Abstract
Background
Pleiotropic (lipid lowering-independent) effects of statins are attributed to their antiinflammatory, antioxidant, and/or vascular actions. Extensive studies in various experimental models have established that pretreatment with simvastatin significantly protects heart and kidney injured by ischemia–reperfusion (IR). The purpose of the present study was to examine the effect of simvastatin on intestinal recovery and enterocyte turnover after intestinal IR injury in rats.
Methods
Male Sprague–Dawley rats were divided into three experimental groups: (1) sham rats underwent laparotomy, (2) IR-rats underwent occlusion of both superior mesenteric artery and portal vein for 30 min followed by 48 h of reperfusion, and (3) IR-SIM rats underwent IR and were treated with oral simvastatin (10 mg/kg) given by gavage immediately before and 24 h after operation. Intestinal structural changes, Park’s injury score, enterocyte proliferation and enterocyte apoptosis were determined 24 h following IR. A non-parametric Kruskal–Wallis ANOVA test was used for statistical analysis with P less than 0.05 considered statistically significant.
Results
Treatment with simvastatin resulted in a significant increase in bowel and mucosal weight in ileum, villus height and crypt depth in jejunum and ileum compared to IR animals. IR-SIM rats had also a significantly lower intestinal injury score as well as lower apoptotic index in jejunum and ileum compared to IR animals.
Conclusions
Treatment with simvastatin prevents gut mucosal damage and inhibits programmed cell death following intestinal IR in a rat.
Similar content being viewed by others
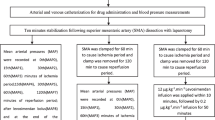
Avoid common mistakes on your manuscript.
Introduction
Enteric ischemia–reperfusion (IR) injury is a well known entity, yet not all the mechanisms involved are well recognized. The mechanisms of intestinal injury following an IR event include nonspecific damage induced by ischemia and damage caused by reperfusion [1–3]. During the ischemic phase, the main causes for the tissue damage are an inadequate oxygen supply relative to metabolic tissue demands, depletion of cellular energy stores and accumulation of toxic metabolites, resulting in cell necrosis. During the reperfusion phase, several factors mediate the injury and apoptosis, including reactive oxygen species (ROS), inflammatory leukocytes, mitochondrial dysfunction, and the release of cytochrome c from mitochondria into the cytosol. Reestablishment of oxygen supply causes ROS formation, leading to reactions with poly-unsaturated fatty acids and lipid peroxidation within cell membranes [4]. The re-established blood supply facilitates neutrophils recruitment, upon which an immunologic cascade is started, and tissue damage is exacerbated by various cytokines and growth factors, leading to increased bowel permeability, gut barrier dysfunction, translocation of bacteria and bacterial products into the systemic blood circulation, and hence a systemic life threatening situation [3].
Therapy of intestinal IR injury includes the pharmacological agents that can prevent ischemic injury as well as agents that can prevent production of ROS and agents which can improve intestinal rehabilitation following the IR event. Statins are inhibitors of HMG-CoA reductase, and cause lowering of plasma concentration of cholesterol and especially the LDL fraction by blocking intracellular synthesis of cholesterol. Pleiotropic (lipid lowering-independent) effects of statins are attributed to their antiinflammatory, antioxidant, and/or vascular actions. Statins are classified according to their physical–chemical properties into two major groups: the first group includes lipophilic statins (simvastatin, lovastatin, atorvastatin, cerivastat) which are metabolized via the hepatic system of cytochrome P450 isoenzymes, especially isoenzyme CYP3A4. The second group includes hydrophilic statins (pravastatin), which are not metabolized by cytochrome P450 and are excreted by the kidney [5]. Extensive studies in various experimental models have established that pretreatment with simvastatin significantly protects heart and kidney injured by IR [6, 7].
Our study was designed to evaluate the effect of simvastatin on intestinal recovery and enterocyte turnover after intestinal IR injury in rats.
