Abstract
The Axios River delta and the Inner Thermaikos Gulf coastal zone have experienced a long period of human interventions during the past 100 years. A post-evaluation of long run coastal zone changes under the Drivers-Pressures-State-Impacts-Response (DPSIR) conceptual framework is presented. The DPSIR approach is then used to project out into possible futures in order to connect with policy and management options proposed for the improvement of the current conditions and the achievement of sustainable development, in the coastal zone. Socio-economic driving forces with their origins in the end of the 19th century have generated numerous pressures in the coastal environment that changed the state of the environment. In the first part of the last century, there was no coupling between change of state and policy. Due to increasing environmental awareness, a coupling became more apparent over the last thirty years. Human interventions include river route realignment, extensive drainage of the plains, irrigation network, roads and dam constructions. The consequences were positive for the economic development of the area, human health, and navigation for the port of Thessaloniki. In contrast, the manipulation and over-use of natural resources has led to a reduction of wetlands, biodiversity loss, stress on freshwater supplies, and subsidence of coastal areas, aquifer salinization, and rapid coastal erosion. Three plausible future scenarios are utilised in order to investigate the implications of this environmental change process and possible socio-economic consequences.
Similar content being viewed by others
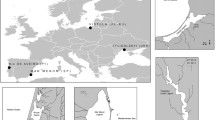
Avoid common mistakes on your manuscript.
The need for integrated environmental assessment to support the management of marine systems is now widely accepted (Cicin-Sain and others 1995; Pavasovic 1996; Antunes and Santos 1999; Talaue-McManus and others 2003). The key objective is to develop and demonstrate practical, science-based methods that support regional planning and management of the resources and environment (Condie and others 2003). Although the scientific community provides an extensive and continuous flow of information on the functioning of marine systems, this is often compartmentalised. Managers, on the other hand, require a more integrated data set with supporting interpretations in order to gain a more simple but holistic picture. In this context, the increasingly used DPSIR approach has been developed (Figure 1). DPSIR stands for Drivers-Pressures-State-Impacts-Response; the components form a broad analytical framework that links science to the causes of change and to the social, economic, and legal responses by society to that change (Turner and others 1998a,b; Elliott 2002).
The DPSIR cycle and continuous feedback processes (adapted from Turner and others 1998a).
The Axios (Vardar) River is the most important river, in terms of water and sediment supply, flowing into the northern sector of Thermaikos Gulf (NW Aegean Sea, eastern Mediterranean), hereafter referred to as Inner Thermaikos Gulf (Figure 2). The Axios river catchment covers an area of ∼25,000 km2, shared between the Former Yugoslav Republic of Macedonia (FYROM; 83%) and Greece (17%). Other rivers flowing into the area are the Aliakmon, Loudias, and Gallikos, having catchments of 10,000 km2, 1000 km2, and 1000 km2, respectively (Poulos and others 2000). Historically, the coastline was situated several kilometres to the NW (Figure 3; Stürck 1908; Poulos and others 1994; Kapsimalis and others 2005). The shallow embayment was gradually filled by riverine sediments and, in combination with tectonic uplift, the coastline retreated, creating an extensive lagoon and, later on, the Loudias Lake, which no longer exists (Figure 3). At the beginning of the 20th century, the Axios River valley was often affected by floods and the delta area was an extended swamp (Konstantinidis 1989). Since then, the Axios River delta area and its coastal zone have been heavily modified by a range of human interventions (Georgas and Perissoratis 1992; Kapsimalis and others 2005). Recently, nutrient over-enrichment and associated eutrophication problems in the Thermaikos Gulf have been assessed under the DPSIR analytical framework (Karageorgis and others 2005).
The evolution of the Thessaloniki deltaic plain since 500 BC (Konstantinidis 1989).
In this work, we use the DPSIR framework to: (1) assess the historical positive and negative socio-economic and environmental pressures and impacts; (2) identify coastal zone changes; and (3) predict future trends in the evolution of the catchment-coastal environment. The analysis includes a particular focus on the coastline configuration, as well as the eutrophication problems.
A Short Methodological Digression into DPSIR
Coasts are notoriously complex systems encompassing highly variable biotic and abiotic components. With respect to coastal management issues, such complexity entails the necessity of studying the various natural and human forcing on the coastal resources and their ability to absorb the ensuing pressures. If conducted properly, a scoping analysis should highlight long run casual chains, reveal information and modelling needs, inform about (spatial and temporal) scaling issues, indicate possible interventions and the relevant social groups involved, set the objectives and the assessment criteria for the research, and, help researchers to structure baseline and alternative scenarios (Turner 2000). In a retrospective fashion, a scoping analysis can hindcast the chain of events that led to a certain environmental problem, highlight major failures in past policy decisions and, therefore, help to assess the effectiveness of current policy proposals from a historical point of view (Meybeck 2002). The approach also allows for future scenario-based analysis in which plausible future coastal zone contexts can be formulated and examined. The aim is to reduce inevitable future uncertainties by exploring a wide range of possible environmental change sequences, their consequences, and mitigation policy options. Equally important though is the team learning process, inherent in a scoping analysis, by which natural and social science researchers learn to integrate their specific approaches and results.
