brain vasculature are regarded as protectors of the central nervous system by virtue of their active efflux capacity for neurotoxic compounds. Our data extends the role for these efflux pumps, providing first-hand evidence that, under inflammatory conditions, a key member of this family (P-glycoprotein) also regulates leukocyte trafficking into the brain by releasing CCL2. Under neuro-inflammatory conditions, P-glycoprotein translocates from the cytosol to the plasma membrane and intra-endothelial vesicles at the contact site of the adhered T cell. TNF-α treatment of brain endothelial cells resulted in a similar P-glycoprotein distribution pattern (unpublished data), indicating that the observed effects may be regulated by downstream TNF-α receptor signaling events as also described for brain capillaries [29]. Electron microscopy analysis further confirmed our observations of a membrane and vesicle-like expression pattern of P-glycoprotein upon T cell attachment. Recent findings show that, besides T cells being activated by chemokines presented at the endothelial surface of peripheral vascular beds, effector T lymphocytes also cross inflamed endothelial barriers by sensing intra-endothelial chemokines stored within vesicles localized at the contact site [35]. Based on our data, it may be postulated that brain endothelial P-glycoprotein plays a role in this process. Subsequent focal release or presentation of endothelial CCL2 may then be mediated by P-glycoprotein, providing a local cue for the adhered lymphocyte to initiate migration while maintaining low levels of CCL2 in the circulation. That adherent CD8+ T cells appeared most inhibited from migrating is consistent with P-glycoprotein/CCL2 regulating a post-adhesion event.
A controversial issue remains whether ATP-binding cassette transporters themselves are capable of secreting chemokines directly, or are involved in the secretion of other more lipophilic inflammatory substrates, e.g., like bioactive lipids, which in turn may affect CCL2 secretion as a secondary effect [20]. Further research is warranted to define the nature of the P-glycoprotein-mediated secretome and its potential autocrine effect on endothelial cells (chemokine release) or its paracrine effect on the adhering leukocyte (e.g., leukocyte activation).
CCL2 is a pro-inflammatory chemokine induced during a variety of neuro-inflammatory and neurodegenerative diseases [37]. The cognate receptor for CCL2 is chemokine (C–C motif) receptor 2 (CCR2), and the CCL2/CCR2 axis attracts immune cells like monocytes [28], and activated and memory T cells [6] to the site of inflammation. Several reports have indicated the relevance for CCR2 during neuro-inflammation, as mice that lack CCR2 are resistant to the induction of EAE [11, 16]. Moreover, CCR2-deficient leukocytes show impairments in their adhesion and migration capacity across microvascular endothelium in vitro [9, 23, 44]. Our data indicate P-glycoprotein-mediated release of CCL2 preferentially affects CD8+ T cell migration across TNF-α-activated brain microvascular endothelial cells in culture. Although further research is warranted to confirm these findings in primary human endothelial cells, our data suggest that P-glycoprotein-mediated release of CCL2 may also be responsible for controlling trafficking of this leukocyte subset into the central nervous system parenchyma under neuro-inflammatory conditions. This may be because CCR2 is differentially expressed among effector T cell subsets. Indeed, CCR2 appears to be specifically induced on activated CD8+ T cells, whereas CD4+ T cells express a wider range of chemokine receptors [5]. In addition, CCL2/CCR2 interaction can also rescue CD8+ T cells from antigen/growth factor deprivation-apoptosis [7], enabling them to migrate to sites where antigen is available. Moreover, the generation of effector and central memory CD8+ T cells is abrogated in the absence of CCL2, as is the migration of central memory CD8+ T cells to inflammatory sites [41], indicating CCL2 may influence CD8+ T cells at various levels. In multiple sclerosis, CD8+ T cells may clonally expand and may exert their lytic functions [1], such as axonal damage [30].
Brain microvessels isolated from multiple sclerosis patients were shown to contain high levels of CCL2. Our results with endothelial CCL2−/− mice underscore the significance of these clinical findings, highlighting that vascular-derived CCL2 is a critical determinant in the multi-step process of leukocyte extravasation into the central nervous system. The finding of reduced density of vessel-associated CD8+ T cells in endothelial CCL2−/− mice with EAE could imply that the actual extent of these extravasating cells is diminished in the absence of an endothelial pool of CCL2. This interpretation is consistent with the proposed role of CCL2 as an ‘arrest’ chemokine, functioning at the luminal endothelial surface to increase the affinity and avidity of leukocyte integrins and, thus, to promote to stronger leukocyte–endothelial interaction, thereby selectively inducing transendothelial migration attachment [24]. In this regard, it was reported that addition of anti-CCL2 antibody just prior to intravital microscopy, blocked leukocyte adhesion, but not rolling, along brain pial microvessel during EAE [8]. Endothelial CCL2 released at the abluminal surface can alternatively regulate leukocyte transendothelial migration as an ‘inflammatory’ chemokine to stimulate leukocyte chemotaxis [33], and/or serve to concentrate and orient leukocytes within the remodeled subendothelial and/or perivascular space [40], while they await additional cues to advance further into the brain parenchyma. And a more recently proposed role for endothelial CCL2 is as a facilitator of diapedesis to modulate migration at a ‘post-adhesion’ step, when contained in endothelial vesicles inaccessible to the circulation [35].