Materials and methods
Animals
Surgical procedures and animal care were conducted in compliance with the guidelines established by the “Guide for the Care and Use of Laboratory Animals”, Rappaport Faculty of Medicine, Technion (Haifa, Israel). Male Sprague–Dawley rats (250–300 g) were used in this study. Animals were housed in wire-bottomed cages and were acclimatized at 21°C on 12:12-h light–dark cycle for a minimum of three days before the experiment. The rats were allowed access to water and chow ad libitum.
Experimental design
Forty rats were divided randomly into three experimental groups of eight rats each. (1) Group A rats underwent midline laparotomy (Sham); (2) Group B (IR) animals underwent occlusion of the superior mesenteric artery (SMA) and portal vein (PV) for 30 min followed by 24 h of reperfusion; (3) Group C rats (IR-SMV) were pre-treated with oral simvastatin given by gavage at a dose of 10 mg/kg, once daily, 48 h before and 24 h after the IR event.
Surgery
After overnight fasting, the animals were anesthetized with intraperitoneal ketamine (90 mg/kg) and xylasine (10 mg/kg). Using sterile techniques, the abdomen was opened using a midline incision. A sham operation was performed by identification and isolation of the SMA and PV without clamping. In IR animals, SMA and PV were occluded with a microbulldog clamp for 30 min. During the period of ischemia the abdominal wall incision was kept approximated to prevent fluid and heat loss. At the end of the ischemic period, the clamp was released, thus starting the reperfusion period of 24 h. Immediately following reperfusion, the rats were resuscitated with a 3-ml intraperitoneal injection of warm (37°C) 0.9% NaCl solution and the abdominal cavity was closed in two layers with a running suture of 3/0 Dexon. The rats were fasted for 24 h but were allowed free access to water.
Intestinal mucosal parameters
All animals were sacrificed 24 h following the ischemia. The entire small intestine was carefully removed and placed on ice. Portions of intestine 10 cm distal to the ligament of Treitz and 10 cm proximal to the ileo-cecal region were removed, representing the proximal (jejunum) and distal (ileum) segments. Intestinal segments were split on the antimesenteric border, washed with cold saline, dried, and weighed. Each segment was rinsed thoroughly with physiological saline and opened longitudinally on its antimesenteric border to expose the intestinal mucosa. The mucosa was scarped from the underlying tissue with a glass slide. Total RNA, DNA and protein from the jejunum and ileum was extracted sequentially with a TRIzol reagent. DNA and protein were extracted by the method of Chromsczynski [8].
Histology
Tissue samples were removed from the jejunum and ileum and were immediately fixed in 4% neutral-buffered formalin. The samples were then embedded in paraffin and sectioned. Deparaffinized 5 μm sections were stained with hematoxylin and eosin. The villus height and crypt depth for each specimen were measured using an objective mounted micrometer (100× magnification) and an optical microscope (10 × 100 magnification). Villus height and crypt depth data were from eight rats, and each measurement consists of the mean of five villi and crypts.
The mucosal damage of the small bowel was graded using the Park score [9]: 0, normal mucosa; 1, subepithelial space at villus tip; 2, more extended subepithelial space; 3, epithelial lifting along villus sides; 4, denuded villi; 5, loss of villus tissue; 6, crypt layer infarction; 7, transmucosal infarction; 8, transmural infarction.
Enterocyte proliferation and apoptosis
Crypt cell proliferation was assessed using 5-bromodeoxyuridine (5-BrdU). Standard BrdU labeling reagent (Zymed Laboratories, Inc, San Francisco, CA) was injected intraperitoneally at a concentration of 1 ml/100 g body weight 2 h before sacrifice. Tissue slices (5 μm) were stained with a biotinylated monoclonal anti-BrdU antibody system provided in a kit form (Zymed Laboratories, Inc, San Francisco, CA). An index of proliferation was determined as the ratio of crypt cells staining positively for BrdU per ten crypts.