Scoping analysis, as it has now been developed within large-scale, multidisciplinary research teams is based on the well-known DPSIR framework or variations of it. DPSIR was conceived (as Pressure-State-Response, see OECD 1994) as an organizing framework for indicators development but later refined (in the European Environment Agency) in order to be used as a more general, unifying platform for environmental data collection, categorization, and dissemination. The analytical substance of DPSIR has been greatly enchanced through its coupling with the notion of functional diversity (Steele 1991). “Macroscopic,” the conceptual model behind DPSIR, is based on the notions of functional diversity and integrity, which links ecosystem processes, composition, and functions with outputs of goods and services that can then be assigned monetary, economic, and/or other values (Turner 2000). The DPSIR approach is not itselt a model; on the contrary, in order to understand the dynamics between causes and effects, it is imperative to focus on the links between DPSIR nodes by applying socio-economic and natural science models in sensus stricto, i.e., the link between pressures and state could be investigated with the help of input-output models, physical run-off models, pathways, and dispersion models; the link between state and impact could be established with the help of dose-response relationships and carrying capacities considerations; and the link between impact and responses should be studied on the basis of socioeconomic models (e.g., cost-effectiveness, cost-benefit analysis, multi-criteria analysis) of risk perception and valuation of action/inaction. Finally, the effects of responses on the problem depend on which link in the causal chain the measures are mainly directed (Turner and others 1998a).
The DPSIR approach provides a cross-disciplinary conceptual map, which places the analysis in a certain sequence in order to maximize cross-disciplinary collaboration and the production of policy-relevant findings. The initial scoping stage is followed by a detailed data collection and causal chain-impacts analysis stage, which leads to the final impacts, projects, programmes, or courses of action evaluation stage. It is in this latter stage that techniques such as cost-effectiveness, cost-benefit analysis, risk-benefit analysis, and multi-criteria analysis can be deployed. The application of specific models in order to link the consequent steps in the DPSIR chain is by no means a straightforward task since: (1) what constitutes a driving force is a matter of deliberatively choosing the starting point in the causal chain (one could start from what can be labeled a “first cause” (i.e., life style, strategic policy goals) or from a “secondary cause” (i.e., a specific sectoral policy); (2) the distinction between “State” and “Impacts” is not fully clear because often “Impacts” are regarded as a further processing of the “State”; (3) “Impacts” are differently conceived by social or natural scientist, as (negative) changes to human welfare in contrast with (unfavorable) changes of the natural environment per se; and (4) data for stock and flow variables are defined, categorized, and collected in specific domains, on different spatial and/or administrative units, under specific rules and procedures, which makes their joint use problematic (see for example Marsili-Libelli and others 2004).
The DPSIR framework is not unique in its aim at visualizing the whole management cycle of a specific environmental issue. A number of other approaches attempt a scoping analysis in the coastal zone domain, such as the “outcomes” approach proposed by Olsen (Olsen and others 1997; Olsen 2003), the ecosystem domain, such as the management cycle approaches within the tradition of adaptive management (Holling 1978), the domain of atmospheric quality, such as the NAMEA (National Accounting Matrix including Environmental Accounts) approach of EUROSTAT, and in a much more restricted sense the SWOT (Strengths, Weaknesses, Opportunities, and Trends) analysis, applied mainly to economic activities and their financial and environmental externalities (Learned and others 1965; Oliver and others 2005). Each approach has its own specific merits; the fact is that a large research effort has been dedicated by OECD and EEA in the last years to the dissemination and conceptual refinement of the DPSIR approach. As a result, DPSIR has been established as the main, non-mandatory organizing framework in all major work on indicators and as a powerful scoping framework for complex environmental issues. Especially, the ongoing work on the implementation of the European Union (EU) Water Framework Directive with its consequent advancement in stakeholder consultation shows that a harmonized application of DPSIR has started influencing real policy in the EU member states while contributing to the transferability of insights within the European watershed management initiatives. (Ledoux and Burgess 2002; Vermaat and others 2005).