In conclusion, herein we show that the ATP-binding cassette transporter P-glycoprotein plays a significant role in the selective recruitment of CD8+ T cells into the central nervous system during neuro-inflammation, and that this process may be mediated by CCL2 derived from brain microvascular endothelial cells. Since CD8+ T cells are capable of clonally expanding within multiple sclerosis lesions and subsequently inducing axonal damage and consequent neurodegeneration, our results may provide novel tools to specifically hamper CD8+ T cell trafficking into the brain, thereby preventing severe tissue damage.
Abbreviations
- ATP:
-
Adenosine triphosphate
- CCL2:
-
Chemokine (C–C motif) ligand 2
- EAE:
-
Experimental autoimmune encephalomyelitis
- TNF-α:
-
Tumor necrosis factor-α
References
Babbe H, Roers A, Waisman A, Lassmann H, Goebels N, Hohlfeld R, Friese M, Schroder R, Deckert M, Schmidt S, Ravid R, Rajewsky K (2000) Clonal expansions of CD8(+) T cells dominate the T cell infiltrate in active multiple sclerosis lesions as shown by micromanipulation and single cell polymerase chain reaction. J Exp Med 192(3):393–404
Barreiro O, de la FH, Mittelbrunn M, Sanchez-Madrid F (2007) Functional insights on the polarized redistribution of leukocyte integrins and their ligands during leukocyte migration and immune interactions. Immunol Rev 218:147–164
Barreiro O, Yanez-Mo M, Serrador JM, Montoya MC, Vicente-Manzanares M, Tejedor R, Furthmayr H, Sanchez-Madrid F (2002) Dynamic interaction of VCAM-1 and ICAM-1 with moesin and ezrin in a novel endothelial docking structure for adherent leukocytes. J Cell Biol 157(7):1233–1245
Bleijs DA, Binnerts ME, van Vliet SJ, Figdor CG, van KY (2000) Low-affinity LFA-1/ICAM-3 interactions augment LFA-1/ICAM-1-mediated T cell adhesion and signaling by redistribution of LFA-1. J Cell Sci 113(Pt 3):391–400
Bromley SK, Mempel TR, Luster AD (2008) Orchestrating the orchestrators: chemokines in control of T cell traffic. Nat Immunol 9(9):970–980
Carr MW, Roth SJ, Luther E, Rose SS, Springer TA (1994) Monocyte chemoattractant protein 1 acts as a T-lymphocyte chemoattractant. Proc Natl Acad Sci USA 91(9):3652–3656
Diaz-Guerra E, Vernal R, del Prete MJ, Silva A, Garcia-Sanz JA (2007) CCL2 inhibits the apoptosis program induced by growth factor deprivation, rescuing functional T cells. J Immunol 179(11):7352–7357
dos Santos AC, Barsante MM, Arantes RM, Bernard CC, Teixeira MM, Carvalho-Tavares J (2005) CCL2 and CCL5 mediate leukocyte adhesion in experimental autoimmune encephalomyelitis––an intravital microscopy study. J Neuroimmunol 162(1–2):122–129
Dzenko KA, Song L, Ge S, Kuziel WA, Pachter JS (2005) CCR2 expression by brain microvascular endothelial cells is critical for macrophage transendothelial migration in response to CCL2. Microvasc Res 70(1–2):53–64
Engelhardt B (2008) Immune cell entry into the central nervous system: involvement of adhesion molecules and chemokines. J Neurol Sci 274(1–2):23–26
Fife BT, Huffnagle GB, Kuziel WA, Karpus WJ (2000) CC chemokine receptor 2 is critical for induction of experimental autoimmune encephalomyelitis. J Exp Med 192(6):899–905
Frohman EM, Racke MK, Raine CS (2006) Multiple sclerosis––the plaque and its pathogenesis. N Engl J Med 354(9):942–955
Ge S, Murugesan N, Pachter JS (2009) Astrocyte- and endothelial-targeted CCL2 conditional knockout mice: critical tools for studying the pathogenesis of neuro-inflammation. J Mol Neurosci 39(1–2):269–283
Greenwood J, Heasman SJ, Alvarez JI, Prat A, Lyck R, Engelhardt B (2011) Review: leucocyte–endothelial cell crosstalk at the blood–brain barrier: a prerequisite for successful immune cell entry to the brain. Neuropathol Appl Neurobiol 37(1):24–39
