Abstract
Chromosomal translocation t(11;14)(q13;q32) is a characteristic molecular marker of mantle cell lymphoma (MCL) and leads to the fusion of the immunoglobulin heavy chain enhancer-promoter with the cyclin D1 gene. Both aberrant cyclin D1 expression and underlying chromosomal aberration may be used as molecular targets for monitoring minimal residual disease (MRD). The present study aims to assess the usefulness of quantitative cyclin D1 gene expression compared to the standardised but more technologically demanding DNA-based method for immunoglobulin heavy chain (IGH) or t(11;14) clone-specific gene rearrangement quantification in a cohort of bone marrow (BM) and peripheral blood (PB) samples from patients with MCL. We simultaneously evaluated DNA-MRD and cyclin D1 expression levels in 234 samples from 57 patients. We observed that both in DNA-MRD positive and negative BM/PB pairs from the same time points the expression levels of cyclin D1 are lower in PB than in BM (median 19×, BM/PB range 0.41–352). The correlation of cyclin D1 transcript levels with DNA-MRD or with flow cytometry was good only in samples with a very high infiltration. In DNA-MRD-negative BM samples, we observed a significant heterogeneity of cyclin D1 expression (in the range of more than three orders of magnitude). This is in contrast to previous reports demonstrating the usefulness of cyclin D1 for MRD monitoring that did not use DNA-based method as a reference. In PB, the specificity of cyclin D1 expression was better due to a lower physiological background. In conclusion, we show that cyclin D1 is unsuitable for MRD monitoring in BM.
Similar content being viewed by others
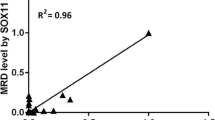
Avoid common mistakes on your manuscript.
Introduction
Mantle cell lymphoma (MCL) is defined as an aggressive type of B cell non-Hodgkin’s lymphoma (B-NHL) and represents 6–9% of lymphomas in Western Europe [1]. MCL is more often diagnosed in males than in females, and the average age at diagnosis is around the mid-60s. MCL is characterised by a rapid clinical progression and a poor long-term prognosis. The median of overall survival is 3–5 years after standard treatment [2]. The pathogenetic hallmark of MCL is chromosomal translocation t(11;14)(q13;q32) leading to overexpression of the cyclin D1 protein that is encoded by the CCND1 gene. The cyclin D1 protein is a crucial cell-cycle regulator of the G1-S transition, and cyclin D1 overexpression is directly related to tumour cell proliferation and the pathogenesis of MCL in general [3].
Molecular monitoring of minimal residual disease (MRD) using real-time quantitative polymerase chain reaction (RQ-PCR) is an effective tool for detecting very low number of malignant cells in many haematologic disorders, including MCL. Quantitative MRD detection using tumour-specific DNA targets is based on RQ-PCR analysis of the immunoglobulin (Ig) gene rearrangement or breakpoint fusion region of chromosomal translocations [4]. Clone-specific immunoglobulin heavy chain (IGH) gene rearrangements and translocation t(11;14) with the fusion gene BCL1-IGH are two major molecular markers for the detection and follow-up of MRD in patients with MCL [5, 6]. This methodology is based on DNA and has been thoroughly standardised within the EuroMRD consortium. The only limitation of this method is the time-consuming optimisation of each patient-specific MRD assay.
Previous studies have demonstrated the usefulness of detecting cyclin D1 mRNA as a tumour-specific RNA target in MCL [7,8,9]. The present study compares the results obtained by monitoring MRD using the standardised DNA-based method that detects IGH gene rearrangements or t(11;14) translocations to results obtained by monitoring the expression levels of cyclin D1 in patients with MCL. We used reverse transcription and RQ-PCR with absolute quantification to detect cyclin D1 mRNA levels in BM and PB samples and compared the results with MRD levels determined using IGH gene rearrangements or t(11;14) translocations in the largest cohort of samples yet evaluated.
