Abstract
Developing biomaterial implants for bone regeneration usages has earned great attention during the last two decades. Doping technique is one of the recent approaches that have great promises in the enhancement of biomaterials features. Herein, the features of biphasic TCP/HA were modified by single and co-doping using silver (Ag+) and zirconium (Zr4+) on the expense of calcium utilizing chemical precipitation method. Doping effect on the physicochemical, thermal behaviour, particles charge, morphologies and mechanical properties was determined. Furthermore, dielectric, antibacterial activity, bioactivity and cell death mode (MG63 cell line) were also evaluated. It was confirmed that surface area and total pore volume of the prepared samples were improved upon doping. Negative particle charges, especially for the Zr4+ and the co-doped samples, were confirmed. The co-doped TCP/HA samples possess a strong bactericidal effect against all tested strains compared with the single-doped samples. They also showed a significant effect on the bioactivity properties and the electrical conductivity. The cell mode of death results also supports the importance of transition metal single and co-doping. Implementation of current co-doped biphasic calcium phosphate materials in bone reformation is encouraged due to their proved enhanced features.
Similar content being viewed by others
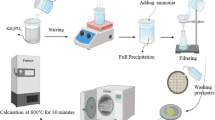
Avoid common mistakes on your manuscript.
1 Introduction
Biphasic TCP/HA (BCPs) materials have drawn attention for hard tissue regeneration because of their structural and chemical resemblance with the inorganic component of bone [1]. Its great osteoconductivity is owed to its capability to bond with bone and soft tissue along with motivating the bone growth [2]. Various methods were developed to attain BCP including sol–gel [3], hydrothermal [4], hydrolysis [5] or microwave treatment [6]. However, the quantities obtained by these methods are not enough to provide the potential needs of such materials applicable for different fields. In addition, the used precursors for these mentioned methods are expensive, which precludes the large-scale productions. Considering the economic benefits, alternative low-cost starting materials are to be used. In 2014, our team conducted previous work on the production of BCP from phosphogypsum (PG) [7]. PG is an industrial waste material mostly made of gypsum matrix (CaSO4·2H2O) with extra impurities. It might be safely used as a calcium source as it is free from radioactive elements and heavy metals [8]. Furthermore, wet chemical precipitation method was reported as a simple and inexpensive preparation method for BCP using egg shells or calcium nitrate or calcium carbonate as sources of calcium. Moreover, the yield of this method could be adjusted by controlling the solvent type as well as the pH of the reaction [9]. Moreover, it was earlier highlighted that the final properties could be amended by the incorporation of transition metals into BCP on expense of calcium. Doping process had a significant effect on the physicochemical, mechanical, bio-mineralization and the interaction of the BCP with the biological environments [10]. Therefore, transition metal substitutions have gained a lot of attention in augmentation of bone sites damaged by different causes.
In this regard, several previous researches have explored both single and co-doping effects on the obtained features of some bioactive materials. For instance, Veerla et al. [11] have developed hydroxyapatite nanosystems for the cancer therapy, which were doped by silver and/or iron ions. Their results indicated the co-doping had enhanced the anticancer effect along with normal cells viability. Türk et al. [12] also have compared between two types of hydroxyapatite doped with zinc and silver through biomimetic fabrication in the absence and the presence of multi-walled carbon nanotubes (fMWCNT). Their results demonstrated impressive cytocompatibility for co-doped samples. Wu et al. [13] fabricated calcium phosphate coatings in amorphous phase that was co-doped by silver and tantalum or silver alone. They have declared that the existence of tantalum had adjusted the release of silver ions and controlled their antibacterial efficiencies. This highlights the previous trails related to silver doping in calcium phosphates materials and positive effect on their final characteristics. Moreover, other researchers have stressed out the great influence achieved for some biomaterials that have been co-doped with two transition metals including zirconium oxide. Kaygili and Keser [14] have enhanced the crystalline nature of hydroxyapatite by inclusion of three co-dopants that contain zirconium. Abdellahi et al. [15] also have reported enhanced hyperthermia application of strontium hexaferrite co-doped by zinc and zirconium. Opraet et al. have reflected the positive influence of zirconium on the final conductivity features of titanium oxide materials.
Accordingly, the combination of both Ag and Zr as co-dopants might exhibit impressive enhancement for TCP/HA prepared in this study as an affordable biocompatible material. Therefore, the effect of the transition metals on the physical structure and chemical integrity is assessed by using X-rays diffraction (XRD) and Fourier-transform infrared spectroscopy (FTIR) before and after doping with transition metals. Thermal behaviour is assessed using differential scanning calorimetry (DSC), while, surface area and zeta potential were determined by Brunauer–Emmett–Teller (BET) and Malvern nano-Zetasizer, respectively. Morphology and size of the obtained samples were scanned via scanning electron microscopy (SEM). Their mechanical and dielectric properties with different dopant concentrations were also assessed. Moreover, antibacterial characteristics of the samples were studied against gram-positive, gram-negative bacterial species and yeast broth. Moreover, the bioactivity testing of the doped samples was carried out in simulated body fluid (SBF) to confirm the formation of apatite onto the surfaces using SEM coupled with energy-dispersive X-rays (EDX) and FTIR. Finally, cell death mode of MG63 cell line was also determined against the developed samples in this work.
2 Materials and methods
2.1 Preparation of Ag- and/or Zr-doped BCP
Ag- and/or Zr-doped BCP powders were prepared utilizing wet precipitation method. In this work, Ca10(PO4)6(OH)2 was doped with Ag, Zr and a mixture of Ag and Zr[Ca10–x(Ag. Zr or Ag + Zr) x(PO4)6(OH)2] with different concentrations of doping [(xAg or Zr = 0, 0.2, 0.4 and 0.8),(x[Ag + Zr] = 0, 0.2 + 0.2 and 0.4 + 0.4)] were synthesized by the wet precipitation method. Typically, the individual doping of Ag or Zr was with different concentrations of doping (0, 0.2, 0.4 and 0.8) and the bidoping of Ag and Zr was with different concentrations of doping (0.2 + 0.2 and 0.4 + 0.4). To obtain BCP and Ag- and/or Zr-doped powders, a series of steps were done. The appropriate amounts of PG waste material, AgNO3 and/or ZrOCl2.8H2O were mixed according to calculated x values under vigorous stirring in deionized water and the solutions pH was adjusted usingNH4OH. Followed by adding appropriate amounts of H3PO4, and the reaction temperature was adjusted at 80 °C. The resulting precipitates were washed several times with deionized water then filtered. The powders were dried at 100 °C for 5 h then calcined at 900 °C for 2 h. Samples were coded based on the doping amounts as illustrated in (Table 1).
2.2 Characterizations
2.2.1 XRD analysis
Formed phases and crystalline nature of the prepared BCP powder were recognized in the absence and presence of the dopant materials utilizing XRD (BRUKER, D8 ADVANCED Cu target, Germany), employing Cu-Kα radiation of 1.5406 Å. The diffraction patterns were recorded in 2θ range of 2–90° and 2°/min scan speed. Samples were prepared for the XRD measurements by introducing small pieces of scaffolds to be fixed on a Perspex holder using special gum. As references for the identification of the designed phases, Joint Committee on Powder Diffraction Standard (JCPDS) was used. The crystallite size was calculated based on the Scherrer’s equation, the lattice parameters (a and c) and volume of the unit cell (V) were calculated according to Eqs. (1) and (2), respectively [16, 17].
