Abstract
Use of many pesticide products poses the problem of their effects on environment and health. Amongst them, the effects of glyphosate with its adjuvants and its by-products are regularly discussed. The aim of the present study was to shed light on the real impact on biodiversity and ecosystems of Roundup®, a major herbicide used worldwide, and the glyphosate it contains, by the study of their effects on growth and viability of microbial models, namely, on three food microorganisms (Geotrichum candidum, Lactococcus lactis subsp. cremoris and Lactobacillus delbrueckii subsp. bulgaricus) widely used as starters in traditional and industrial dairy technologies. The presented results evidence that Roundup® has an inhibitory effect on microbial growth and a microbicide effect at lower concentrations than those recommended in agriculture. Interestingly, glyphosate at these levels has no significant effect on the three studied microorganisms. Our work is consistent with previous studies which demonstrated that the toxic effect of glyphosate was amplified by its formulation adjuvants on different human cells and other eukaryotic models. Moreover, these results should be considered in the understanding of the loss of microbiodiversity and microbial concentration observed in raw milk for many years.
Similar content being viewed by others
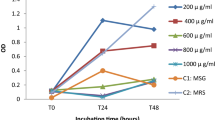
Explore related subjects
Discover the latest articles, news and stories from top researchers in related subjects.Avoid common mistakes on your manuscript.
Introduction
Any modification of the environment which leads to a response by living organisms may be considered as a stress [1]. Stresses affecting biological functions are classified according to two categories: (i) abiotic including physical and chemical stresses, and (ii) biotic. Furthermore, the stress response in biology, actually considered as a global phenomenon, can be extended to anthropogenic pressure such as genetic engineering or xenobiotics (including Roundup) pollution [2].
The necessity for better control of food microorganisms, which are widely used in traditional and industrial dairy technologies, requires knowledge concerning their response to numerous stresses [3]. Amongst the high number of food microorganisms found in raw or fermented milk [4], some of them, such as Lactobacillus delbrueckii subsp. bulgaricus (L. bulgaricus), Lactococcus lactis subsp. cremoris (L. cremoris) and Geotrichum candidum (G. candidum) have been deeply studied at the stress response level [5, 6], but, to our knowledge, not when the stress is induced by herbicide exposures.
The lactic acid L. bulgaricus is a starter lactic acid bacteria (LAB) that is commonly used in fermented food technology [4, 7]. Besides its primary function to produce lactic acid during the fermentation process, L. bulgaricus also contributes in the proper development of both flavour (production of aromatics compounds such as acetoïne and acetaldehyde) and texture (polysaccharides synthesis) [8, 9]. Amongst the LAB, L. lactis plays the role of model besides its role as major contributor in cheese processing [4]. Most of the food starters are based on this species which includes many subspecies and strains including the cremoris subspecies. The most important properties are the ability to produce acid in milk and to convert milk proteins to flavour components. G. candidum is a deuteromycete fungus whose teleomorph state is Galactomyces geotrichum [10, 11]. It is found in raw milk and is widely used for its major application as a ripening agent in cheese-making [12]. In France, G. candidum is the dominant yeast-like fungus from smear-ripened soft cheese and mould-ripened cheese in which it forms a substantial part of the microflora [4, 13].
It is estimated that at least 99% of the microorganisms on earth are not cultivable using current technologies. Paradoxically enough, this tremendous uncontrolled microbiodiversity could be the reason for the lack of interest in the endangered bacteria hypothesis [14]. While plant and animal preservation are approached both in situ and ex situ, preservation of microorganisms is managed only ex situ [15]. Studies on the distribution of subspecies within the species L. cremoris, have shown the difficulty of isolating the cremoris subspecies from the dairy environment in some geographic areas. Some hypothetical intrinsic and extrinsic causes can be proposed to analyse this type of rarefaction. Over the past few decades, the environment of L. cremoris has been drastically modified by human activity, giving rise firstly to anthropogenic stress for instance pesticides and secondly to biodiversity reduction (plant varieties in pasture) [14].
Roundup is the major herbicide used worldwide partly because 70% of GM food plants are Roundup-tolerant [16]. Roundup formulations contain glyphosate, claimed active component, and also adjuvants like polyethoxylated tallowamine (POEA) for a better stability and penetration by modifying the physicochemical properties of formulations [17].