Hindcast Application of the DPSIR Framework in the Axios River Delta Area
Socio-Economic Drivers Evolution
At the end of the 19th century and the beginning of the 20th century, the area was occupied by the Ottoman Empire. The swamp area surrounding the Loudias Lake, and forming the deltaic plain (Figure 4), was a refuge for Greek rebels who were fighting for the liberation of the area. In addition, the Greeks had to fight against Bulgarian troops who were also claiming the land. As a result, the Turkish authorities were unable to control the area due to political instability. At the same time, malaria was a significant threat to the local population and disastrous floods during May and June, the snow-melting period, exacerbated their plight (Konstantinidis 1989). These were the origins of the socio-economic and political drivers (Figure 5) that led to the formulation of the first plans for the drainage of the Loudias Lake and the deltaic plain. The initial plans for the construction of draining channels were conducted by Kinniple and Jaffery in the period 1892–1896 and were only partly completed during 1900–1908 by the sultan Abdul Hamit. In the 1910s, the river was naturally diverted to the east, flowing into Thessaloniki Bay; the new river mouth was located 6.5 km west of Thessaloniki port (Figure 4; Albanakis and others 1993). After the liberation of Thessaloniki in 1912 and during 1916–1918, several battles took place in the Axios River area between the English-French army and Bulgarian forces. The newspapers at this time documented heavy losses in human lives due to malaria and typhus. The catastrophe of the Greeks in Asia Minor brought a wave of 800,000 refugees into the wider area of Macedonia, which generated an immediate need for economic and infrastructure support (Konstantinidis 1989). Growth of the economy remains the most powerful driving force (Figure 5). Superimposed on the socio-economic drivers are the “natural processes,” which control the geomorphologic evolution of the area, and are characterized by the river’s delta progradation, due to the accumulation of sediments.
The deltaic plain and coastline configuration in 1920 (Albanakis and others 1993).
Diachronic Pressures and Drivers
The Axios delta has been subject to a sequence of drivers and pressures over the past 100 years (Figures 5, 6). In the 1930s, the Greek state had to face the growing demands for new agricultural areas, flood control, improvement in public health, increased standard of living, reduction of unemployment, and transport improvements (Papastathis 1996). In addition, the Axios River mouth area, close to the port of Thessaloniki, progradated (siltation) rapidly and threatened to block the entrance of the port (Figure 7; Albanakis and others 1993; Kapsimalis and others 2005). Maintenance of navigation, therefore, became an additional driver and environmental pressure source (see also Figure 6).
Evolution of the deltaic plain and coastline configuration during different stages of the 20th century (Albanakis and others 1993).
To confront this situation, the Greek state assigned the task of the construction of a drainage network to the American “Foundation Company,” which operated from 1927 to 1936 (Konstantinidis 1989). In the period 1937–1952, the maintenance and improvement of the works conducted by the state’s authorities continued and are still active. The most important intervention (1930–1934) was the realignment of the river route to its present position; this work resolved the siltation problem of the Thessaloniki port entrance. At the same time (1930–1934), the Loudias Lake and the swamps were drained. Since these works, the river sediments have accumulated rapidly and formed a bird-foot type delta (Albanakis and others 1993; Poulos and others 1994; Kapsimalis and others 2005). During 1958, two irrigation dams were constructed: (1) in the Aliakmon River, near Veroia; and (2) in the Axios River, at Prochoma, about 40 km north of the river mouth. Three hydroelectric dams were constructed along the Aliakmon River and eleven more dams were constructed in the Former Yugoslav Republic of Macedonia (FYROM) part of the Axios River for irrigation purposes (Kapsimalis and others 2005); in the latter country, the irrigation systems consist of 17 high dams with a total reservoir capacity exceeding 500 million m3 of water (Konstantinidis 1989). There are plans to increase the capacity of the irrigation system and two new dams (Lisiche and Kozjak) are under construction (National Environmental Action Plan 1996). The extensive dam construction during the 20th century, therefore, constitutes an environmental pressure of major importance (Poulos and Collins 2002; Syvitski and others 2005), which was increased by over-consumption of water supplies for irrigation and the emerging increased demand for drinking water. Economic growth also resulted in the demand for construction materials, mainly for the development of an extended transportation network (railway, roads and bridges). Finally, at the end of the 20th century a new pressure for the coastal zone has emerged through the rapid evolution of aquaculture activities, which generally increase the emissions of nitrogen and phosphorous (Islam 2005).