2.2.2 FTIR analysis
The chemical entities of the prepared BCP were studied before and after the doping process at room temperature in wavenumber range of 400–4000 cm−1. The FTIR spectra were collected using FTIR (Demonstrate 1600, Perkin-Elmer USA). KBr was utilized as a popular alkaline halide and disc forming material for the FTIR measurements. Likewise, FTIR analysis was carried out for the samples post-immersion to check the formation of apatite layer onto the surface.
2.2.3 DSC measurements
Differential scanning calorimetry of the prepared BCP dried powder was examined in the absence and the presence of the dopant materials utilizing (SETARAM Labsys™) differential scanning calorimetry (DSC). Predetermined amount of the powder samples was fired at a heating rate of 10 °C/min in a platinum crucible up to 1000 °C.
2.2.4 Determination of surface area by BET
The surface area, total pore volume and average pore diameter of the BCP were estimated employing Brunauer–Emmett–Teller (BET) before and after doping process. 100 mg of the calcined powder was degassed and the adsorption–desorption of nitrogen was conducted at − 196 °C using a Micromeritics ASAP2020 surface area and porosity analyser (USA).
2.2.5 Zeta potential analysis
The effect of the presence of dopant materials on the charge of the prepared TCP/HA powder was studied by Zetasizer [Nano ZS, Malvern Instruments, UK]. A suspension in distilled water was prepared from the powders and forced to bath through micro-filter (0.22 µm). Sample pH was 7.4 and the measured concentration was 20 mg/ml. Filtered samples were transferred to a measuring cell (DTS1060, Malvern). For data analysis, specific software (Version 4.0) was utilized. The zeta-potential is determined by retaining the fine particles in an electric field and evaluating their mobility, using an appropriate microscopic technique. The mobility is then related to the ζ-potential at the interface using Smoluchowski equation [18].
2.2.6 Morphological properties
An important feature of the tested materials is the calcium mineralization deposition as it pledges bone formation, remodelling and tissue integration. Osteoconductivity of single and co-doped samples was scrutinized by examining the apatite forming ability on the surface of the samples upon the immersion in SBF for 15 days. Effect of doping on particle morphology and size of BCP samples were investigated utilizing SEM (JEOL JXA-840A, Electron probe micro-analyser, Japan) at 15 kv. The BCP powder surfaces were transformed into conducting surfaces through gold/palladium coating followed by the samples examination using the SEM machine. Moreover, elemental analyses (EDX) of BCP powder as well as the calcium phosphate ratios (Ca/P) were determined.
2.2.7 Mechanical haracterizationsC
Mechanical characterizations of the BCP powders were determined by measuring compression strength, stiffness and Young's modulus using universal testing machine (Zwick Roell-Z0.5 TH Mechanical Test Equipment, Germany). Each sample was pressed in triple cylinders (20 mm height and 10 mm diameter) using hydraulic press (10 tons for 1 min). The BCP cylinders were compressed after being pointed between parallel plates with a compressing velocity of 0.5 mm/min and load cell of 10 kN. The average value for each test was taken for examined samples to verify the results.
2.2.8 Dielectric properties
The dielectric parameters of BCP and doped samples as a function of frequency at room temperature were interpreted. There was a reflection of the charge carriers’ transportation causing conductivity or accumulated at the interphases in the composite materials or at the electrodes causing the electrode polarization. The real part of dielectric constant, ε', and the dielectric loss, ε'', are calculated according to Eqs. (3) and (4).
where C is the measured capacitance of the samples, d is the thickness, A is the area, ε0 is the permittivity of free space and D is the loss coefficient.
2.2.9 Antibacterial study
The lyophilized vial of every microbe, the proliferation methodology was conducted as following. Open vial as per encased directions, then at that point defrosting and aseptically move the whole substance of the vial to a container of new Mueller–Hinton stock (MHB) for microbes and potato dextrose agar (PDA) for yeast and bacteria. This was then incubated at 37 °C for 24 h for microorganisms and 25 °C for 2–3 days for growth. The microbe great development ought to be acquired in 10% glycerol vial and stirred at 80 °C. Further subcultures can be made utilizing stock and kept up with on inclines of supplement agar and potato dextrose agar then, at that point utilized as inoculum source. Stock cultures were kept at 4 °C on slants of supplement agar and potato dextrose agar. Dynamic cultures for tests were ready by moving a lapful of cells from the stock cultures to test containers of Mueller–Hinton stock (MHB) for microorganisms and Sabouraud dextrose stock (SDB) for organisms that were incubated without fomentation for 24 h at 37 °C and 25 °C separately. To 5 ml of MHB and SDB, 0.2 ml of culture was immunized and incubated till it arrived at the turbidity equivalent to that of the standard 0.5 McFarland solution at 625 nm (A = 0.08 to 0.1) which is identical to 1.5 × 108 cfu/ml.
To analyse the bacterial development, immunize 100 µl of different pathogenic microorganisms in clean cone-shaped flacon (100 ml) containing 20 ml of Muller–Hinton stock medium enhanced with 100 µl of the suspended powder tests. Inoculated cultures medium liberated from suspended powder tests under similar conditions and were utilized as a control. To keep away from expected optical obstruction during optical estimations of the inoculated cultures brought about by the light-dispersing properties of the examples particles, similar fluid medium without microorganisms was utilized, but containing similar concentration of tests particles refined under similar conditions as clear controls. Then, at that point the all cups were incubated for 24 h in hatched shaker at 200 rpm and 37 ºC. The turbidity of immunized inoculated cultures was estimated by a spectrophotometer at OD600nm (Agilent carry 100 UV/VIS Spectrometer, Range 200–800 nm, Agilent, Santa Clara, California, USA).
The antibacterial characteristics of the BCP powder were studied using shake flask method to calculate the antimicrobial activity throughout reduction % of these microorganisms after getting in contact with the test sample compared to the number of bacterial cells surviving after being in contact with the control for 24 h incubation period according to early reported research [19]. All results were expressed according to Eq. (5).
where A is the number of microorganisms present in control flask containing pure bacterial strain. B is the number of microorganisms present in shake flask after applying samples. Bacteria and yeast strains are American Type Culture Collection obtained from the culture collection of the Department of Chemistry of Natural and Microbial Products, National Research Center, Cairo, Egypt. The used organisms are gram-positive bacteria Bacillus subtilis (ATCC 6633 (Bacillus cereus (ATCC 6629) and Staphylococcus aureus (ATCC 6538). The gram-negative bacteria are Escherichia coli (ATCC25922) and Pseudomonas aeruginosa (ATCC 27853). Finally, the pathogenic yeast was Candida albicans (ATCC 10231).
2.2.10 Bioactivity in SBF
The bioactivity of BCP powder free and doped samples with Ag and Zr ions was conducted in SBF at 37 °C and pH 7.4 for 15 days. Samples were individually soaked in 100 ml of SBF in non-reactive plastic containers. At the end of the soaking period, samples were removed from SBF, washed to stop reaction, then dried at room temperature to be further analysed by FTIR and SEM coupled with EDX for the evaluation of different phases of calcium phosphate precipitations on the sample surface.