Glyphosate and AMPA (aminomethyl phosphonic acid), its main metabolite, are amongst the first major pollutants of surface waters [18]. Roundup reduces the biodiversity of species in the aquatic community [19]. Currently, the maximum tolerated glyphosate residue in milk in Europe is 0.05 mg/kg or 0.05 ppm [20]. Glyphosate is found in humans at the ppm level [21]. Some of us have previously observed that Roundup is cytotoxic to human placental, embryonic kidney and liver cell lines at very low sub-agricultural dilutions [22–25].
In vivo, effects of Roundup on male reproductive system of ducks and rabbits [26, 27] and liver of rats [28, 29] have been observed. It is known that Roundup has negative effects on fauna and flora at different qualitative and quantitative levels. In the sea urchin egg, cell cycle alteration and inhibition of transcription were observed [30, 31].
In microbiology, it was reported that glyphosate affects growth and metabolism of Saccharomyces cerevisiae [32]. Moreover, glyphosate is more toxic in distilled water than in seawater on prokaryotic bacteria Vibrio fischeri [33]. Also, Tetrahymena pyriformis a eukaryotic cilli protozoan is less sensitive to glyphosate and AMPA than V. fischeri [34].
The work described in the present article was carried out on three microorganisms of food interest, to highlight the impact of the Roundup herbicide and of glyphosate on food microbial world. The need to study and preserve the microbial diversity in dairy ecosystems (i.e. raw milk, cheese and yogurt) is of major interest for future developments in food processing.
Materials and Methods
Strains and Culture Conditions
Experiments were performed with G. candidum ATCC 204307, Lactobacillus delbrueckii subsp. bulgaricus CFL1 and Lactococcus lactis subsp. cremoris ATCC 19257. G. candidum was cultured in MSF, pH 5.6; L. lactis in M17 containing lactose, pH 7.1 (AES Laboratoire, Combourg, France); and L. bulgaricus in MRS, pH 6.4 (AES Laboratoire). Cultures recovered in stationary phase of growth were used to inoculate new media at 2% (volume of liquid culture/volume of culture broth). The initial cell concentrations before treatments are 1.95.104 ± 0.36, 6.31105 ± 2.03 and 6.70.108 ± 2.52 UFC/ml for G. candidum, L. bulgaricus and L. cremoris, respectively.
Roundup and Glyphosate Treatments
Roundup R400 (400 g/l of glyphosate) and R450 (450 g/l of glyphosate) (Monsanto, Anvers, Belgium) were diluted in autoclaved culture media, pH adjusted to each medium and 0.2 μm filtered. A solution of glyphosate (Sigma-Aldrich, Saint-Quentin Fallavier, France), equivalent in glyphosate concentration and pH to R450, was diluted in different media.
Turbidimetry Measurement and Colony Counting
Experiments were performed with 96-well plates. The minimal inhibitory concentration (MIC) was evaluated, after treatment, by turbidimetry measurement at 600 nm using a microplate reader (Metertech Σ960, Taipei, Taiwan). The minimal microbicide concentration (MMC) which corresponds to the minimal treatment leading to 99.99% of lethality was evaluated by colony counting, after plating the previously treated micro-organisms. Concentrations between the MIC value and the MMC value correspond to cells that do not growth but are not dead. For these experiments, it is not necessary to wash the cells before plating. The final dilution is so important that the herbicide residues are then negligible.
Colonies Observations
Each microorganism was plated on Petri dishes containing agar and Roundup, and then incubated during 48 h. Colonies were macroscopically observed using a Canon EOS 350D camera and microscopically using a Leica DMLB microscope (magnification ×500 for G. Candidum and ×1,000 for L. bulgaricus and L. cremoris) after coloration with cotton blue for G. candidum and methylene blue for the two bacteria.
Statistical Analysis
Data were expressed as mean ± SEM for three independent determinations (n = 9). Significant differences were determined by Student t test with P < 0.05*, P < 0.01** and P < 0.001***.
Results
Our first observation is the relatively comparable toxicity profiles on three essential food microorganisms, in a short 24-h period (Fig. 1a–c). Roundup is always more potent than glyphosate, and in all cases, toxic from levels 10–100 times below the lowest agricultural uses (10,000 ppm). G. candidum and L. cremoris are more Roundup sensitive than L. bulgaricus. Similar impacts have not been observed for glyphosate alone (except for G. candidum at 10,000 ppm). Roundup effect was not proportional to glyphosate concentration in the Roundup formulation, since R400 is almost 10-fold more inhibitory than R450 (Fig. 1a). A specific biphasic inhibitory effect was transiently observed at low doses (around 100 ppm) for L. cremoris, and possibly for G. candidum. Microbicide effect of Roundup was obtained at concentrations 1.2, 1.6 and 2 times higher than the measured MICs for L. bulgaricus, G. candidum and L. cremoris, respectively (Table 1). The macroscopic observations (Fig. 2) showed differences in sensitivity between the three micro-organisms: L. cremoris, G. candidum and L. bulgaricus, and no colonies were observed at 200, 1,000 and 2,000 ppm of Roundup, respectively. Considering the size, colonies of G. candidum and L. bulgaricus decrease depending on the concentration of Roundup (Fig. 2g, i) present. Microscopically, no significant differences were observed between controls and treated colonies. However, it seems that cell contents of L. bulgaricus leaks out (Roundup 1,000 ppm; Fig. 2l).