Material and methods
Patients and samples
We assessed MRD in a group of patients with MCL using real-time PCR analyses of IGH gene rearrangements or t(11;14) translocations [10]. The patients received standardised treatment at four medical institutions (Supplementary Table 1). Towards this end, we analysed cyclin D1 expression levels in a total of 234 samples (174 BM; 60 PB) from 57 patients in whom the DNA-MRD results were available. The samples were taken during the initial treatment (after 3 cycles, after 6 cycles) or during the maintenance rituximab treatment (Supplementary Table 1). For the control group, we investigated cyclin D1 expression levels in 34 samples (10 BM; 24 PB) of anaplastic large cell lymphoma (ALCL) patients who were in complete remission after bone marrow transplantations (BMT). Informed consent was obtained from all patients. The study was performed after receiving approval from the institutional ethical committee and in accordance with the Helsinki declaration.
Cyclin D1 quantification
Mononuclear cells from BM/PB samples were isolated by Ficoll-Paque (density 1077 g/ml; GE Healthcare Bio-Sciences, Uppsala, Sweden) density centrifugation and stored as pellets at − 80 °C before RNA or DNA extraction. Total RNA was isolated using TRIzol Reagent (Invitrogen, Carlsbad, CA, USA) according to the manufacturer’s instructions. Complementary DNA was prepared from 10 μL of total RNA that was incubated for 5 min at 70 °C to release the possible secondary structure. The reverse transcription reaction was prepared according to conditions described previously [11].
Real-time quantitative detection assays were performed using an iQ5™ real-time PCR detection system (Bio-Rad, Hercules, CA, USA) according to the manufacturer’s instructions and utilising TaqMan real-time PCR technology. Quantification of cyclin D1 and β2-microglobulin (β2M) used a set of primers and specific probes reported previously [11, 12]. β2M was used as an endogenous reference housekeeping gene to normalise the effects of variability on RNA quality and quantity and variability in reverse transcription efficiency in the samples. The reaction mixture contained 2× concentrated TaqMan Universal PCR Master Mix II (Thermo-Fisher Scientific, Waltham, MA, USA), 0.5 μmol/L of each primer, 0.2 μmol/L of the specific probe, 5 μg bovine serum albumin, 1 μL of cDNA template per reaction, and ddH2O to a final volume of 25 μL. The reactions were performed in 96-well PCR plates (Bio-Rad, Hercules, CA, USA). The samples were amplified by using precycling hold at 50 °C for 2 min and at 95 °C for 10 min, followed by 50 cycles at 95 °C for 20 s and at 60 °C for 1 min. The samples were amplified separately from runs utilising the same cDNA, and every measurement was performed in duplicate. The copy numbers of cyclin D1 and β2M in each sample were calculated using a standard curve generated from serially diluted plasmids containing cyclin D1 or β2M cDNA, and the values from duplicates were averaged. The sensitivity of RQ-PCR was assessed in terms of the system’s ability to detect 30 copies of cyclin D1 mRNA. The concentrations of cyclin D1 and β2M transcripts were measured in each sample, and the expression level of cyclin D1 was normalised as the ratio of cyclin D1 and β2M levels.
DNA-based MRD assessment (“DNA-MRD”)
Genomic DNA was isolated using the QIAamp DNA Blood Mini from PB or BM or by QIAamp DNA FFPE Tissue Kit (Qiagen GmbH, Hilden, Germany). The clonality of the IGH rearrangements and translocation t(11;14)(q13;q32) BCL1/JH breakpoints in the BCL1-MTC region were tested using multiplex PCR, as described by the BIOMED2 collaborative study group [13]. The clonality of the PCR products was assessed using an Agilent 2100 Bioanalyzer (Agilent Technologies, Santa Clara, CA, USA). Sequencing of the clonal products was performed with a BigDye® Terminator v3.1 Cycle Sequencing Kit (Life Technologies, Carlsbad, California).
Analyses of BCL1-IGH junctional regions, the design of patient-specific primers, and the construction of dilution series in polyclonal DNA were performed as described previously [14, 15]. The dilution series and assays for MRD were prepared from BM or PB in patients with high infiltration or from lymph nodes or lymph node biopsy samples in patients with low BM/PB infiltration (Supplementary Table 1). The dilution curves were adjusted to lymphoma infiltration based on flow cytometry (FC) or on the histological staining with cyclin D1 antibody in qPCR assays established from lymph nodes, as described in our previous study (Kalinova, BJH 2015). In case of simultaneous detection of both IGH and BCL1/IGH target, the assay with a higher sensitivity and quantitative range was chosen for DNA-MRD.