2.2.11 Cytocompatibility of the synthesized materials
The cell death mode of the selected samples TCP/HA, 4AgTCP/HA, 4ZrTCP/HA and 4AgZrTCP/HA was determined at different exposing time (25 mg disc/well) (1, 3 and 5 days) to MG-63 cells (1 × 104 cells), which were seeded on cell culture slides (SPL, South Korea). Upon finishing of the incubation periods, all slides were washed with Phosphate-Buffered Saline (PBS) and stained by ethidium bromide/acridine orange (EB/AO; 100 µg/ml of each in 100 µl PBS) for 10 min in dark and then examined under fluorescence microscope (Axio Imager Z2, Zeiss, Jena, Germany) with Fluorescent camera (20 × magnification) (AxioCam MRc3 S/N 4299, Carl Zeiss Microscopy GmbH (Jena, Germany)). The obtained images were then analysed utilizing ZEN 11 blue edition software (Zeiss, Jena, Germany). Then, the obtained data were expressed in a form of percentages of living, early apoptotic, late apoptotic and necrotic cells at each interval and compared to the untreated control cells (Table 1).
3 Results and discussion
3.1 XRD analysis
XRD pattern of BCP (Fig. 1) indexes main peaks corresponding to HA observed as sharp and narrow peaks which proved that the obtained material was in a crystalline phase (JCPDS PDF no. 09-432). This HA phase was accompanied with the appearances of β-TCP peaks at 2θ = 25.5°, 27.6°, 31.5°, 34.3°, 39.7°, 47°, 51.2°, 53.5°, 60o (JCPDSPDF no 9-169) [19, 20]. For AgTCP/HA samples, the XRD patterns (Fig. 1a) are very similar to that of BCP with little increasing in crystallite size due to the expansion of lattice parameters (a and c) as shown in Table 2 that caused by the substitution of smaller radius ion of the Ca2+ (0.099 nm) by the larger Ag+ (0.128 nm) [21]. By increasing Ag+ concentration (xAg = 0.4), new diffraction peak at 32.928° was noted which is corresponding with (JCPDS PDF no. 43-997) card of Ag2O. This suggests the formation of separated phase of Ag2O which is responsible for decreasing the crystallite size. With More increasing of Ag+ concentration (xAg = 0.8), an increasing in crystallite size was occurred due to the decreasing of the ratio of Ca/P as the amount of Ag+ ions were not doped completely into Ca2+ sides that causing higher formation of TCP %[22].
Furthermore, the XRD patterns of Zr4+ substitute TCP/HA powder samples are illustrated in Fig. 1b. There is decreasing in peak intensity as accursed for 2ZrTCP/HA sample because Zr4+ ion radius (0.084 nm) is smaller than the Ca2+ ion radius so, the crystallite size was decreased due to a contraction of lattice parameters (a and c) (Table 2). By increasing Zr4+ concentration (xZr = 0.4 and 0.8), new peak was appeared at 2θ = 30.177 and indexed as zirconia as compared to (JCPDS PDF no. 01-81-1544). Also the formation of zirconia phase influences on the TCP%: HA% due to the same reason which explained in the case of Ag ions [23, 24], therefore, the crystallite size was increased (Table 2). Moreover, the co-doping of both Ag and Zr ions is demonstrated in Fig. 1c, the peaks in the XRD patterns were matched with TCP/HA, ZrO2 and Ag2O according to the JCPDS standard patterns. As compared to undoped hydroxyapatite, the substitution of Ag+ and Zr4+ caused little decreasing in lattice parameters and crystallite size and then an increasing of both [25] due to formation of zirconia and Ag2O phases that have a notable effect on the TCP%: HA% as illustrated in Table 2. All the patterns showed diffraction peak at 26.8° which is the characteristic peak of quartz due to the presence of quartz in the phosphogypsum [26].
3.1.1 FTIR Ccharacterization
FTIR spectra of TCP/HA, AgTCP/HA and ZrTCP/HA samples (with x value = 0.2, 0.4 and 0.8) and AgZrTCP/HA (with x value = 0.2, 0.4) are similar as shown in Fig. 2a–c. All the spectra show the characteristic signature HA double bands at 605 and 560 cm−1, attributed to the ν4 vibrational mode, while the weak band at 467 cm−1 related to the ν2 vibrational mode corresponds to P–O bending. The strongest bands at 1020–1100 cm−1, that appeared as a doublet or a shoulder in all samples related to ν3 vibrational mode corresponds to P–O (asymmetrical stretching vibrational mode) [27, 28]. The existence of O–H stretching bands at 3440–2850 cm−1 confirms the presence of HA and the bands at 1630 and 1385 cm−1 correspond to O–H bending vibrational mode [29]. The absorption band at 795–800 cm−1 may be due to the presence of quartz in the samples that originated from the phosphogypsum [30]. The very weak band appeared at 1423 cm−1 corresponds to the ν3 asymmetric stretching vibrations of CO32− confirming that partially carbonated hydroxyapatite was formed during preparation [31]. The effect of Ag doping appeared at 1000–1100 cm−1 (Fig. 2a) where the intensity of bands was increased whereas the Zr doping decreases the intensity of bands at the same region (Fig. 2b). In addition, doping by Ag or Zr or co-doping by Ag and Zr ions (Fig. 2c) decreased the intensity of bands at 605 and 560 cm−1 may due to partial conversion of HA to TCP during doping process [32] as illustrated at XRD part. The characteristic bands observed in all FTIR spectra are given in Table 3.
3.2 DSC measurements
Differential scanning calorimetry of the BCP dried powder before and after doping (with x value = 0.4) was evaluated as represented in Fig. 3. All doped samples showed DSC similarity to BCP. Particularly, two main characteristic peaks were recognized in all samples, the first one is an endothermic peak in the range of 600–800 °Ccorresponding to glass transition temperature (Tg) [13]. The second one is an exothermic peak allocated at 800–850 °C, attributed to the crystallization temperature (Tc). In addition to these two main peaks, a broad peak was observed for all the samples in the range of 400–580 °C, recognized for the carbonates decomposition, confirming that partially carbonated hydroxyapatite was formed during preparation [33] and these results were consistent with the FTIR results. Comparable thermal behaviour was reported for biphasic TCP/HA sample prepared from phosphogypsum waste materials [34]. It is suggested that the dopant materials have no distinguished effect on the thermal behaviour of the Ag, Zr and co-doped BCP powder. The DSC is showing peak in the range of 850–900 °C, based on that we chose the higher temperature to ascertain the stability of the formed phases.
3.3 BET surface area measurements
The surface area of un-doped TCP/HA and doped powder with Ag and Zr (with x value = 0.4) was measured using the BET method as verified in Table 4. Surface area was improved by doping with Ag+; nevertheless, it was decreased by doping with Zr4+. This result could be explained according to different ionic radii of the dopant material, as the ionic radius of Ag+ ions (129 pm) is greater than that of Ca2+ ions (114 pm), and the substitution of the larger silver size ions has the tendency to distort the lattice parameters of the TCP/HA. Therefore, the crystal size is expected to decrease [35]. However, it was explained in the XRD result that some of Ag2O and ZrO2 were formed by the segregated parts of Ag+ and Zr4+ from the structure and that is why the expected decrease in the crystal size was not achieved. This in turn decreased the surface area measured for sample 4AgZr TCP/HA and 4 Zr TCP/HA. Santos et al. [36] concluded that even though Ag+ and Ca2+ have different ionic radii, the lodgings of Ag+ ions in the Ca2+ sites of the calcium phosphate lattice can be elucidated by the models anticipated for introducing monovalent ions. In this case, since the ionic radii of Ag+ ions are larger than that of Ca2+ ions and likewise due to the difference in their charge, the Ag+ ions could be alleviated in the calcium phosphate structure via co-substitution with the CO32− ions. If we considered that the Ag+ ions can individually reside in the positive Ca2+ sites, the essential charge balance will preserve the calcium phosphate structure neutrality [37].