Growth inhibition of the three microorganisms (a Geotrichum candidum, b Lactococcus lactis subsp. cremoris and c Lactobacillus delbrueckii subsp. bulgaricus) after 24 h of incubation in growth media supplemented with Roundup (grey circles 400 and black circles 450) or equivalent amount of glyphosate (triangles) evaluated by turbidimetry (600 nm). The herbicides' concentrations are up to the lowest agricultural uses (10,000 ppm). SEMs are shown in all instances (n = 3); Student test (P < 0.05*; P < 0.01** and P < 0.001***)
Macroscopic (a–c, and g–i) and microscopic (d–f and j–l) observations of Geotrichum candidum, Lactococcus lactis subsp. cremoris and Lactobacillus delbrueckii subsp. bulgaricus, after 48 h of incubation on solid growth media supplemented with Roundup (500 ppm for g and j, 100 ppm for h and k, 1,000 ppm for i and l) or without (for a, d, b, e, c and f). The herbicide concentrations are up to the lowest agricultural uses (10,000 ppm)
Discussion
The relatively comparable toxicity profiles on these three essential food microorganisms is surprising since the fungus is phylogenetically far from the other two [35]. It has previously been shown that glyphosate and Roundup are toxic and can reduce fungal growth at concentrations above 10 ppm for mycorrhizal fungi (Hebeloma crustuliniforme, Laccaria laccata, Thelephora americana, T. terrestris and Suillus tomentosus) [36]. Similarly, some adverse effects were observed at concentrations below those found in the soil after typical applications. Moreover, differences in the sensitivities of the different species have also been observed towards the herbicide [36, 37]. The inhibitory and microbicide effects observed at agricultural sub-doses in this work are consistent with those previously observed in fungi [36, 37]. Glyphosate is believed to be the major active principle in the herbicide through its inhibition of EPSPS. Glyphosate metabolism varies by organism [38]. Glyphosate blocks EPSPS (5-enolpyruvylshikimate-3-phosphate synthase), a key enzyme involved in the biosynthesis of aromatic amino acids, naturally present in plants, fungi and some bacteria. EPSPS, which exists in two classes—glyphosate-sensitive and glyphosate-tolerant—is essential in plants for protein production via the shikimic acid pathway [39–41]. There could be an enzyme highly tolerant to glyphosate, which would have some genetical homologies with the first and/or the second classes of this enzyme [42, 43]. This enzyme is absent in mammals. However, other inhibition pathways are involved, like Cyp450 aromatase inhibition, since glyphosate is weakly responsible of the cytotoxicity on eukaryotic cells (human placental, embryonic or umbilical cord cells) [22, 23, 25] and cellular endocrine disruption [24]. This is in agreement with the lethality observed on the three food microorganisms studies in the present work. Moreover, like on eukaryotic cells, impact of glyphosate is not proportional to its concentration in Roundup formulations, confirming adjuvants are not inert—an observation that supports the findings of previous studies [23, 25]. Similar effects on microorganisms have been reported previously in the literature; in fact, it appeared that Ichthyophthirius multifiliis and T. thermophila tolerate glyphosate but not Roundup. The commercial formulation was then 100 times more toxic than the active ingredient (G) [44]. Amongst these adjuvants, POEA which promotes a xenobiotic penetration into cells, is more toxic than glyphosate [23]. Microorganisms studied here are 10 times less sensitive than human embryonic, placental and hepatic cells. At non-toxic concentrations, some endocrine biphasic disrupting effects have already been observed in human cells with Roundup [24]. This could be the case of a receptor-mediated phenomenon with a stimulation followed by internalization and desensitization. Thus, if there is a stress reaction widely distributed in evolution, we could suggest that biphasic inhibitory effects at non-toxic doses of Roundup and glyphosate on L. cremoris and probably G. candidum could be receptor-mediated.