Quantitative PCR was performed using patient-specific primers and germline FAM-labelled probes and reverse primers [16]. The results were interpreted according to the EuroMRD guidelines for MRD evaluation in ALL [10].
Flow cytometry
The proportion of MCL cells in the BM or PB diagnostic samples was assessed via an eight-colour flow cytometry using the following antibodies: CD4 Pacific Blue, CD20 Pacific Blue, CD45 Pacific Orange, CD8 FITC, SmIgl FITC, CD56 PE, SmIgk PE, CD5 PerCPCy5.5, CD19 PE-Cy7, TCRgd PE-Cy7, CD3 APC, CD38 APC H7, and a lymphocyte screening tube as published previously [17]. The MCL infiltration was expressed as the percentage of MCL cells among the total number of gated mononuclear cells.
Results
Cyclin D1 expression levels in BM/PB samples
We correlated the expression levels of cyclin D1 in 46 paired samples of BM and PB that were collected at the same time point of therapy and revealed a strong correlation (R 2 = 0.79) between expression levels of cyclin D1 in BM and PB samples. However, the levels could be influenced by lymphoma infiltration. Figure 1 shows the cyclin D1 expression in 46 paired BM/PB samples with available DNA-MRD (which was regarded as a reference) and in 5 paired BM/PB samples from patients with ALCL in complete remission (as non-MCL control). The results show that both in samples with positive and negative MRD, the cyclin D1 expression levels were on average 46× lower in PB compared to BM samples (BM/PB ratio: range 0.41–352, median 19). The expression levels of cyclin D1 were broadly overlapping between the DNA-MRD-positive vs. negative groups.
Correlation between cyclin D1 expression (logarithmic scale) in bone marrow (BM) and peripheral blood (PB) paired samples from patients with mantle cell lymphoma (MCL) and from patients with anaplastic large cell lymphoma (ALCL) who were in complete remission after bone marrow transplantations. DNA-MRD positive samples: any positivity in BM or PB
We analysed cyclin D1 expression in BM and PB samples of ALCL patients in complete remission after BMT and tried to establish a reliable cut-off value to distinguish between physiological and abnormal expression levels of cyclin D1 based on non-MCL control group. We detected the expression levels of cyclin D1 in BM samples at a median level of 3.1 × 10−4 (range 3.83 × 10−4–1.28 × 10−5) and in PB samples at a median level of 2.2 × 10−5 (range 3.54 × 10−4–3.53 × 10−6). Based on these findings, we established a provisional cut-off (5 × 10–4) for detection of aberrant cyclin D1 expression.
Correlation between cyclin D1 expression and IGH/t(11;14)-based MRD
To compare data obtained by cyclin D1 expression with the reference DNA-based method, we analysed 234 samples (174 BM; 60 PB). At first, we assessed the levels of cyclin D1 expression in diagnostic (Dx) samples. In a DNA-based approach, the diagnostic BM or PB is used for dilution curve preparation after adjusting to the levels of lymphoma infiltration and are arbitrarily set as 1. We therefore analysed the cyclin D1 expression in either BM or PB at Dx against the lymphoma infiltration assessed by flow (Supplementary Fig. 1). The normalised values of cyclin D1 expression seem to roughly correlate with the lymphoma infiltration assessed by flow in most samples, but there are two exceptions: first, a patient with no infiltration in BM but with a very high cyclin D1 expression and, second, a patient with blastoid variant, very high infiltration in BM, but low cyclin D1 expression. However, the numbers are limited to draw any conclusions.
Figure 2a displays a correlation of DNA-MRD with cyclin D1 expression in 158 follow-up BM samples and shows a large degree of heterogeneity in cyclin D1 expression at lower levels of IGH/t(11;14) MRD. Correlation between both methods was only good in samples for which MRD levels in cyclin D1 were higher than 6 × 10−3; however, even the samples with higher levels contained three IGH/t(11;14) MRD-negative samples. In samples with lower levels, there were a large proportion of IGH/t(11;14) negative/cyclin D1-positive samples, indicating poor specificity of the cyclin D1 measurements. In IGH/t(11;14) MRD-negative MCL patients, we detected cyclin D1 in BM samples at a median level of 7.1 × 10−4 (range 2.4 × 10−2–1.2 × 10−5). Using the cut-off of 5 × 10–4 for cyclin D1 negativity, 57 of 158 samples (36%) were false-positive compared to standard DNA-based method. Median cyclin D1 expression in those samples was 1.1 × E-03.