On the other hand, the smaller dimension of Zr4+ ions (86 pm) compared with that of Ca2+ also influences the cell parameters in terms of a contraction; with a reduction of both a and c axes, so the doping with Zr4+ ions strongly influences the crystal size of the TCP/HA [38]. In case of AgZr, the combined effect of these ions influences the crystallinity degree of the biphasic TCP/HA and the cell parameters, where Ag+ ions influence the BCP structure with an expansion of cell parameters, especially evident for the a axis, from 9.501 Å compared to 9.424 Å for biphasic TCP/HA. Moreover, Zr4+ ions may be more associated with the β-TCP phase, as indicated by a decrease in its cell parameters: a and c axes being 10.377 and 37.167 Å, respectively, compared to 10.42 and 37.380 Å for pure β-TCP. The Zr4+ions also influence the TCP/HA structure in terms of contraction of the cell parameters and, opposite to the behaviour of Ag+. Hence, it could be concluded that, Zr4+ reduces the crystallinity degree of TCP/HA [39].
3.4 Zeta potential
To indicate the stability of particle suspensions, surface charges and particles size were measured using zeta potential for TCP/HA and Ag, Zr and Ag/Zr-doped samples (with x value = 0. 4), all zeta potential values were negative. This negative zeta potential could be an advantageous property for bone-derived TCP/HA when implanted in bone containing viable cells [40]. The lower values of zeta potential distribution of all investigated samples indicated the incipient instability of particles suspension. In Fig. 4, the obtained zeta potential values were −12.20, −9.79, −21.70 and −19.60 mV with the area % values of 100% for all the samples. The observed narrow range for zeta potential values could be responsible for the good cell viability if these samples were studied on the cells cultural or in vivo bases. In addition, the incorporation of transition metal (Ag+ and Zr4+) in the biphasic TCP/HA powder had increased the negativity of their zeta potential values especially for Zr and co-doped samples. Previous study had revealed that the presence of transition metals enhanced the proliferation of mesenchymal stem cells [41]. Materials are incipient, screening little or no repulsive force for a greater zeta potential related to a greater electrostatic repulsion between particles.
3.5 Morphological properties
In order to examine the microstructure and elemental composition of biphasic TCP/HA and those doped with Ag+ and Zr4+ (with x value = 0.4), SEM studies were performed. The SEM images and EDX spectra of the samples are presented in Fig. 5a–d. The ellipsoidal shape of the submicron particles was preserved in all samples revealing that the doping components have no influence on the surface morphology of the samples. SEM micrographs confirmed the submicron dimensions of the particles but also their tendency to agglomerate. The presence of Zr4+ ions doped into TCP/HA resulted in the fusion of the agglomerated particles. The aggregate, that is consisting of interconnected elongated spherical-like particles. The presence of Ag+ ions doped into biphasic TCP/HA improved the surface morphology and inhibited the aggregation. EDX spectra (Fig. 6e–h) of the studied samples which confirmed the presence of all constituent elements of the biphasic TCP/HA and those doped with Ag and Zr ions. It could be settled that as a result of the biomimetic coating, EDX documented calcium, phosphorous and oxygen confirm the presence of HA coating on the sample. These results suggest that Ag and Zr ions were incorporated in the biphasic TCP/HA structure. For biphasic TCP/HA, the Ca/P ratio was 1.64 which is indicative of calcium-deficient hydroxyapatite [42]. For sample doped with Ag ions, the Ca/P ratio was 1.18 which reveals the formation of amorphous calcium phosphates (ACP) and confirms the substitution of Ca ions by Ag ions inside the biphasic structure [43]. For the sample doped with Zr ions, the Ca/P ratio was 1.59 indicating the increasing of β-TCP phase in the presence of Zr ions as mentioned previously in surface area and XRD results [22]. In the case of Ag+ and Zr4+co-doping, the obtained biphasic TCP/HA ratio was affected that's why they possessed intermediate value of Ca/P (1.42) [44]. Similar results were early reported in the same line of the obtained results in the current research article [45]. The existence of HA in BCP looks to be very imperative and might play a part of a catalyser for the crystalline growth of apatite layer with hexagonal phase structure [46]. Daculsi set up that the degradation rate or resorption of the BCP ceramics in vivo can be enhanced by increasing the amount of β-TCP which is the more soluble phase [47].
3.6 Mechanical characterization
For the TCP/HA hybrid, the decreasing of the compressive strength was responsible of the HA increase, and it was appraised owing to the more brittle property of HA. The additions of ions cause alteration in the lattice. The difference could be contingent upon factors such as phases (t-ZrO2, m-ZrO2) and the particle size. The influence of doping on the different mechanical properties of TCP/HA was studied by measuring compressive strength, stiffness and Young’s modulus to reflect the changes occurred upon doping. To tolerate the low mechanical strength of BCP, it was amended with Zr4+ (with x value = 0.4) to increase the mechanical strength in the implant field [32]. Basically, in the case of co-doping [AgZrTCP/HA], the mechanical values were lower than that of the TCP/HA' one possibly due to the presence of two different doping ions inside the biphasic lattice that initiated the hindering effect on the final mechanical properties. Ag+ ions caused expansion while Zr4+ caused contraction for lattice parameters of TCP/HA, so double distortion for lattice parameters was negatively reflected on the mechanical properties. In 2020, Hu et al. decided that the mechanical enhancement mechanism of the TCP/HA composite was essentially attributed to the good amorphous calcium phosphate (ACP), HA phase dispersion and the crystallization of ACP [44]. Similar results were early reported for doping of lower density and porous ceramic materials [45]. In the case of the 4ZrTCP/HA sample, the increased intensity of t-ZrO2 and m-ZrO2 phases amended all the mechanical properties, for instance compressive strength, Young’s modulus and stiffness for this sample [46].