Environmental State of the Coastal Zone
Due to the sequence of drivers and pressures, the state of the coastal zone has changed dramatically over the past 100 years. According to Kapsimalis and others (2005), the evolution of the coastal zone can be separated into three successive stages (Table 1). During Stage I (∼2000 BC to 1920 AD), the coastal zone is characterized by accretion trends, dominated by the continuous undisturbed riverine sediment discharge. At this stage, the Axios River was filling the lowlands with sediment. In Stage II, between 1920 and 1952, the sediment discharge of the river increased substantially. This resulted in the artificial deviation of its course to its present location. In addition, meanders and wetlands were destroyed, agricultural land was increased, through the draining of the Loudias Lake and the swamps, and finally, the new river bed was artificially aligned (Figure 7). Thus, sedimentation rates increased, resulting in fast accretion of the coastline. The third Stage (1952 to 2000) represents the period of intense human interventions. Several dams, for irrigation and hydroelectric power, were constructed. The demand for irrigation water is still an increasing pressure, as land reclamation projects have provided vast areas for agriculture. For example, the drainage of Loudias Lake produced an extra of 217,000 ha for cultivation (Konstantinidis 1989), while the total amount of flood-protected or drained land reached 1,000,000 ha (Papastathis 1996).
Impacts on the Coastal Zone
The most apparent impact induced by human interventions is the actual disappearance of the Loudias Lake and the swamps, mainly due to drainage works. The only remaining wetlands are located in the lower delta area of the Axios River. However, a significant loss of wetlands and biodiversity occurred during the 20th century (Figure 8). Konstantinidis (1989) describes a wealth of wildlife in the Loudias Lake and the associated reeds; during 1930, freshwater fish production reached 650 tonnes. However, during the past ten years in particular, the flora and fauna biodiversity has declined significantly and some rare bird species are almost extinct (Albanis and others 1996; Goutner and others 2001). However, Evmorphopoulos (1961) has suggested that the lake would probably have disappeared anyway due to continuous infill by river sediments.
The demand for irrigation water increased substantially during the second half of the 20th century; thus, freshwater was stored in reservoirs or pumped directly from the aquifer to support the cultivation of mainly wheat, maize, rice, tobacco, and barley. During this period, the Axios River water discharge fell by 40%, from 5 billions m3 yr−1 to 3 billions m3 yr−1, while the sediment discharge suffered a 20-fold decrease (Karageorgis and Anagnostou 2001; Karageorgis and others 2005). Freshwater quality loss is particularly evident during the dry summer months, where the Axios River discharge is often less than 10 m3 s−1, and the river bank is completely dry. Another indirect impact is related to the freshwater supply to the Thermaikos Gulf. Freshwater enhances the cyclonic circulation of the water masses that help with the dilution/mixing of the Inner Thermaikos waters (Kontoyiannis and others 2003), which are enriched in various domestic and industrial effluents (Kontoyiannis and Karamanos 2000). This equilibrium is often disturbed during the summer months, when stagnation is observed in the Inner Gulf with negative consequences for the aquaculture (mainly mussel farming) activities and the occurrences of harmful algal blooms (Moncheva and others 2001). We return to this eutrophication problem in a later section dealing with future scenarios. Freshwater deficiency that is also related to overpumping of the aquifers has also induced sedimentary deposits compaction; thus subsidence occurred since 1960, particularly in the eastern part of the delta and near the city of Thessaloniki (Stiros 2001). Similarly, salinization of the aquifers and the soils is observed throughout the Axios River valley, with an increasing trend from the north to the south.
On the other hand, the substantial reduction in river sediment resulted in the deterioration of coastal deposits and the stimulation of rapid erosion processes (Kapsimalis and others 2005), which occurred for the first time in the history of the delta (Figure 8). On top of this, additional problems arise from the (illegal) sand extraction from the river beds for use as a construction material. Breakwaters and seawalls were constructed (length of ∼22 km) to protect the coast from erosion and these works are still in progress. The erosion also impacted upon the underwater topography; since 1956, the inner Thermaikos Gulf has entered an erosional period featuring overall sediment losses of about 110,000,000 m3 (Kapsimalis and others 2005). Unfortunately, long-term zoo- and phyto-benthos studies are not available to assess the consequences of these changes to benthic communities.
Policy Responses
State policy and management responses have been implemented in a piecemeal fashion in relation to changing drivers and pressures. This phenomenon was particularly evident in the first half of the 20th century, when environmental conservation was not a primary concern. It should be noted that during this period the legislative background permitted extensive interventions in the environment. Summarizing, the “immediate” responses were: (1) Axios River diversions; (2) drainage of the lakes and swamps; (3) construction of irrigation networks; (4) construction of roads and bridges; and (5) dams construction. While this strategy has led to economic development gains, it is questionable whether these socio-economic net benefits are sustainable over the long run.