In conclusion, the pesticide Roundup sprayed on Roundup tolerant GMOs and on non-agricultural soils could thus impact on specific microbiodiversity including food interest microorganisms. This is illustrated by the fact that actual food processing requires industrial food starters for milk fermentation. Furthermore, unpredictable consequences of Roundup on soil microorganisms have to be considered.
References
Missous G, Thammavongs B, Dieuleveux V et al (2007) Improvement of the cryopreservation of the fungal starter Geotrichum candidum by artificial nucleation and temperature downshift control. Cryobiology 55:66–71
Thammavongs B, Denou E, Missous G et al (2008) Response to environmental stress as a global phenomenon in biology: the example of microorganisms. Microbes Environ 23:20–23
Yousef A, Juneja VK (2003) Microbial stress adaptation and food safety. CRC Press, Boca Raton
Wouters JTM, Ayad EHE, Hugenholtz J et al (2002) Microbes from raw milk for fermented dairy products. Int Dairy J 12:91–109
Denou E, Thammavongs B, Gueguen M et al (2005) Interspecies protection against freezing stress within a food microbial community. Cell Preserv Technol 3:75–83
Thammavongs B, Poncet JM, Desmasures N et al (2004) Resin straw as an alternative system to securely store frozen microorganisms. J Microbiol Methods 57:181–186
Beresford TP, Fitzsimons NA, Brennan NL et al (2001) Recent advances in cheese microbiology. Int Dairy J 11:259–274
Petry S, Furlan S, Crepeau MJ et al (2000) Factors affecting exocellular polysaccharide production by Lactobacillus delbrueckii subsp. bulgaricus grown in a chemically defined medium. Appl Environ Microbiol 66:3427–3431
Roissart de H, Luquet FM (Eds.) (1994) Bactéries lactiques: aspects fondamentaux et technologiques. Uriage, France
Smith MT, Poot GA, de Cock AW (2000) Re-examination of some species of the genus Geotrichum Link: Fr. Antonie Van Leeuwenhoek 77:71–81
Ueda-Nishimura K, Mikata K (2000) Two distinct 18S rRNA secondary structures in Dipodascus (Hemiascomycetes). Microbiology 146(Pt 5):1045–1051
Guéguen M, Schmidt J (1992) Les germes utiles: Les levures et Geotrichum candidum, Paris, France
Prillinger H, Molnar O, Eliskases-Lechner F et al (1999) Phenotypic and genotypic identification of yeasts from cheese. Antonie Van Leeuwenhoek 75:267–283
Panoff JM, Desmasures N, Thammavongs B et al (2002) In situ protection of microbiodiversity is under consideration. Microbiology 148:625
Chamba JF, Beal C, Delbes C et al (2005) Sauvegarde de consortia microbiens fromagers : Mise au point de méthodes de conservation ex situ à long terme. Les Actes du BRG 5:393–409
Clive J (2009) Global status of commercialized Biotech/GM crops, ISAAA Brief 41
Cox C (2004) Herbicide factsheet—Glyphosate. J Pest Reform 24:10–15
IFEN (2006) Report on pesticides in waters. Data 2003–2004
Relyea RA (2005) The impact of insecticides and herbicides on the biodiversity and productivity of aquatic communities. Ecol Appl 15:618–627
EFSA (2009) Modification of the residue definition of glyphosate in genetically modified maize grain and soybeans, and in products of animal origin on request from the European Commission. EFSA J 7:42
Acquavella JF, Alexander BH, Mandel JS et al (2004) Glyphosate biomonitoring for farmers and their families: results from the Farm Family Exposure Study. Environ Health Perspect 112:321–326
Benachour N, Sipahutar H, Moslemi S et al (2007) Time- and dose-dependent effects of roundup on human embryonic and placental cells. Arch Environ Contam Toxicol 53:126–133
Benachour N, Séralini GE (2009) Glyphosate formulations induce apoptosis and necrosis in human umbilical, embryonic, and placental cells. Chem Res Toxicol 22:97–105
Gasnier C, Dumont C, Benachour N et al (2009) Glyphosate-based herbicides are toxic and endocrine disruptors in human cell lines. Toxicology 262:184–191
Richard S, Moslemi S, Sipahutar H et al (2005) Differential effects of glyphosate and roundup on human placental cells and aromatase. Environ Health Perspect 113:716–720