3.7 Dielectric properties
Dielectric measurements were used as a characterization implement to evaluate the calcium phosphate microstructure. Figure 7a–f represents the variation of ε' and ε'' with the frequency for pure TCP/HA, AgTCP/HA, ZrTCP/HA(with x value = 0.2, 0.4 and 0.8) and AgZrTCP/HA with (x value = 0.2 and 0.4). It is observed that the value of ε' and ε'' decreased by increasing the frequency for all samples. This is because of that the electric dipoles may have enough time to align themselves completely in the direction of the field during all alternation of the applied electric field; therefore dielectric constant has the maximum value at low frequencies [47]. Conversely, dielectric constant has the minimum value at high frequencies owing to these dipoles which may not follow the alternation of the field. At low frequencies, in the case of (x = 0.2) Zr or AgZr doping, both of ε' and ε'' values decreased then increased on the rise of the dopant ratio (x = 0.4) of ZrTCP/HA (Fig. 7a, d) and (x = 0.2) of AgTCP/HA (Fig. 7c, f), while values of ε' and ε'' for pure TCP/HA varies by adding Ag+ (Fig. 7b, e). On the other hand, the highest values of ε' and ε'' are recorded for 4AgZrTCP/HA while the lowest value of ε' belongs to 4ZrTCP/HA, 8AgTCP/HA and 2AgZrTCP/HA which have nearly values and the lowest value of ε'' is appropriate to 2AgZrTCP/HA. At high frequencies, the highest values of ε' and ε'' are recorded for 4AgZrTCP/HA while the lowest value of ε' belongs to 2AgZrTCP/HA and the lowest value of ε'' belongs to x = 0.2 and 0.8 for AgTCP/HA and 0.8ZrTCP/HA. Increasing the dielectric constant values by increasing concentration of dopant are due to higher dielectric polarization arising from the presence of Zr4+and Ag+ ions in TCP/HA which causes induction in electric dipoles [46]. The AC conductivity versus frequency is presented in Fig. 8. It is noticed that the conductivity remains constant at lower frequencies up to 102 Hz nearly for all samples, then its value increases by increment of frequency for all samples [48,49,50,51,52]. The enhancement in conductivity of the biphasic TCP/HA is caused by proton hopping across O2− ions or by interaction of OH− ion with the double-bonded oxygen of the PO43− group [53]. The AC conductivity of all samples is lower than that of TCP/HA except for 4AgZrTCP/HA sample which demonstrates the maximum value. Thus, 4AgZrTCP/HA increases the electrical polarization and hence causes an increase in electrical conductivity [53,54,55].
3.8 Antibacterial activity
The antibacterial activities of samples were evaluated using the shake-flask method. Antibacterial activity of the single-doped 4AgTCP/HA, 4ZrTCP/HA and co-doped 4AgZrTCP/HA samples was estimated against three types of gram-positive, two gram-negative and pathogenic yeast, in comparison with TCP/HA as a control. Based on literature, tablet (75 ± 5 mg) of each tested material was soaked in flask that contain phosphate buffer (0.3 M, pH = 7.2) was inoculated with 105 CFU ml−1 (colony-forming units per millilitre) bacteria [54]. For the single-doped 4AgTCP/HA sample, antibacterial activity towards gram negative [E. coli strains (ATCC25922)] was evaluated and its reduction % was completely inactivated after 24 h of incubation [27]. The obtained results clearly reveal that the co-doped 4AgZrTCP/HA sample possesses a strong bactericidal effect against all tested strains as it exhibits 100% reduction of bacterial strain cells after 24 h of incubation (see Table 5 and Fig. 9), compared with the single-doped samples [55, 56]. Gram-positive and Gram-negative microscopic organisms have both adversely charged cell dividers, this considers as a supposition to impact the cell wall of microscopic organisms. Submicron particles obtained in the current work, have a wide potential due to their capacity to create of ROS. The produce of ROS hinders the framework and makes mechanical harm to the bacterial cell [57, 58]. Submicron particles that possess negative charges could change the activity of the electron transport chain in microorganisms. The creation of hydrogen peroxide from the silver- or zirconium-doped surfaces is a successful method for the inhabitation of bacterial development [19, 21, 57].
3.9 Bioactivity in SBF
3.9.1 FTIR after immersion in SBF
The powder samples were investigated using FTIR and SEM coupled with EDX after 15 days of soaking in SBF. All samples were tested using FTIR spectra and the results are illustrated in Fig. 10. It is worth to highlight that the characteristic signature HA double bands at 605 and 560 cm−1, attributed to the ν4 vibrational mode of P–O became sharper and stronger for TCP/HA and all doping samples, thus the precipitation of apatite layers on the samples surface is confirmed [59, 61]. In addition, the detected band before immersion in SBF in the range of 1000–1100 cm−1 was found to be more pronounced after immersion in SBF, which also confirms the precipitation of apatite layers. Also, these bands were highly pronounced in co-doping case as noticed in Fig. 10c, thus co-doping showed significant effect on the bioactivity properties of the studied materials.
3.9.2 SEM and EDAX after immersion in SBF
Apatite formations on the surface of single-doped (4AgTCP/HA and 4ZrTCP/HA) and co-doped 4AgZrTCP/HA were detected via SEM and EDAX, as shown in Fig. 11a–d. After soaking, changes in the surface morphology were evident for all samples, due to the deposition of apatite-like layer on their surface (Fig. 11). This result was in contrast to SEM before immersion in SBF as revealed in Fig. 5. SEM images indicated that the samples were concealed with a layer of submicron-sized crystals of a typical morphology of HA after 15 days of immersion in SBF. Also, the SEM observations showed that the surface of the samples was partially covered by a dense apatite layer of tiny worm-like particles. In 2010, Wongwitwichot et al. stated that by soaking the composite, transformation from particles to tiny-like crystalline was detected. Such change was found to inspire cell attachment, migration and growth [1]. The EDAX spectra (Fig. 11e–h) of the studied samples upon immersion confirmed the presence of calcium phosphate precipitations on the surfaces of the tested samples. For the biphasic TCP/HA, the Ca/P ratio is 1.59 which is calcium-deficient hydroxyapatite. For 4AgTCP/HA, the Ca/P ratio is 1.46which indicates the formation of TCP and calcium pyrophosphate β-Ca2P2O7 [59], due to the substitution of Ca ions by Ag ions. For the single-doped 4ZrTCP/HA and co-doped 4AgZrTCP/HA samples, the Ca/P ratios are 1.77 and 1.78, respectively, which designate the formation of HA and CaO. Formerly, El-Bassyouni et al. in 2012, concluded that the composites containing zirconia have high bioactive properties as a result of the formation of Zr–OH group in SBF [24]. The higher, non-stoichiometric ratio is related to the presence of either calcium-phosphorus compounds other than hydroxyapatite with a higher Ca/P ratio or Ca compounds without phosphorus. Brushite (CaHPO4) and calcium carbonate (CaCO3) are two of the most often reported in connection with production of HA. Also, the presence of small amounts of tricalcium phosphate [Ca3(PO4)2] existed in derived HA materials [60].
3.10 Cytocompatibility of the synthesized materials
The analysis of the cells viabilities was represented in the form of mode of cell death for two time intervals 1 and 3 days after the exposure to MG63 bone-like cells in Figs. 12, 13 and 14. From Figs. 12 and 13, it could be noted that most of cell (up to 95%) is surviving after 24 h, which is very close to the normal cell viability. Increasing the incubation time to 72 h had slightly decreased the percentages of intact cell even for control (normal cells alone). However, only very little decrement for those samples doped with single dopant (up to 80%), while the sample doped with both Ag and Zr demonstrated the same cells viability compared with pure TCP/HA and control. This result highlights the advantage of doping with more than one transition metal (co-doping) over single doping, which might limit the individual cytocompatibility of each element alone. This was also affirmed by the cell mode of death (Fig. 14) that was calculated from the obtained images in Figs. 12 and 13. Most of non-viable cells were found to be on the basis of the apoptotic mode of death, which takes place by phagocytosis not the cytotoxicity of the investigated samples. These results are in the same line of previously reported researches [61, 62]. All programmed cell death modes (apoptosis, autophagy, programmed necrosis) appear normally in every cell population whatever treated or not. The judgement on the overall cell death mode depends on the prevailing cell death markers in the studied cell population. In order to study the cell death mode in every sample, a population of minimum 1000 cells were quantified, and every cell death mode marker was counted and illustrated as percentage in Fig. 14.