Policy responses in the second half of the 20th century can be characterized by a more strategic and controlled approach, one in which environmental concerns were also considered. These are, in chronological order: (1) development of a “master plan” for the Thessaloniki plain and the Thermaikos Gulf catchments (1953); (2) 6,700 ha of the lower Axios River delta were designated as a protected area under the Ramsar convention (1974); (3) 22,643 ha of the Aliakmon-Loudias-Axios River deltas and other coastal areas were designated as parts of the EU network of protected areas Natura 2000 (1993); (4) development of the Axios/Vardar River master plan between Greece and FYROM (1994); and (5) 13,763 ha of the delta were designated as Special Protected Areas under the European Union Wild Birds Directive 79/409/EEC (1998). In addition, considerable effort was put into the improvement of wastewater treatment plants for the domestic and industrial effluents of the city of Thessaloniki, which contributed to the control of nutrient emissions into the marine environment.
Future Environmental Change
Long-term analysis has shown that more than 11,800 t of nitrogen and 3,400 t of phosphorous are currently being released into the marine system (Karageorgis and others 2005). Over the last 20 years, while domestic and industrial effluent loads have started to decrease, riverine nutrient loadings have increased and freshwater discharge have fallen. Eutrophic events are still, therefore, a significant problem. The damage costs borne by society include the negative impact of eutrophicated waters on aquaculture, e.g., mussel farm productivity losses, and an environmental amenity loss focused around recreation and local environmental civic pride (Kontogianni and others, 2001).
Three future scenarios—“business as usual, BAU,” “policy targets, PT,” and “deep green, DG”—were formulated in order to assess a range of plausible outcomes (Salomons 2004). The scenarios were used to generate an array of inputs for the catchment-coastal zone modelling and the impacts on different stakeholder groups in society (Karageorgis and others 2005). The shellfish farming activity in the Axios delta is financially profitable and provides around 85% of the total Greek shellfish output per annum. Eutrophic episodes adversely affect the size, weight, and growth period of mussels, as well as engendering a negative image in the minds of consumers concerned about possible health effects. The probability of toxic blooms and consequent significant economic damage costs in the mussel farming sector was assessed across the different scenarios. Under BAU, the probability of occurrence of such events remained high (0.8), falling to (0.65) under PT and to (0.20) in the DG scenario. The economic cost of algal bloom events in terms of lost sales (or delayed sales at reduced prices) was estimated to be around 3 million € per annum (on production levels of 30,000 t). The expected annual losses across the three scenarios were 2.4, 1.95, and 0.6 million €, respectively.
The coastal area east and southeast of the city of Thessaloniki has been an important tourist resort area for local people. The stakeholder analysis also revealed that other motivations beyond recreational usage were held. People felt that a cleaner Bay was part of a requirement for general improvement in the area’s environs. Citizens felt that environmental improvement would enhance local self-esteem and identity (civic pride), as well as safeguarding opportunities for future generations (Kontogianni and others 2001). A contingent valuation study of the willingness to pay of residents to clean up the water in the Thessaloniki Bay indicated that the ecological goods and services provided have significant economic value. The survey asked residents about their willingness to pay extra quarterly water rate payments for cleaning up Thessaloniki Bay. The mean willingness to pay was ∼15€ per quarter and the aggregate social benefits of clean coastal water was around 34 million€ per annum (Kontogianni and others 2001). If realised in practice, this funding would be sufficient to install and maintain adequate waste water treatment plant capacity, but is only likely in a PT or DG future state of affairs.
Synthesis and Conclusions
Societal Benefits and Impacts on Nature
Human interventions have resulted in significant benefits for society during the past 100 years in the Axios delta area. At first, drainage works provided land for cultivation, especially for the Greek refugees from Asia Minor in the early 1930s. Several hundreds of thousands of hectares have been exploited since then, changing the economic profile of the area, from poverty to a prosperous agricultural community, with a reduction in unemployment. Refugees were initially employed by the construction companies as workers; they were then offered land by the state to cultivate. Furthermore, lethal diseases, such as malaria, typhus, and tuberculosis, were eradicated. A huge irrigation network, probably the best in Greece, was developed, serving the needs of agriculture. The road network was also substantially improved. Just in the period 1927–1936, for example, 29 road and railroad bridges were constructed, together with several thousands of kilometres of subsidiary roads that were used for goods transportation. Finally, the diversion of the Axios River resolved effectively the potential problem of siltation of the Thessaloniki port.
On the other hand, several negative changes on the environment can be highlighted. The significant reduction of wetlands was accompanied by significant biodiversity loss. Freshwater resources decreased by 40%, resulting in subsidence of urban and industrial areas, aquifer and soil salinization. The most serious threat is probably coastal erosion, due to the general decrease in freshwater and sediment flows, which are now retained within the reservoirs. This effect has an impact on the biological resources of the Thermaikos Gulf as well, as river-borne silicates are used as nutrients by marine organisms.