Additional 5 μm thick sections were prepared to establish the degree of enterocyte apoptosis. Immunohistochemistry for Caspase-3 (Caspase-3 cleaved concentrated polyclonal antibody; dilution 1:100; Biocare Medical, Walnut Greek, CA) was performed for identification of apoptotic cells using a combination of the streptovidin-biotin-peroxidase method and microwave antigen retrieval on formalin-fixed, paraffin-embedded tissues according to the manufacturer’s protocols. The apoptotic index (AI) was defined as the number of apoptotic cells per ten villi.
A qualified pathologist blinded as to the source of intestinal tissue performed all measurements.
Statistical analysis
The data are expressed as the mean ± SEM. Statistical analysis of parameters of adaptation, enterocyte proliferation, and apoptosis was performed using the non-parametric Kruskal–Wallis ANOVA test, followed by the corrected Mann–Whitney test, with P less than 0.05 considered statistically significant.
Results
Body weight changes
About 90% of animals suffered from appetite loss, diarrhea and weight loss. IR rats demonstrated a lower final body weight (95 ± 1 vs. 98 ± 1% preoperative, P < 0.05) compared to control animals (Group A) (Fig. 1). Treatment with simvastatin (Group C) did not change significantly final body weight compared to IR animals (Group B).
Intestinal mucosal parameters
There was no difference in bowel diameter in duodenum, jejunum and ileum among all three experimental groups. IR injury (Group B) resulted in a significant decrease in bowel (15.3 ± 0.7 vs. 18.2 ± 1.4 mg/cm length/100 g body weight, P < 0.05) and mucosal (5.7 ± 0.5 vs. 7.6 ± 0.8 mg/cm length/100 g body weight, P < 0.05) weight in ileum compared to control animals (Group A) (Fig. 2). Following oral simvastatin administration, IR-rats (Group C) demonstrated a significant increase in ileal bowel (18.3 ± 0.3 vs. 15.3 ± 0.7 mg/cm length/100 g body weight, P < 0.05) and mucosal (7.5 ± 0.3 vs. 5.7 ± 0.5 mg/cm length/100 g body weight, P < 0.05) weight compared to IR-untreated animals (Group B).
Microscopic bowel appearance
Villus height significantly decreased in jejunum (315 ± 23 vs. 368 ± 13 μm, P < 0.05) in IR-rats (Group B) compared to sham animals (Group A) (Fig. 3). Oral simvastatin (Group C) resulted in a significant increase in crypt depth in jejunum (192 ± 14 vs. 163 ± 5 μm, P < 0.05) compared to IR-nontreated animals (Group B), and in a trend toward an increase in villus height in jejunum and ileum; however, this trend did not achieve statistical significance.
Park’s intestinal injury grade
IR injury (Group B) led to a significant increase in the mean intestinal injury grade (Park’s criteria) in ileum (2.8 ± 0.6 vs. 0.8 ± 0.5, p < 0.05) compared to sham animals as well as in a trend toward an increase in injury grade in jejunum; however, this trend was not statistically significant (Fig. 4). Although oral simvastatin supplementation led to a trend toward decrease in the injury grade in ileum compared to IR-animals, this trend did not achieve statistical significance.
Cellular proliferation and apoptosis
In contrast to our previous experiment in which IR resulted in a significant inhibition of cell proliferation within first 24 h, the current data suggest that 48 h following the IR event enterocyte proliferation increases non-significantly in ileum compared to sham animals, suggesting a compensatory process (Fig. 5). Treatment with simvastatin (Group C) did not change significantly cell proliferation rates in jejunum and ileum compared to IR-animals (Group B), but this group demonstrated a greater index of proliferation in ileum compared to control animals.