Hohlfeld R, Wekerle H (2004) Autoimmune concepts of multiple sclerosis as a basis for selective immunotherapy: from pipe dreams to (therapeutic) pipelines. Proc Natl Acad Sci USA 101(Suppl 2):14599–14606
Izikson L, Klein RS, Charo IF, Weiner HL, Luster AD (2000) Resistance to experimental autoimmune encephalomyelitis in mice lacking the CC chemokine receptor (CCR)2. J Exp Med 192(7):1075–1080
Juliano RL, Ling V (1976) A surface glycoprotein modulating drug permeability in Chinese hamster ovary cell mutants. Biochim Biophys Acta 455(1):152–162
Kooij G, Backer R, Koning JJ, Reijerkerk A, van Horssen J, van der Pol SM, Drexhage J, Schinkel A, Dijkstra CD, den Haan JM, Geijtenbeek TB, de Vries HE (2009) P-glycoprotein acts as an immunomodulator during neuro-inflammation. PLoS One 4(12):e8212
Kooij G, Mizee MR, van Horssen J, Reijerkerk A, Witte ME, Drexhage JA, van der Pol SM, Van Het HB, Scheffer G, Scheper R, Dijkstra CD, van DV, de Vries HE (2011) Adenosine triphosphate-binding cassette transporters mediate chemokine (C–C motif) ligand 2 secretion from reactive astrocytes: relevance to multiple sclerosis pathogenesis. Brain 134(Pt 2):555–570
Kooij G, van Horssen J, Bandaru VV, Haughey NJ, de Vries HE (2012) The role of ATP-binding cassette transporters in neuro-inflammation: relevance for bioactive lipids. Front Pharmacol 3:74
Kooij G, van Horssen J, de Lange EC, Reijerkerk A, van der Pol SM, Van Het HB, Drexhage J, Vennegoor A, Killestein J, Scheffer G, Oerlemans R, Scheper R, van DV, Dijkstra CD, de Vries HE (2010) T lymphocytes impair P-glycoprotein function during neuro-inflammation. J Autoimmun 34(4):416–425
Kuijk LM, Klaver EJ, Kooij G, van der Pol SM, Heijnen P, Bruijns SC, Kringel H, Pinelli E, Kraal G, de Vries HE, Dijkstra CD, Bouma G, Van DI (2012) Soluble helminth products suppress clinical signs in murine experimental autoimmune encephalomyelitis and differentially modulate human dendritic cell activation. Mol Immunol 51(2):210–218
Kuziel WA, Morgan SJ, Dawson TC, Griffin S, Smithies O, Ley K, Maeda N (1997) Severe reduction in leukocyte adhesion and monocyte extravasation in mice deficient in CC chemokine receptor 2. Proc Natl Acad Sci USA 94(22):12053–12058
Ley K (2003) Arrest chemokines. Microcirculation 10(3–4):289–295
Ley K, Laudanna C, Cybulsky MI, Nourshargh S (2007) Getting to the site of inflammation: the leukocyte adhesion cascade updated. Nat Rev Immunol 7(9):678–689
Loscher W, Potschka H (2005) Drug resistance in brain diseases and the role of drug efflux transporters. Nat Rev Neurosci 6(8):591–602
Mahad DJ, Ransohoff RM (2003) The role of MCP-1 (CCL2) and CCR2 in multiple sclerosis and experimental autoimmune encephalomyelitis (EAE). Semin Immunol 15(1):23–32
Matsushima K, Larsen CG, DuBois GC, Oppenheim JJ (1989) Purification and characterization of a novel monocyte chemotactic and activating factor produced by a human myelomonocytic cell line. J Exp Med 169(4):1485–1490
Miller DS (2010) Regulation of P-glycoprotein and other ABC drug transporters at the blood–brain barrier. Trends Pharmacol Sci 31(6):246–254
Neumann H, Medana IM, Bauer J, Lassmann H (2002) Cytotoxic T lymphocytes in autoimmune and degenerative CNS diseases. Trends Neurosci 25(6):313–319
Nourshargh S, Hordijk PL, Sixt M (2010) Breaching multiple barriers: leukocyte motility through venular walls and the interstitium. Nat Rev Mol Cell Biol 11(5):366–378
Paul D, Cowan AE, Ge S, Pachter JS (2013) Novel 3D analysis of Claudin-5 reveals significant endothelial heterogeneity among CNS microvessels. Microvasc Res 86:1–10