Possible Environmental Futures Related to EU Policies
The application of the DPSIR framework was used to hindcast the changes in the Thermaikos Gulf and Axios Delta in order to better assess the future challenges that the region will inevitably face. The framework also facilitated a general picture of possible coastal futures for Europe as a whole, and specifically for our region, indicating that pressures on coastal ecosystems will continue to increase under all plausible assumptions (Vermaat and others 2005). In our case, if current regional trends persist, many negative impacts will be maintained or reinforced, with significant negative economic consequences for aquaculture and unrealised environmental amenity. Maximising economic development remains a primary goal of the state, meaning that additional pressures will be exerted on the coastal system under the BAU projection. However, state and public awareness has been rising during past decades and has generated plans and support for a more sustainable use of ecosystem resources. Accordingly, fundamental changes have occurred in our understanding of the functions and values of catchments and these have prompted many recent international efforts to protect and use them in a sustainable way. Today, these efforts are coordinated through a number of international protocols and agreements, the implementation of which requires concerted action, joint fund raising, and mutual cooperation in catchment management and policy. A relatively large number of legal documents are directly or indirectly affecting the management of land, water, and natural resources in the Axios catchment area. They are categorized into three groups: National framework (national laws, Ministerial Decisions, Prefecture Decisions, etc.), Common European framework (Directives), and international (including bilateral) agreements and conventions.
On a European scale, the recent Water Framework Directive (WFD) stands prominently in offering tools in support of an integrated management of watersheds. In this context, adopting the “wise use” imperative of the European Union is a prerequisite, as is also taking explicitly into account a number of factors considered to affect specifically the management of Mediterranean catchments: (1) developmental needs and economic inequality; (2) pressure from population growth, immigration and mass tourism; and (3) social and cultural conflicts.
The socio-economic setting defining the relevant institutions in the Axios catchment are: the political situation in the Balkans, Greek development policy for the area, and the citizen’s life-style driven by major EU/national legislative framework. The major socio-economic drivers that affect the total Axios catchment area (FYROM and Greece) can then be summarized as follows: (1) the wider political and economic destabilization of the Balkans region leading to uncertainty, inhibition of the rate of growth and lack of sufficient effort to tackle environmental degradation, as well as the influx of 261,000 refugees in FYROM; (2) the new Greek Development Strategy for the role of Thessaloniki as the new Metropolitan center of the Balkans (Balkan and Black Sea area Cooperation Pole); and (3) the European Union Policies, especially the Common Agricultural and Fisheries Policies, the Water Framework Directive, and the Habitat Directive.
Within the third Community Support Framework 2000–2006, the Central Macedonia Funding Program, which will finance the above-mentioned actions, has a total public expenditure of 1.2 billion €. FYROM is in a period of transition as it moves towards a market-based economy. Within this framework, it is apparent that FYROM is seeking to make progress in the field of environmental protection and is also very keen to comply with EU environmental requirements and standards (National Environmental Action Plan 1996). Nevertheless, the adoption of new environmental legislation and the creation of new institutional structures by FYROM need to be supported by investment, implementation, and enforcement. Specific environmental management recommendations given by UNEP (2000) for the water sector in FYROM include the following: “an integrated river basin management plan should be developed and implemented for the Vardar (Axios) River. The approach should be consistent with the EU Water Framework Directive and take full account of trans-boundary considerations.”
On the regional scale, our suggestions for the future include: (1) gradual replacement of highly water-consuming cultivations (rice) in the lower part of the Axios River delta; (2) better management (storage) of freshwater, particularly during the winter months of high river discharge; (3) control of overpumping in the deltaic plain; (4) legislation imposing ‘hard and fast” rules for the illegal sand extraction from the river bed; (5) encouragement of protected areas under the Ramsar convention and the Natura network of protected areas; (6) improvement of agro-tourism; (7) further expansion of erosion protecting constructions in the coastal zone under most threat; (8) to abandon, on cost-benefit grounds, certain areas of deltaic coast under subsidence; (9) improve wastewater treatment, and (10) galvanise public support for environmental improvements as part of a wider civic amenity enhancement policy.
The DPSIR conceptual framework could be applied easily to other catchment/coastal zone systems, which have experienced human interventions during past decades. This would help in the identification and assessment of socio-economic drivers, pressures, environmental state, impacts and policy responses, in the long term, thus providing a holistic and comprehensive approach on issues pertaining to environmental protection and the sustainable management of natural resources. Moreover, the ability to apply future scenarios enlarges the use of DPSIR to a robust and reliable management tool. Natural scientists, stakeholders, and policy makers would all benefit from such integrated analysis.