4 Conclusion
In the current study, BCP co-doped with both Ag and Zr, were successfully fabricated from industrial waste materials as cost-effective bone grafting materials. Because of the high biodegradability of the TCP component, the reactivity of biphasic formulations of HA with TCP increases by increasing the TCP/HA ratio. Some properties were improved for co-doped biphasic TCP/HA compared with the native and single-doped TCP/HA such as physicochemical, morphology and size parameters. Other properties were negatively affected by co-doping such as the surface area and mechanical properties. Likewise, co-doped sample possesses a strong bactericidal effect against all tested strains as it exhibits 100 reduction % of bacterial strain cells after 24 h of incubation, compared with the single-doped ones. Bioactivity properties, of single-doped 4ZrTCP/HA and co-doped 4AgZrTCP/HA samples show deposition of apatite layers on their surfaces as verified by FTIR and SEM/EDX, which suggests their capabilities in bone regeneration. The cell death mode demonstrated good viability for all samples, which increases the chances of the current developed materials to be implemented in restoration of damaged bone sites.
4.1 Significance of this study
The prepared biomaterials are considered low-cost materials as their starting materials are very cheap (industrial waste). Mass production also could be governed for the current biomaterials as they are prepared using facile and reproducible wet chemical precipitation method. If the current materials could get the required approvals in future, it is thought to be price comparable materials to the present alternative biomaterials in the market.
4.2 Future aspects
Several tests are required to get full image about the current biomaterials such as in vivo bone regeneration and biosafety tests along with visibility study to the final product.
References
P. Wongwitwichot, J. Kaewsrichan, K.H.Chua, B.H.I.Ruszymah, Comparison of TCP and TCP/HA Hybrid Scaffolds for Osteoconductive Activity. Open Biomed Eng J. 4279–285 (2010).
J.M. Sadowska, F. Wei, J. Guo, J. Guillem-Marti, Z. Lin, M-P. Ginebra, Y. Xiao, The effect of biomimetic calcium deficient hydroxyapatite and sintered β-tricalcium phosphate on osteoimmune reaction and osteogenesis, ActaBiomater., 96605–618 (2019). https://doi.org/10.1016/j.actbio.2019.06.057.
G.T. El-Bassyouni, S.H. Kenawy, R.S. Hassan, M. Mabrouk, y E.M.A. Hamzaw, Removal of 137 Cs and 152+ 154Eu Using Hydroxyapatite Prepared from Mussel Shells, Int. Ceram. 68 (6), 34–41 (2019). https://doi.org/10.1007/s42411-019-0049-0
Y. Wang, X. Ren, X. Ma, W. Su, Y. Zhang, X. Sun, X. Li, Alginate-intervened hydrothermal synthesis of hydroxyapatite nanocrystals with nanopores. Cryst. Growth Des. 15(4), 1949–1956 (2015). https://doi.org/10.1021/acs.cgd.5b00113
R.C. Richard, J. Dai, M.S. Sader, G.A. Soares, R.M.S.M. Thiré, Characterization of β-TCP, β-TCMP and BCMP Produced by Hydrolysis. Bioceram Dev Appl S, pp 11–3 (2013). https://doi.org/10.4172/2090-5025.S1-001
J. Liu, K. Li, H. Wang, M. Zhu, H. Yan, Rapid formation of hydroxyapatite nanostructures by microwave irradiation. Chem. Phys. Lett. 396(4–6), 429–432 (2004). https://doi.org/10.1016/j.cplett.2004.08.094
K.R. Mohamed, S.M. Mousa, G.T. El Bassyouni, Fabrication of nano structural biphasic materials from phosphogypsum waste and their in vitro applications. Mater. Res. Bull. 50, 432–439 (2014). https://doi.org/10.1016/j.materresbull.2013.11.023
S.M. Mousa, A. Hanna, Synthesis of nano-crystalline hydroxyapatite and ammonium sulphate from phosphogypsum waste. Mater. Res. Bull. 48(2), 823–828 (2013). https://doi.org/10.1016/j.materresbull.2012.11.067
M. Mabrouk, S.A. ElShebiney, S.H. Kenawy, G.T. El-Bassyouni, E.M.A. Hamzawy, Novel, cost-effective, Cu-doped calcium silicate nanoparticles for bone fracture intervention: Inherent bioactivity and in vivo performance. J Biomed. Mater. Res. Part B 107B, 388–399 (2019). https://doi.org/10.1002/jbm.b.341
M. Mabrouk, S.H. Kenawy, G.T. El-Bassyouni, A.A.I. Soliman, E.M.A. Hamzawy, Cancer cells treated by clusters of copper oxide doped calcium silicate, Adv. Pharm. Bull., 9(1), 102–109 (2019). https://doi.org/10.15171/apb.2019.0xx.
S.C. Veerla, D.R. Kim, J. Kim, H. Sohn, S.Y. Yang, Controlled nanoparticle synthesis of Ag/Fe co-doped hydroxyapatite system for cancer cell treatment. Mater. Sci. Eng. C 98, 311–323 (2019). https://doi.org/10.1016/j.msec.2018.12.148
S. Türk, I. Altınsoy, G. Çelebi Efe, M. Ipek, M. Özacar, et al., Biomimetic synthesis of Ag, Zn or Co doped HA and coating of Ag, Zn or Co doped HA/fMWCNT composite on functionalized Ti, Mater. Sci. Eng. C, 99, 986–998 (2019). https://doi.org/10.1016/j.msec.2019.02.025
J. Wu, K. Ueda, T. Narushima, Fabrication of Ag and Ta co-doped amorphous calcium phosphate coating films by radiofrequency magnetron sputtering and their antibacterial activity. Mater. Sci. Eng. C 109, 110599 (2020). https://doi.org/10.1016/j.msec.2019.110599
O. Kaygili and S. Keser, Zr/Mg, Zr/Sr and Zr/Zn co-doped hydroxyapatites: Synthesis and characterization, Ceram. Int., 42 (7), 9270–9273(2016). https://doi.org/10.1016/j.ceramint.2016.02.027
M. Abdellahi, A. Najfinezhad, S. Saber-Samanadari, A. Khandan, H. Ghayour, Zn and Zr co-doped M-type strontium hexaferrite: Synthesis, characterization and hyperthermia application. Chin. J. Phys. 56(1), 331–339 (2018). https://doi.org/10.1016/j.cjph.2017.11.016
O. Kaygili, S. Keser, T. Ates, A.A. Al-Ghamdi, F. Yakuphanoglu, Controlling of dielectrical and optical properties of hydroxyapatite based bioceramics by Cd content. Powder Technol, 245, 1–6 (2013). https://doi.org/10.1016/j.powtec.2013.04.012
R. AL-Wafi, R. Jafer, I.S. Yahia, A. A. Al-Ghamdi, M. A. Al-ghamdi, A.M. El-Naggar, Fast and easy synthesis of novel Strontium apatite nanostructured phase: Structure, spectroscopy, and dielectric analysis. Ceram. Int. 43, 17153–17159 (2017). https://doi.org/10.1016/j.ceramint.2017.09.137
A. Sze, D. Erickson, L. Ren, D. Li, Zeta-potential measurement using the Smoluchowski equation and the slope of the current-time relationship in electroosmotic flow. J. Colloid Interface Sci. 261(2), 402–410 (2003). https://doi.org/10.1016/S0021-9797(03)00142-5
S. Jadalannagari, K. Deshmukh, S.R. Ramanan, M. Kowshik, Antimicrobial activity of hemocompatible silver doped hydroxyapatite nanoparticles synthesized by modified sol–gel technique. Appl. Nanosci 4, 133–141 (2014). https://doi.org/10.1007/s13204-013-0197-x
K. Sinulingga, M. Sirait, N. Siregara, H. Abdullah, Synthesis and characterizations of natural limestone-derived nano hydroxyapatite (HAp): a comparison study of different metals doped Haps on antibacterial activity. RSC Adv. 11, 15896–15904 (2021). https://doi.org/10.1039/D1RA00308A
D. G. Syarif, D. H. Prajitno, A. Kurniawan,M. B. Febrian, R. Lesmana, Hydrothermally synthesis and characterization of HAp and Zr-doped HAp nanoparticles from bovine bone and zircon for photodynamic therapy, Process. Appl. Ceram., 15 (2), 146–153 (2021). https://doi.org/10.2298/PAC2102146S
S.F. Mansour, S.I. El-dek, M.K. Ahmed, Physico-mechanical and morphological features of zirconia substituted hydroxyapatite nano crystals. Sci. Rep. 7, 43202 (2017). https://doi.org/10.1038/srep43202
R. K. Chadha, A. P. Singh, K. L. Singh, C. Sharma, V. Naithani, Influence of microwave processing and sintering temperature on the structure and properties of Sr/Zr doped hydroxyapatite, Mater. Chem. Phys. https://doi.org/10.1016/j.matchemphys.2018.09.086
S. Helen, and A.R. Kumar, Microhardness of Hydroxyapatite Doped ZrO2 using Sol-Gel Method. MMSE, April ISSN 2412–5954(2017). https://doi.org/10.2412/mmse.48.98.680.