Randolph GJ, Furie MB (1995) A soluble gradient of endogenous monocyte chemoattractant protein-1 promotes the transendothelial migration of monocytes in vitro. J Immunol 155(7):3610–3618
Reijerkerk A, Lopez-Ramirez MA, Van Het HB, Drexhage JA, Kamphuis WW, Kooij G, Vos JB, van der Pouw Kraan TC, van Zonneveld AJ, Horrevoets AJ, Prat A, Romero IA, de Vries HE (2013) MicroRNAs regulate human brain endothelial cell-barrier function in inflammation: implications for multiple sclerosis. J Neurosci 33(16):6857–6863
Shulman Z, Cohen SJ, Roediger B, Kalchenko V, Jain R, Grabovsky V, Klein E, Shinder V, Stoler-Barak L, Feigelson SW, Meshel T, Nurmi SM, Goldstein I, Hartley O, Gahmberg CG, Etzioni A, Weninger W, Ben-Baruch A, Alon R (2012) Transendothelial migration of lymphocytes mediated by intra-endothelial vesicle stores rather than by extracellular chemokine depots. Nat Immunol 13(1):67–76
Slot JW, Geuze HJ (2007) Cryosectioning and immuno-labeling. Nat Protoc 2(10):2480–2491
Sokolova A, Hill MD, Rahimi F, Warden LA, Halliday GM, Shepherd CE (2009) Monocyte chemoattractant protein-1 plays a dominant role in the chronic inflammation observed in Alzheimer’s disease. Brain Pathol 19(3):392–398
Springer TA (1990) Adhesion receptors of the immune system. Nature 346(6283):425–434
van de Ven R, Oerlemans R, van der Heijden JW, Scheffer GL, de Gruij TD, Jansen G, Scheper RJ (2009) ABC drug transporters and immunity: novel therapeutic targets in autoimmunity and cancer. J Leukoc Biol 86(5):1075–1087
van Horssen J, Bo L, Vos CM, Virtanen I, de Vries HE (2005) Basement membrane proteins in multiple sclerosis-associated inflammatory cuffs: potential role in influx and transport of leukocytes. J Neuropathol Exp Neurol 64(8):722–729
Wang T, Dai H, Wan N, Moore Y, Dai Z (2008) The role for monocyte chemoattractant protein-1 in the generation and function of memory CD8+ T cells. J Immunol 180(5):2886–2893
Weiss HA, Millward JM, Owens T (2007) CD8+ T cells in inflammatory demyelinating disease. J Neuroimmunol 191(1–2):79–85
Weksler BB, Subileau EA, Perriere N, Charneau P, Holloway K, Leveque M, Tricoire-Leignel H, Nicotra A, Bourdoulous S, Turowski P, Male DK, Roux F, Greenwood J, Romero IA, Couraud PO (2005) Blood–brain barrier-specific properties of a human adult brain endothelial cell line. FASEB J 19(13):1872–1874
Yopp AC, Fu S, Honig SM, Randolph GJ, Ding Y, Krieger NR, Bromberg JS (2004) FTY720-enhanced T cell homing is dependent on CCR2, CCR5, CCR7, and CXCR4: evidence for distinct chemokine compartments. J Immunol 173(2):855–865
Acknowledgments
We thank the Netherlands Brain Bank for providing human brain tissues and Dr. Shujun Ge who provided the endothelial CCL2−/− mice. This work was supported by grants 016.046.314 from the Netherlands Organization of Scientific Research, and MS 08-652 from the Dutch foundation of Multiple Sclerosis Research, to G. Kooij; and Grants R0-1-MH061525 from the National Institutes of Health, and PP-1215 and RG 4828-A-5 from the National Multiple Sclerosis Society, to J.S. Pachter.
Conflict of interest
The authors declare that they have no conflict of interest.
Author information
Authors and Affiliations
Corresponding author
Electronic supplementary material
Below is the link to the electronic supplementary material.
Rights and permissions
About this article
Cite this article
Kooij, G., Kroon, J., Paul, D. et al. P-glycoprotein regulates trafficking of CD8+ T cells to the brain parenchyma. Acta Neuropathol 127, 699–711 (2014). https://doi.org/10.1007/s00401-014-1244-8
Received:
Accepted:
Published:
Issue Date:
DOI: https://doi.org/10.1007/s00401-014-1244-8