Literature Cited
Albanakis, K., Vavliakis, E., Psilovikos, A., and L. Sotiriadis. 1993. Mechanisms and evolution of the delta of Axios River during the 20th Century. Pages 311–325 in Proceedings of the 3rd Hellenic Geography Conference, Hellenic Geographical Society, Athens, Greece
Albanis T. A., Hela D., Papakostas G., V. Goutner. 1996. Concentration and bioaccumulation of organochlorine pesticide residues in herons and their prey in wetlands of Thermaikos Gulf, Macedonia, Greece. The Science of the Total Environment 182:11–19
Antunes P., R. Santos. 1999. Integrated environmental management of the oceans. Ecological Economics 31:215–226
Cicin-Sain B., Knecht R.W., G.W. Fisk. 1995. Growth in capacity for integrated coastal management since UNCED: an international perspective. Ocean & Coastal Management 29(1-3):93–123
Condie S., Fandry C., McDonald D., Parslow J., K. Sainsbury. 2003. Linking ocean models to coastal management on Australia’s North West shelf. Eos, Transactions of American Geophysical Union 84(6):49
Elliott M. 2002. The role of the DPSIR approach and conceptual models in marine environmental management: an example for offshore wind power. Marine Pollution Bulletin 44:iii–vii
Evmorphopoulos L. 1961. The changes of Thessaloniki Bay. Greek Technical Annals 205-208:51–76
Georgas D., C. Perissoratis. 1992. Implications of future climatic changes on the Inner Thermaikos Gulf. In L. Jeftic and J. Milliman(eds.), Climatic change and the Mediterranean. UNEP, Edward Arnold, London. Pages 495–534
Goutner V., Papagiannis I., V. Kalfakakou. 2001. Lead and cadmium in eggs of colonially nesting waterbirds of different position in the food chain of Greek wetlands of international importance. The Science of the Total Environment 267:169–176
Holling C.S. (ed.). 1978. Adaptive environmental assessment and management. John Wiley and Sons, Inc., New York, 377 pp
Islam M.S. 2005. Nitrogen and phosphorus budget in coastal and marine cage aquaculture and impacts of effluent loading on ecosystem: review and analysis towards model development. Marine Pollution Bulletin 50(1):48–61
Kapsimalis V., Poulos S. E., Karageorgis A. P., Pavlakis P., M. B. Collins. 2005. Recent evolution of a Mediterranean deltaic coastal zone: human impacts on the Inner Thermaikos Gulf, NW Aegean Sea. Journal of Geological Society, London 162:1–12
Karageorgis A. P., C. L. Anagnostou. 2001. Particulate matter spatial-temporal distribution and associated surface sediment properties: Thermaikos Gulf and Sporades Basin, NW Aegean Sea. Continental Shelf Research 21:2141–2153
Karageorgis A. P., Skourtos M. S., Kapsimalis V., Kontogianni A. D., Skoulikidis N.T., Pagou K., Nikolaidis N.P., Drakopoulou P., Zanou B., Karamanos H., Levkov Z., C. Anagnostou. 2005. An integrated approach to watershed management within the DPSIR framework: Axios River Catchment and Thermaikos Gulf. Regional Environmental Change 5:138–160
Konstantinidis K. A. 1989. Land reclamation project of the Thessaloniki plain. Geothechnical Chamber of Greece, Thessaloniki, Greece, 217 pp
Kontogianni, A., Langford, I. H., Papandreou, A., and Skourtos, M. S. 2001. Social preferences for improving water quality: an economic analysis of benefits of waste-water treatment, CSERGE Working Paper GEC o1-04, University of East Anglia, Norwich, UK
Kontoyiannis, H., and H. Karamanos. 2000. North Thermaikos Gulf: The role of the rivers in the inflow of Aegean water. Pages 48–53 in Proceedings of the 6th Hellenic Symposium on Oceanography & Fisheries, 23–26 May 2000. Chios, Greece
Kontoyiannis, H., Kourafalou, V. H., and V. Papadopoulos. 2003. The seasonal characteristics of the hydrology and circulation in the northwest Aegean Sea (eastern Mediterranean): Observations and modeling. Journal of Geophysical Research 108(c9), 3302, doi:10.1029/2001.JC001132
Learned E.P., Christensen C.R., Andrews K.E., W.D. Guth. 1965. Business policy: Text and cases. Irwin, Homewood, IL
Ledoux, L., and D. Burgess (eds.). 2002. Page 728 in Proceedings of the Science for Water Policy (SWAP): The implications of the Water Framework Directive, 1–4 September 2002, University of East Anglia, Norwich