Figure 2b shows the same comparison for 56 PB samples. In DNA-MRD-negative PB samples, the median level of cyclin D1 expression was 3.1 × 10−5 (range 4.4 × 10−4–1.7 × 10−6). Using the cut-off of 5 × 10–4 for cyclin D1 expression, there are no cyclin D1 false-positive samples. Thus, the detection of cyclin D1 in PB seems to be more specific than in BM.
Comparison of IGH/t(11;14)-based MRD and expression levels of cyclin D1 detected during individual monitoring of the disease dynamics
We report three model cases for monitoring MRD by cyclin D1 expression in the follow-up samples obtained during therapy of patients with MCL. The cyclin D1 levels below the threshold of 5 × 10–4 were defined as negative but are depicted numerically in Fig. 3.
a–c Levels of IGH/t(11;14)-based MRD and cyclin D1 expression during the courses of treatment in three selected patients. The vertical axis shows levels of specific molecular targets. The horizontal axis shows the time between the follow-up and the initial diagnosis in days. Cyclin D1 expression levels below the suggested threshold (5 × 10–4) were defined as negative, but are depicted numerically. CR, complete remission; IGH, immunoglobulin heavy chain
In the first patient (Fig. 3a), a high MCL infiltration in the diagnostic BM was detected by flow cytometry. The cyclin D1 expression level was high at the time of diagnosis in BM and PB. After 3 cycles of therapy using R-CHOP, MRD levels decreased in both IGH and cyclin D1. The patient responded well to the treatment and, after 6 cycles of R-CHOP, reached complete remission, corresponding with MRD negativity in IGH. Afterwards, the patient passed through 8 cycles of rituximab maintenance therapy, and since the final restaging, the MRD levels have always been negative in BM and PB. Cyclin D1 expression levels were below the suggested sensitivity threshold and thus were regarded negative after 3 cycles of R-CHOP therapy.
In the second patient, we detected high levels of both DNA-MRD and cyclin D1 that corresponded with massive infiltration in BM and PB during R-CHOP therapy and led to progression of the disease (Fig. 3B).
In the third patient, we observed low expression of cyclin D1 (approximately 10−3) and very low and negative IGH MRD levels in two follow-up samples taken during 6 cycles of R-CHOP therapy (Fig. 3c). After this result, we detected a massive increase of IGH MRD and more than one log increase in cyclin D1 expression, accompanied by rapid disease progression and a clinical relapse of MCL. Due to the low frequency of follow-up examinations, we were not able to predict an impending relapse.
Discussion
The present paper presents the first study to monitor and compare MRD IGH/t(11;14) and cyclin D1 expression levels in patients with MCL and the largest study so far evaluating cyclin D1 expression in MCL specimens. Molecular monitoring of MRD by RQ-PCR of clone-specific immunoreceptor gene rearrangements and/or specific chromosomal translocations with various fusion genes is an essential part of treatment protocols for some haematological malignancies. MRD assessment enables monitoring of the disease dynamics and comparison of the efficiencies of new treatment modalities and facilitates evaluation of individual patients’ responses to therapy. MRD monitoring is also pertinent to autologous stem cell transplantation (ASCT) [6, 18, 19]. The present study endeavours to reveal the correlation between MRD IGH/t(11;14) and levels of cyclin D1 expression in patients with MCL. Optimisation of the patient-specific RQ-PCR system to enable standardised MRD monitoring using clonal rearrangement of the immunoreceptor genes and/or chromosomal translocations is labourious, expensive and time-consuming. Therefore, cyclin D1 expression could complement MRD monitoring methods in patients with MCL. A previous study in this laboratory as well as other published reports [7,8,9] have shown that monitoring cyclin D1 expression may be a useful molecular tool for assessing MRD [7]. However, at the times of publication, the monitoring of IGH/t(11;14) at DNA level had not been sufficiently standardised and, thus, was not available for use as a reference. Therefore, these studies did not evaluate an adequate number of MRD low-positive samples but rather examined mostly diagnostic or relapse specimens. The current study explores the role of cyclin D1 expression in a specific cohort of patients who were treated according to current treatment protocols. The main goal was not to compare the patients’ clinical outcomes as previous studies but to enable comparison with the standardised method based on DNA.