C. Fu, X. Zhang, K. Savino, P. Gabrys, Y. Gao, W. Chaimayo, B.L. Miller, M.Z. Yates, Antimicrobial silver-hydroxyapatite composite coatings through two-stage electrochemical synthesis. Surf. Coat. Technol. 301, 13–19 (2016). https://doi.org/10.1016/j.surfcoat.2016.03.010
S.H. Daryan, J. Javadpour, A. Khavandi, M. Erfan, Morphological evolution on the surface of hydrothermally synthesized hydroxyapatite microspheres in the presence of EDTMP. Ceram. Int. 44(16), 19743–19750 (2018). https://doi.org/10.1016/j.ceramint.2018.07.229
D. Siek, A. Ślósarczyk, A. Przekora, A. Belcarz, A. Zima, G. Ginalska, J. Czechowska, Evaluation of antibacterial activity and cytocompatibility of α-TCP based bone cements with silver-doped hydroxyapatite and CaCO3. Ceram. Int. 43(16), 13997–14007 (2017). https://doi.org/10.1016/j.ceramint.2017.07.131
J. Vecstaudza, M. Gasik, J. Locs, Amorphous calcium phosphate materials: Formation, structure and thermal behavior. J. Eur. Ceram. Soc. 39, 1642–1649 (2019). https://doi.org/10.1016/j.jeurceramsoc.2018.11.003
V. Stanic, D. Janackovic, S. Dimitrijevic, B.S. Tanaskovic, M. Mitric, S.M. Pavlovic, A. Krstic, D. Jovanovic, S. Raicevic, Synthesis of antimicrobial monophase silver—doped hydroxyapatite nanopowders for bone tissue engineering. Appl. Surf. Sci. 257, 4510–4518 (2011). https://doi.org/10.1016/j.apsusc.2010.12.113
R. Ravisankar, S.Kiruba, P.Eswaran, G. Senthilkumarand A.Chandrasekaran, Mineralogical Characterization Studies of Ancient Potteries of Tamilnadu, India by FT-IR Spectroscopic Technique, J. Chem., 7(S1), S185–S190 (2010). https://doi.org/10.1155/2010/643218
S. Hiromoto, M. Inoue, T. Taguchi, M. Yamane, N. Ohtsu, In vitro and in vivo biocompatibility and corrosion behaviour of a bioabsorbable magnesium alloy coated with octacalcium phosphate and hydroxyapatite. Actabiomat. 11, 520–530 (2015). https://doi.org/10.1016/j.actbio.2014.09.026
C. L. Popa, C. S. Ciobanu, G. Voicu, E. Vasile, M. C. Chifiriuc, S. L. Iconaru and Daniela Predoi, Influence of thermal treatment on the antimicrobial activity of silver-doped biological apatite, Nanoscale Res. Lett., 10, 502–512 (2015). https://doi.org/10.1186/s11671-015-1211-x
H.H. Beherei, K.R. Mohamed, G.T. El-Bassyouni, Mechanical and bioactivity properties of nano ceramic composite-based oxyapatite materials. Int. Ceram. 07–08, 386–392 (2014). https://doi.org/10.1007/BF03401090
S. Türk, I. Altınsoy, G.C. Efe, M. Ipek, M. Özacar, C. Bindal, Biomimetic synthesis of Ag, Zn or Co doped HA and coating of Ag, Zn or Co doped HA/fMWCNT composite on functionalized Ti. Mater. Sci. Eng. C 99, 986–998 (2019). https://doi.org/10.1016/j.msec.2019.02.025
M. Roy, A. Bandyopadhyay, S. Bose, Chapter 6—Ceramics in Bone Grafts and Coated Implants. Materials for Bone Disorders, pp 265–314 (2017). https://doi.org/10.1016/B978-0-12-802792-9.00006-9
S. Jayakumar, P.V. Ananthapadmanabhan, K. Perumal, T.K. Thiyagarajan, S.C. Mishra, L.T. Su, A.I.Y. Tok, J. Guo, Characterization of nano-crystalline ZrO2 synthesized via reactive plasma processing. Mater. Sci. Eng: B 176(12), 894–899 (2011). https://doi.org/10.1016/j.mseb.2011.05.013
B. Aksakaland, Demirel M., The effect of Zirconia/Yttria/Silver substitutions on mechanostructure and cell viability of the synthesized bioceramic bone grafts. Ceram. Int., 43(10), 7482–7487 (2017). https://doi.org/10.1016/j.ceramint.2017.03.026
M. Šupová, Substituted hydroxyapatites for biomedical applications: a review. Ceram. Int. 41(8), 9203–9231 (2015). https://doi.org/10.1016/j.ceramint.2015.03.316
E. Landi, A. Tampieri, G. Celotti, S. Sprio, Densification behaviour and mechanisms of synthetic hydroxyapatites. J Eur Ceram Soc. 20(14–15), 2377–2387 (2000). https://doi.org/10.1016/S0955-2219(00)00154-0
I.M.G. Santos, L.S.N.S. Barbosa, C.X. Resende, G.A. Soares, E.A. Santos, Crystallographic aspects regarding the insertion of ag+ ions into a hydroxyapatite structure. Mater. Res. 18(4), 881–890 (2015). https://doi.org/10.1590/1516-1439.012515
E. Boanini, M. Gazzano, A. Bigi, Ionic substitutions in calcium phosphates synthesized at low temperature. Acta Biomater. 6(6), 1882–1894 (2010). https://doi.org/10.1016/j.actbio.2009.12.041
K.R. Mohamed, H.H. Beherei, G.T. El Bassyouni, N. El Mahallawy, Fabrication and mechanical evaluation of hydroxyapatite/oxide nano-composite materials,Mater. Sci. Eng. C,33 4126–4132 (2013). https://doi.org/10.1016/j.msec.2013.05.059
H. Zhou, S. Kong, S.B. Bhaduri, L. Deng, Preparation of calcium phosphates with negative zeta potential using sodium calcium polyphosphate as a precursor. Mater. Lett. 156, 79–81 (2015). https://doi.org/10.1016/j.matlet.2015.04.116
A. Fahami, G.W. Beall, Mechanosynthesis of carbonate doped chlorapatite-ZnO nanocomposite with negative zeta potential. Ceram. Int. 41, 12323–12330 (2015). https://doi.org/10.1016/j.ceramint.2015.06.061
X. Wang, J.T. Li, M.Y. Xie, L.J. Qu, P. Zhang, X.L. Li, Structure, mechanical property and corrosion behaviours of (HA + β-TCP)/Mg-5Sn composite with interpenetrating networks. Mater. Sci. Eng. C 56, 386–392 (2015). https://doi.org/10.1016/j.msec.2015.06.047
H.H. Ahmed, H.A. Aglana, M. Mabrouk, A.A. Abd-Rabou, H.H. Beherei, Enhanced mesenchymal stem cell proliferation through complexation of selenium/titanium nanocomposites. J. Mater. Sci.: Mater. in Med., 30(2), 24 (2019). https://doi.org/10.1007/s10856-019-6224-z.