Effect of ischemia–reperfusion and oral simvastatin on crypt cell proliferation. 5-BrdU incorporation into proliferating jejunal and ileal crypt cells was detected with a goat anti-BrdU antibody. Values are mean ± SDV. IR ischemia–reperfusion, SMV simvastatin *P < 0.05 IR versus Sham rats, † P < 0.05 IR-SMV versus IR rats
The frequency of apoptotic cells was increased in the animals after the IR insult in jejunum (1.4 ± 0.1 vs. 0.6 ± 0.2 apoptotic cells/10 villi, P < 0.05) and ileum (1.9 ± 0.7 vs. 0.7 ± 0.1, P < 0.05) compared to sham animals (Fig. 6). The AI decreased following simvastatin administration in jejunum (twofold decrease, P < 0.05) and ileum (fivefold decrease, P < 0.05) compared to IR-untreated animals.
Effect of IR and oral insulin on enterocyte apoptosis. These representative sections demonstrate that cell apoptosis is increased following IR compared to sham animals (arrows). Following administration of oral simvastatin, IR-rats demonstrated a marked decrease in a number of apoptotic cells compared to IR-nontreated animals. Values are mean ± SDV. IR ischemia–reperfusion, SMV-simvastatin *P < 0.05 IR versus Sham rats, † P < 0.05 IR-SMV versus IR rats
Discussion
Simvastatin is a well known drug that belongs to the lipophilic statins, and was first introduced as a lipid-lowering drug, affecting the low-density fraction of cholesterol mainly [10]. This effect, exerted through the HMG-CoA reductase inhibition, was initially described, but other effects, referred to as pleiotropic effects, have been identified as well, and different mechanisms of action have been described [11]. Many experiments have shown beneficial effects of simvastatin on IR injury in deferent organs and tissues including heart, lungs, liver and kidneys. Pirat et al. have demonstrated that simvastatin attenuates lung injury during intestinal IR in a rat model [12]. Dibazar et al. published similar conclusions regarding lung and liver injury after ischemia reperfusion injury, attributing this positive effect of simvastatin to inhibition of inflammation and apoptotic pathways [12]. Liver protection by preconditioning with simvastatin, prior to ischemia reperfusion injury induction, expressed by diminished elevation of serum ALT levels and diminished apoptosis rate, correlated with heme-oxygenase activity, and was attributed to the heme-oxygenase-1 induction [7]. Likewise, preconditioning with simvastatin prior to cardiac ischemic insult reduced myocardial infarction size by means of upregulation of NO production [6]. In contrast with its gradual effect of lowering lipid levels, the pleiotropic effects of statins seem to be rapid and may only require a single dose, as shown by Todorovic et al. in a rat model of renal ischemia reperfusion injury [13].
The effects of statins in prevention of intestinal IR injury are unknown. The positive effects of statins on the gastrointestinal tract has been described in certain gastrointestinal disorders. Simvastatin exerted a positive effect in a dextran sulfate sodium model of colitis that was comparable to the effect of anti-TNF-alpha in this model [14].
Since statins have a positive effect during IR events in different organs, we hypothesized in the present study that these agents could enhance intestinal regeneration following IR injury. Statins might stimulate enterocyte turnover by a direct stimulation of proliferation, cell migration, or by inhibition of enterocyte apoptosis. Alternatively, they may exert their stimulating effect on mucosal restitution by stimulating the release of various cytokines or peptide growth factors. Our experimental protocol was based on pilot studies which demonstrated that occlusion of the SMA for 30 min causes small changes, while occlusion of both the SMA and the PV leads to visually severe but reversible intestinal ischemia. Our results have shown that IR causes a direct intestinal mucosal injury. This is evident from the increased Park’s intestinal injury score. In addition, IR leads to intestinal mucosal hypoplasia. The observed decreased bowel and mucosal weight, as well as decreased villus height and crypt depth in this model support this conclusion. Although dry weight was not measured in this experiment, in a recent pilot study we demonstrated that the changes in the weights of dry and wet bowel following IR were parallel to each other. Therefore, it should be emphasized, that mucosal hypoplasia rather than edema, vascular engorgement or intestinal muscle atrophy is responsible for the decreased intestinal mass after IR. Histologically, IR rats showed decreased villus height compared to sham animals, suggesting decreased absorptive surface area. Decreased villus height is presumably due to decreased cell proliferation and migration along the villus axis during the first 24 h after IR or due to the specific arrangement of the villus microvasculature, which results in an oxygen tension gradient along the villus. IR rats also showed an increased rate of cell death via apoptosis. Although necrosis is responsible for the intestinal cell death during the ischemic phase, apoptosis or “programmed cell death” has recently been recognized to be a key factor in enterocyte turnover and gut barrier function following an IR insult [5]. Apoptosis is widely considered to be a distinct entity different from necrotic cell death and is tightly regulated by a variety of extracellular and intracellular signals. Signaling pathways contributing to apoptosis are often driven by the sequential activation of caspases, mediated, in part, by mitochondrial depolarization and cytochrome c release. The observed changes in the current study are consisted with our previous experiments [15–17].