We evaluated cyclin D1 expression levels and endeavoured to determine a cut-off for aberrant expression of cyclin D1 in BM and PB samples. We detected very low levels of cyclin D1 expression in BM (median 3 × 10−4) and PB (median 2 × 10−5) samples in a control group of patients with ALCL who were in complete remission and thus represented physiological controls. However, we observed a substantially broader range of cyclin D1 expression in samples taken during the treatment of MCL patients who were MRD-negative using the standardised IGH/t(11;14) DNA-based method. A possible explanation for this discrepancy may lie in the existence of a small residual neoplastic cell population with very high cyclin D1 expression, which would provide higher sensitivity than the DNA-based method, as suggested by the patient’s MRD kinetics depicted in Fig. 3C. However, this is unlikely because it would imply that extremely low numbers of residual MCL cells would raise the cyclin D1 expression several logs higher, as the limit of detection of DNA-PCR is 10−5 in general. Alternatively, BM regeneration after rituximab therapy may change the BM microenviroment, leading to an increase in cyclin D1 expression that has, so far, remained unrecognised in the physiological cell population. It remains unclear whether there is a biological link between the significant heterogeneity of cyclin D1 expression in BM and one or more of the effects of rituximab therapy.
In conclusion, we demonstrate that the expression of cyclin D1 in bone marrow correlates with IGH/t(11;14) only at very high levels of MRD (above 6 × 10–3). In contrast to previous reports that rely mostly on samples with high tumour loads or on differential lymphoma diagnostics [7,8,9], we cannot recommend routine cyclin D1 testing for detecting MRD in BM with sufficient specificity. Our data demonstrate the significant heterogeneity of cyclin D1 expression in the cohort of non-quantifiable and IGH/t(11;14) MRD-negative samples. In contrast, peripheral blood seems to be a suitable material for MRD monitoring via cyclin D1 testing, because the physiological background of cyclin D1 is low. PB has recently demonstrated its prognostic application in MCL [18]. Given the relative simplicity and cost-effectiveness of cyclin D1 testing, this approach may be relevant for non-invasive MRD monitoring in patients with MCL. However, the lower stability of RNA, possibility of contamination, and the lack of standardisation, together with the availability of standardised DNA-based approach and emerging new MRD technologies as multicolour flow cytometry or next-generation sequencing, are strong arguments against using cyclin D1 expression for MRD monitoring.
References
Dreyling M, Geisler C, Hermine O, Kluin-Nelemans HC, Le Gouill S, Rule S, Shpilberg O, Walewski J, Ladetto M, Group EGW (2014) Newly diagnosed and relapsed mantle cell lymphoma: ESMO Clinical Practice Guidelines for diagnosis, treatment and follow-up. Ann Oncol 25(Suppl 3):iii83–iii92. https://doi.org/10.1093/annonc/mdu264
Herrmann A, Hoster E, Zwingers T, Brittinger G, Engelhard M, Meusers P, Reiser M, Forstpointner R, Metzner B, Peter N, Wormann B, Trumper L, Pfreundschuh M, Einsele H, Hiddemann W, Unterhalt M, Dreyling M (2009) Improvement of overall survival in advanced stage mantle cell lymphoma. J Clin Oncol 27(4):511–518. https://doi.org/10.1200/JCO.2008.16.8435
Jares P, Campo E (2008) Advances in the understanding of mantle cell lymphoma. Br J Haematol 142(2):149–165. https://doi.org/10.1111/j.1365-2141.2008.07124.x