S. Adzila, M.C. Murad, I. Sopyan, Doping metal into calcium phosphate phase for better performance of bone implant materials. Recent Patents Mater. Sci. 5, 18–47 (2012). https://doi.org/10.2174/1874464811205010018
P. Sikder, Y. Ren, S.B. Bhaduri, Microwave processing of calcium phosphate and magnesium phosphate based orthopedic bioceramics: A state-of-the-art review. Acta Biomater. 111, 29–53 (2020). https://doi.org/10.1016/j.actbio.2020.05.018
X. Hu, W. Zhang, D. Hou, Synthesis, microstructure and mechanical properties of tricalcium phosphate-hydroxyapatite (TCP/HA) composite ceramic. Ceram. Int. 46, 9810–9816 (2020). https://doi.org/10.1016/j.ceramint.2019.12.254
F. De Groot-Barrere, V. Miegem, H. Yuan, J. Debruijn, Method for producing an osteoinductive calcium phosphate and products thus obtained. Patent No. P100479 (2015).
G. Daculsi, Biphasic calcium phosphate concept applied to artificial bone, implant coating and injectable bone substitute, Biomaterials 16, 1473–1478 (1998). https://doi.org/10.1016/s0142-9612(98)00061-1.
R.J. Darby, I. Farnan, R.V. Kumar, Method for making minor dopant additions to porous ceramics. Adv. App. Ceram. 108(8), 506–508 (2009). https://doi.org/10.1179/174367609X459531
A. Sakthisabarimoorthi, S.A. Martin BrittoDhas, M. Jose, Fabrication of monodispersed α-Fe2O3@SiO2 core-shell nanospheres and investigation of their dielectric behavior. J. Alloys Compd., 771, 1–8 (2019). https://doi.org/10.1016/j.jallcom.2018.08.250
R. AL-Wafi, R. Jafer, I.S. Yahia, A.A. Al-Ghamdi, M.A. Al-ghamdi, A.M. El-Naggar, Fast and easy synthesis of novel Strontium apatite nanostructured phase: Structure, spectroscopy, and dielectric analysis, Ceram. Int., 43(18), 17153–17159 (2017). https://doi.org/10.1016/j.ceramint.2017.09.137
K.K. Bamzai, S. Suri, V. Singh, Synthesis, characterization, thermal and dielectric properties of pure and cadmium doped calcium hydrogen phosphate. Mater. Chem. Phys. 135(1), 158–167 (2012). https://doi.org/10.1016/j.matchemphys.2012.04.040
R.K. Saini, L.P. Bagri, A.K. Bajpai, Nano-silver hydroxyapatite based antibacterial 3D scaffolds of gelatin/alginate/poly (vinyl alcohol) for bone tissue engineering applications. Colloids Surf. B, 177, 211–218 (2019). https://doi.org/10.1016/j.colsurfb.2019.01.064
A. Krzywicka, E. Megiel, Silver-Polystyrene (Ag/PS) Nanocomposites Doped with Polyvinyl Alcohol (PVA)—fabrication and bactericidal activity. Nanomaterials 10, 2245 (2020). https://doi.org/10.3390/nano10112245
X. Shen, Y. Zhang, P. Ma, L. Sutrisno, Z. Luo, Y. Hu, Y. Yu, B. Tao, C. Li, K. Cai, Fabrication of magnesium/zinc-metal organic framework on titanium implants to inhibit bacterial infection and promote bone regeneration. Biomaterials 212, 1–16 (2019). https://doi.org/10.1016/j.biomaterials.2019.05.008
S. Raynaud, E. Champion, J.P. Lafon, D. Bernache-Assollant, Calcium phosphate apatite with variable Ca/P atomic ratio III. Mechanical properties and degradation in solution of hot pressed ceramics, Biomaterials 23(4), 1081–1089 (2002). https://doi.org/10.1016/S0142-9612(01)00220-4
S. Sáchez-Salcedo, F. Balas, I. Izquierdo-Barba, M. Vallet-Regí, In vitro structural changes in porous HA/β-TCP scaffolds in simulated body fluid, ActaBiomater. 5, 2738–2751 (2009). https://doi.org/10.1016/j.actbio.2009.03.025.
B. Gunay, E. Sarıyar, U. Unal, Z. F. Karagonlar, Ö. Sağlam, Upconversion properties of Tm3+-Er3+ co-doped layered perovskites and in-vitro cytotoxicity of their exfoliated nanomaterials, Colloids Surf. A: Physicochem. Eng. Asp, 612126003(2021). https://doi.org/10.1016/j.colsurfa.2020.126003
S. Chen, M. Michálek, D. Galusková, M. Michálková, P. Švančárek et al., Multi-targeted B and Co co-doped 45S5 bioactive glasses with angiogenic potential for bone regeneration. Mater. Sci. Eng. C 112, 110909 (2020). https://doi.org/10.1016/j.msec.2020.110909
Author information
Authors and Affiliations
Corresponding author
Additional information
Publisher's Note
Springer Nature remains neutral with regard to jurisdictional claims in published maps and institutional affiliations.
Supplementary Information
Below is the link to the electronic supplementary material.
Rights and permissions
About this article
Cite this article
Mabrouk, M., Mousa, S.M., ElGhany, W.A.A. et al. Bioactivity and cell viability of Ag+- and Zr4+-co-doped biphasic calcium phosphate. Appl. Phys. A 127, 948 (2021). https://doi.org/10.1007/s00339-021-05051-1
Received:
Accepted:
Published:
DOI: https://doi.org/10.1007/s00339-021-05051-1