In contrast to our previous studies, in the current experiment the rates of cell proliferation were increased compared to control animals. While previously studied models have shown that cell proliferation was decreased 24 h after intestinal IR damage, probably as a result of ischemic effects, the current data suggest that 48 h following IR cell proliferation undergoes a compensatory up-regulation, suggesting tissue repair.
Oral simvastatin has produced various beneficial effects in our study. Pretreatment with oral simvastatin, however, did not protect the intestinal mucosa from damage caused by IR. The IR-SMV group manifested an intestinal mucosal injury grade similar to IR-untreated animals, suggesting similar degrees of intestinal damage. However, exposure to SMV accelerated intestinal mucosal repair and enhanced enterocyte turnover. This is evident from the significant increase in bowel and mucosal weight, as well as a trend toward an increase in villus height in this model. Our findings suggest that proliferation of crypt cells did not change significantly following oral simvastatin administration and is closely correlated with unchanged crypt depth. Treatment with simvastatin resulted in a significant decrease in cell apoptosis rate, which may represent an additional mechanism which maintains mucosal integrity following IR. Preventive administration of simvastatin may favor the survival of epithelial cells present in the cellular sheet that covers the mucosa at the end of the ischemic period, and the accelerated recovery of the mucosa may be due to increased cellular proliferation as well as decreased cellular death via apoptosis. The antiapoptotic effects of simvastatin were described previously in different cells and tissues. Simvastatin attenuates homocysteine induced endothelial cell apoptosis and caspase 3 activation [18]. Simvastatin prevents endotoxemic liver injury by inhibiting leukocyte infiltration and hepatocyte apoptosis via the 3-hydroxy-3-methyl-glutaryl-coenzyme A reductase pathway [19].
In conclusion, administration of oral simvastatin does not prevent ischemic damage but accelerates intestinal recovery and decreases cell death via apoptosis following an IR event in a rat model. These preliminary observations suggest that simvastatin may have clinical utility for the patient with intestinal IR injury.