van der Velden VH, Hochhaus A, Cazzaniga G, Szczepanski T, Gabert J, van Dongen JJ (2003) Detection of minimal residual disease in hematologic malignancies by real-time quantitative PCR: principles, approaches, and laboratory aspects. Leukemia 17(6):1013–1034. https://doi.org/10.1038/sj.leu.2402922
Cortelazzo S, Ponzoni M, Ferreri AJ, Dreyling M (2012) Mantle cell lymphoma. Crit Rev Oncol Hematol 82(1):78–101. https://doi.org/10.1016/j.critrevonc.2011.05.001
Pott C, Schrader C, Gesk S, Harder L, Tiemann M, Raff T, Bruggemann M, Ritgen M, Gahn B, Unterhalt M, Dreyling M, Hiddemann W, Siebert R, Dreger P, Kneba M (2006) Quantitative assessment of molecular remission after high-dose therapy with autologous stem cell transplantation predicts long-term remission in mantle cell lymphoma. Blood 107(6):2271–2278. https://doi.org/10.1182/blood-2005-07-2845
Brizova H, Kalinova M, Krskova L, Mrhalova M, Kodet R (2008) Quantitative monitoring of cyclin D1 expression: a molecular marker for minimal residual disease monitoring and a predictor of the disease outcome in patients with mantle cell lymphoma. Int J Cancer 123(12):2865–2870. https://doi.org/10.1002/ijc.23883
Howe JG, Crouch J, Cooper D, Smith BR (2004) Real-time quantitative reverse transcription-PCR for cyclin D1 mRNA in blood, marrow, and tissue specimens for diagnosis of mantle cell lymphoma. Clin Chem 50(1):80–87. https://doi.org/10.1373/clinchem.2003.024695
Medeiros LJ, Hai S, Thomazy VA, Estalilla OC, Romaguera J, Luthra R (2002) Real-time RT-PCR assay for quantifying cyclin D1 mRNA in B-cell non-Hodgkin's lymphomas. Mod Pathol 15(5):556–564. https://doi.org/10.1038/modpathol.3880562
van der Velden VH, Cazzaniga G, Schrauder A, Hancock J, Bader P, Panzer-Grumayer ER, Flohr T, Sutton R, Cave H, Madsen HO, Cayuela JM, Trka J, Eckert C, Foroni L, Zur Stadt U, Beldjord K, Raff T, van der Schoot CE, van Dongen JJ, European Study Group on MRDdiALL (2007) Analysis of minimal residual disease by Ig/TCR gene rearrangements: guidelines for interpretation of real-time quantitative PCR data. Leukemia 21(4):604–611. https://doi.org/10.1038/sj.leu.2404586
Brizova H, Kalinova M, Krskova L, Mrhalova M, Kodet R (2008) Quantitative measurement of cyclin D1 mRNA, a potent diagnostic tool to separate mantle cell lymphoma from other B-cell lymphoproliferative disorders. Diagn Mol Pathol: Am J Surg Pathol, Part B 17(1):39–50. https://doi.org/10.1097/PDM.0b013e318146959a
Bijwaard KE, Aguilera NS, Monczak Y, Trudel M, Taubenberger JK, Lichy JH (2001) Quantitative real-time reverse transcription-PCR assay for cyclin D1 expression: utility in the diagnosis of mantle cell lymphoma. Clin Chem 47(2):195–201
van Dongen JJ, Langerak AW, Bruggemann M, Evans PA, Hummel M, Lavender FL, Delabesse E, Davi F, Schuuring E, Garcia-Sanz R, van Krieken JH, Droese J, Gonzalez D, Bastard C, White HE, Spaargaren M, Gonzalez M, Parreira A, Smith JL, Morgan GJ, Kneba M, Macintyre EA (2003) Design and standardization of PCR primers and protocols for detection of clonal immunoglobulin and T-cell receptor gene recombinations in suspect lymphoproliferations: report of the BIOMED-2 Concerted Action BMH4-CT98-3936. Leukemia 17(12):2257–2317. https://doi.org/10.1038/sj.leu.2403202
Pott C, Bruggemann M, Ritgen M, van der Velden VH, van Dongen JJ, Kneba M (2013) MRD detection in B-cell non-Hodgkin lymphomas using Ig gene rearrangements and chromosomal translocations as targets for real-time quantitative PCR. Methods Mol Biol (Clifton, NJ) 971:175–200. https://doi.org/10.1007/978-1-62703-269-8_10