Oliveira AG, Telles LF, Hess RA et al (2007) Effects of the herbicide Roundup on the epididymal region of drakes Anas platyrhynchos. Reprod Toxicol 23:182–191
Yousef MI, Salem MH, Ibrahim HZ et al (1995) Toxic effects of carbofuran and glyphosate on semen characteristics in rabbits. J Environ Sci Health B 30:513–534
Benedetti AL, Vituri Cde L, Trentin AG et al (2004) The effects of sub-chronic exposure of Wistar rats to the herbicide Glyphosate-Biocarb. Toxicol Lett 153:227–232
Beuret CJ, Zirulnik F, Gimenez MS (2005) Effect of the herbicide glyphosate on liver lipoperoxidation in pregnant rats and their fetuses. Reprod Toxicol 19:501–504
Marc J, Mulner-Lorillon O, Boulben S et al (2002) Pesticide Roundup provokes cell division dysfunction at the level of CDK1/cyclin B activation. Chem Res Toxicol 15:326–331
Marc J, Le Breton M, Cormier P et al (2005) A glyphosate-based pesticide impinges on transcription. Toxicol Appl Pharmacol 203:1–8
Braconi D, Sotgiu M, Millucci L et al (2006) Comparative analysis of the effects of locally used herbicides and their active ingredients on a wild-type wine Saccharomyces cerevisiae strain. J Agric Food Chem 54:3163–3172
Hernando MD, De Vettori S, Martinez Bueno MJ et al (2007) Toxicity evaluation with Vibrio fischeri test of organic chemicals used in aquaculture. Chemosphere 68:724–730
Bonnet JL, Bonnemoy F, Dusser M et al (2007) Assessment of the potential toxicity of herbicides and their degradation products to nontarget cells using two microorganisms, the bacteria Vibrio fischeri and the ciliate Tetrahymena pyriformis. Environ Toxicol 22:78–91
Pace NR (2001) The universal nature of biochemistry. Proc Natl Acad Sci USA 98:805–808
Chakravarty P, Sidhu SS (1987) Effect of glyphosate, hexazinone and triclopyr on in vitro growth of five species of ectomycorrhizal fungi. Eur J For Pathol 17:204–210
Estok D, Freedman B, Boyle D (1989) Effects of the herbicides 2,4-d, glyphosate, hexazinone, and triclopyr on the growth of three species of ectomycorrhizal fungi. Bull Environ Contam Toxicol 42:835–839
Williams GM, Kroes R, Munro IC (2000) Safety evaluation and risk assessment of the herbicide Roundup and its active ingredient, glyphosate, for humans. Regul Toxicol Pharmacol 31:117–165
Barry G, Kishore G, Padgette S et al (1992) Inhibitors of amino acid biosynthesis: strategies for imparting glyphosate tolerance to crop plants. Curr Top Plant Physiol 7:139–145
Fitzgibbon JE, Braymer HD (1990) Cloning of a gene from Pseudomonas sp. strain PG2982 conferring increased glyphosate resistance. Appl Environ Microbiol 56:3382–3388
Priestman MA, Funke T, Singh IM et al (2005) 5-Enolpyruvylshikimate-3-phosphate synthase from Staphylococcus aureus is insensitive to glyphosate. FEBS Lett 579:728–732
Liu Z, Lu W, Chen M et al (2006) Genetic analysis of glyphosate tolerance in Halomonas variabilis strain HTG7. World J Microbiol Biotechnol 22:681–686
Reis LF, Van Sluys MA, Garratt RC et al (2006) GMOs: building the future on the basis of past experience. An Acad Bras Cienc 78:667–686
Everett KD, Dickerson HW (2003) Ichthyophthirius multifiliis and Tetrahymena thermophila tolerate glyphosate but not a commercial herbicidal formulation. Bull Environ Contam Toxicol 70:731–738
Acknowledgments
The authors thank J.-M. Bré for his technical assistance during the experimentation. This work was supported by the Ministère de l’Enseignement Supérieur et de la Recherche. E. C. holds a fellowship from the Conseil Régional de Basse-Normandie and CRIIGEN (Committee for Independent Research and Information on Genetic Engineering). The authors would also like to thank Testbiotech for their support.
Author information
Authors and Affiliations
Corresponding author
Rights and permissions
About this article
Cite this article
Clair, E., Linn, L., Travert, C. et al. Effects of Roundup® and Glyphosate on Three Food Microorganisms: Geotrichum candidum, Lactococcus lactis subsp. cremoris and Lactobacillus delbrueckii subsp. bulgaricus . Curr Microbiol 64, 486–491 (2012). https://doi.org/10.1007/s00284-012-0098-3
Received:
Accepted:
Published:
Issue Date:
DOI: https://doi.org/10.1007/s00284-012-0098-3