References
Granger DN, Hollwarth ME, Park DA (1986) Ischemia reperfusion injury: role of oxygen-derived free radicals. Acta Physiol Scand (Suppl) 548:47–56
Haglund U, Bergqvist D (1999) Intestinal ischemia-the basics. Langenbecks Arch Surg 384:233–238
Khanna A, Rossman J, Caty MG, Fung HL (2003) Beneficial effects of intraluminal nitroglycerin in intestinal ischemia–reperfusion injury in rats. J Surg Res 114:15–24
Moore RM, Muir WW, Granger DN (1995) Mechanisms of gastrointestinal ischemia–reperfusion injury and potential therapeutic interventions: a review and its implications in the horse. J Vet Intern Med 9:115–132
Neuvonen PJ, Backman JT, Niemi M (2008) Pharmacokinetic comparison of the potential over-the-counter statins simvastatin, lovastatin, fluvastatin and pravastatin. Clin Pharmacokinet 47:463–474
Bao N, Ushikoshi H, Kobayashi H, Yasuda S, Kawamura I, Iwasa M, Yamaki T, Sumi S, Nagashima K, Aoyama T, Kawasaki M, Nishigaki K, Takemura G, Minatoguchi S (2009) Simvastatin reduces myocardial infarct size via increased nitric oxide production in normocholesterolemic rabbits. J Cardiol 53:102–107
Lai IR, Chang KJ, Tsai HW, Chen CF (2008) Pharmacological preconditioning with simvastatin protects liver from ischemia reperfusion injury by heme oxygenase-1 induction. Transplantation 85:732–738
Chomczynski P (1993) A reagent for the single-step simultaneous isolation of RNA, DNA and proteins from cell and tissue samples. BioTechniques 15:532–533
Park PO, Haglund U, Bulkley GB, Falt K (1990) The sequence of development of intestinal tissue injury after strangulation ischemia and reperfusion. Surgery 107:574
Tobert JA (1987) New developments in lipid-lowering therapy: the role of inhibitors of hydroxymethylglutaryl-coenzyme A reductase. Circulation 76:534–538
Pirat A, Zeyneloglu P, Ademir D, Yucel M, Ozen O, Candan S, Arsian G (2006) Pretreatment with simvastatin reduces lung injury related to intestinal ischemia reperfusion in rats. Anesth Analg 102:225–232
Dibazar F, Hajipour B, Hosseinia MM, Hemmati MR, Ghandiha A (2008) Simvastatin decreases hepatic ischemia/reperfusion induced liver and lung injury in rats. Folia Morphol (Warsz) 67:231–235
Todorovic Z, Nesic Z, Stojanovic R, Basta-Jovanovic G, Radojevic-Skodric S, Velickovic R, Chatterjee PK, Thiemermann C, Prostran M (2008) Acute protective effects of simvastatin in the rat model of renal ischemia–reperfusion injury: It is never too late for the pretreatment. J Pharmacol Sci 107:465–470
Kanagarajan N, Nam JH, Noah ZA, Murthy S (2008) Disease modifying effect of statins in dextran sulfate sodium model of mouse colitis. Inflamm Res 57:34–38
Sukhotnik I, Brod V, Lurie M, Rahat MA, Lahat N, Mogilner JG, Bitterman H (2009) The effect of 100% oxygen on intestinal preservation and recovery following ischemia- reperfusion injury in rats. Crit Care Med 37:1054–1061
Sukhotnik I, Helou H, Lurie M, Khateeb K, Bejar J, Coran AG, Mogilner JG, Shiloni E (2007) The effect of leptin on intestinal recovery following ischemia–reperfusion injury in a rat. Ped Surg Int 23:473–478
Sukhotnik I, Khateeb K, Mogilner JG, Helou H, Lurie M, Coran AG, Shiloni E (2007) Dietary glutamine supplementation prevents mucosal injury and modulates intestinal epithelial restitution following ischemia–reperfusion injury in the rat. Dig Dis Sci 52:1497–1504
Xu Z, Lu G, Wu F (2009) Simvastatin suppresses homocysteine induced apoptosis in endothelial cells: roles of caspase-3, cIAP-1 and cIAP-2. Hypertens Res 32:375–380
SSlotta JE, Laschke MW, Wang Y, Schilling MK, Menger MD, Thorlacius H (2009) Inhibition of 3-hydroxy-3-methyl-glutaryl-coenzyme A reductase reduces leukocyte recruitment and hepatocyte apoptosis in endotoxin induced liver injury. J Investig Med 57:645–649
Author information
Authors and Affiliations
Corresponding author
Additional information
N. Slijper and I. Sukhotnik equally contributed to the preparation of the manuscript.
Rights and permissions
About this article
Cite this article
Slijper, N., Sukhotnik, I., Chemodanov, E. et al. Effect of simvastatin on intestinal recovery following gut ischemia–reperfusion injury in a rat. Pediatr Surg Int 26, 105–110 (2010). https://doi.org/10.1007/s00383-009-2508-6
Published:
Issue Date:
DOI: https://doi.org/10.1007/s00383-009-2508-6