Kalinova M, Fronkova E, Klener P, Forsterova K, Lokvenc M, Mejstrikova E, Belada D, Mocikova H, Trneny M, Kodet R, Trka J (2015) The use of formalin-fixed, paraffin-embedded lymph node samples for the detection of minimal residual disease in mantle cell lymphoma. Br J Haematol 169(1):145–148. https://doi.org/10.1111/bjh.13182
Verhagen OJ, Willemse MJ, Breunis WB, Wijkhuijs AJ, Jacobs DC, Joosten SA, van Wering ER, van Dongen JJ, van der Schoot CE (2000) Application of germline IGH probes in real-time quantitative PCR for the detection of minimal residual disease in acute lymphoblastic leukemia. Leukemia 14(8):1426–1435. https://doi.org/10.1038/sj.leu.2401801
van Dongen JJ, Lhermitte L, Böttcher S, Almeida J, van der Velden VH, Flores-Montero J, Rawstron A, Asnafi V, Lécrevisse Q, Lucio P, Mejstrikova E, Szczepański T, Kalina T, de Tute R, Brüggemann M, Sedek L, Cullen M, Langerak AW, Mendonça A, Macintyre E, Martin-Ayuso M, Hrusak O, Vidriales MB, Orfao A (2012) EuroFlow antibody panels for standardized n-dimensional flow cytometric immunophenotyping of normal, reactive and malignant leukocytes. Leukemia 26(8):1821–1828
Liu H, Johnson JL, Koval G, Malnassy G, Sher D, Damon LE, Hsi ED, Bucci DM, Linker CA, Cheson BD, Stock W (2012) Detection of minimal residual disease following induction immunochemotherapy predicts progression free survival in mantle cell lymphoma: final results of CALGB 59909. Haematologica 97(4):579–585. https://doi.org/10.3324/haematol.2011.050203
Metzner B, Muller TH, Gebauer W, Casper J, Kraemer D, Rosien B, Schumann-Binarsch S, Thole R, Kohne CH, Dreyling M, Hoster E, Pott C (2014) Long-term clinical and molecular remissions in patients with mantle cell lymphoma following high-dose therapy and autologous stem cell transplantation. Ann Hematol 93(5):803–810. https://doi.org/10.1007/s00277-013-1976-x
Acknowledgements
ML, MK, and RK were supported by the Grant Agency of Charles University, GAUK no. 20214; the Ministry of Health of the Czech Republic; the project for conceptual development of research organisation 00064203 (University Hospital Motol, Prague, Czech Republic), and OPPK CZ.2.16/3.1.00/24022. EF, PK, and MT were supported by AZV 17-28980A. CLIP facilities were supported by the Ministry of Education, Youth and Sports NPU I nr.LO1604, by GBP302/12/G101 and by EU-Prague project CZ.2.16/3.1.00/24505. KF, PK, and MT were supported by the Czech Ministry of Education, Youth and Sports Institutional Support for Longterm Development of Research Organizations PROGRES Q26/LF1 and PROGRES Q28/LF1.
Author information
Authors and Affiliations
Corresponding author
Ethics declarations
Conflict of interest
The authors declare that they have no conflicts of interest.
Ethical approval
All procedures involving human participants were performed in accordance with the ethical standards of the institutional and/or national research committee(s) and in compliance with the 1964 Helsinki declaration and its later amendments or comparable ethical standards.
Informed consent
Informed consent was obtained from each participant in this study.
Additional information
Key message
• Lack of clinical utility of cyclin D1 expression in bone marrow samples for MRD monitoring
• Better specificity of cyclin D1 expression in peripheral blood
Electronic supplementary material
ESM 1
(DOCX 153 kb)
Rights and permissions
About this article
Cite this article
Lokvenc, M., Kalinova, M., Forsterova, K. et al. Cyclin D1 mRNA as a molecular marker for minimal residual disease monitoring in patients with mantle cell lymphoma. Ann Hematol 97, 467–474 (2018). https://doi.org/10.1007/s00277-017-3210-8
Received:
Accepted:
Published:
Issue Date:
DOI: https://doi.org/10.1007/s00277-017-3210-8