Marsili-Libelli, S., Betti, F., and S. Cavalieri. 2004. Introducing river modelling in the implementation of the DPSIR scheme of the water framework directive. Pages 1–6 in C. Pahl, S. Schmidt, A.E. Rizzoli, and A. Jakeman (eds.), 3rd Intelligent Environmental Modelling and Software Society 2004 Congress: Complexity and integrated resources management, 14–17 June 2004. Osnabrück, Germany
Meybeck M. 2002. Riverine quality at the Anthropocene: Propositions for global space and time analysis, illustrated by the Seine River. Aquatic Science 64:376–393
Moncheva S., Gotsis-Skretas O., Pagou K., A. Krastev. 2001. Phytoplankton blooms in Black Sea and Mediterranean ecosystems subjected to anthropogenic eutrophication: Similarities and differences. Estuarine Coastal and Shelf Science 53:281–295
National Environmental Action Plan. 1996. Republic of Macedonia-Synthesis Report. Ministry of Urban Planning, Construction and Environment, Skopje, 78 pp
OECD. 1994. Environmental Indicators: OECD Core Set. Organization for Economic Cooperation and Development, Paris, 159 pp
Oliver M., Sorkin L., A., Kaschl. 2005. Science and policy interface: the LIFE programme and its links to the EU Water Framework Directive. Environmental Science & Policy 8:253–257
Olsen S.B. 2003. Frameworks and indicators for assessing progress in integrated coastal management initiatives. Ocean & Coastal Management 46:347–361
Olsen S.B., Tobey J., M., Ker. 1997. A common framework for learning from ICM Experience. Ocean & Coastal Management 37:155–174
Papastathis, G. 1996. Draining works in the Thessaloniki Valley. Pages 689–737 in Proceedings of the 2nd Hellenic Conference on Land reclamation works, management of water resources, automation of agriculture, 24–27 April 1996. Larissa, Greece
Pavasovic A. 1996. The Mediterranean Action Plan phase II and the revised Barcelona Convention: new prospective for integrated coastal management in the Mediterranean region. Ocean & Coastal Management 31(2–3):133–182
Poulos S., Papadopoulos A., M. B. Collins. 1994. Deltaic progradation in Thermaikos Bay, Northern Greece and its socio-economic implications. Ocean and Coastal Management 22:229–247
Poulos, S. E., and M. B. Collins. 2002. Fluviatile sediment fluxes to the Mediterranean Sea: a quantitative approach and the influence of dams. Pages 227–245 in S. J. Jones, and L. E. Frostick (eds.), Sediment flux to basins: causes, controls and consequences. Journal of Geological Society, London, Special Publications 191
Poulos S. E., Chronis G. T., Collins M. B., V. Lykousis. 2000. Thermaikos Gulf coastal system, NW Aegean Sea: an overview of water/sediment fluxes in relation to air–land–ocean interactions and human activities. Journal of Marine Systems 25:47–76
Salomons W. 2004 European Catchments: catchment changes and their impact on the coast. Institute for Enviornmental Studies, Free University, Amsterdam
Steele J. H. 1991. Marine functional diversity. Bioscience 41:470–474
Stiros S.C. 2001. Subsidence of the Thessaloniki (northern Greece) coastal plain, 1960–1999. Engineering Geology 61:243–256
Stürck J. 1908. Mazedonisch Fahrten II, Die Mazedonischen Niederlaender. University of Serjevo, Serjevo
Syvitski J. P. M., Vörösmarty C. J., Kettner A. J., P., Green. 2005. Impact of humans on the flux of terrestrial sediment to the global coastal ocean. Science 308:376–380
Talaue-McManusa L., Smith S. V., R.W. Buddemeierc. 2003. Biophysical and socio-economic assessments of the coastal zone: the LOICZ approach. Ocean & Coastal Management 46:323–333
Turner R. K. 2000. Integrating natural and socio-economic science in coastal management. Journal of Marine Systems 25:447–460
Turner, R. K., Adger, W. N., and I. Lorenzoni. 1998a. Towards integrated modelling and analysis in coastal zones: Principles and practices. LOICZ Reports & Studies, No. 11, LOICZ IPO. Texel, The Netherlands, 122 pp
Turner R. K., Lorenzoni I., Beaumont N., Bateman I. J., Langford I. H., A. L. McDonald. 1998b. Coastal management for sustainable development: analysing environmental and socio-economic changes on the UK coast. The Geographical Journal 164:269–281
UNEP. 2000. Post-conflict environmental assessment-FYR of Mace-donia. United Nations Environment Programme, Switzerland, 88 pp
Vermaat J., Bouwer L., Turner K., W. Salomons (eds.). 2005. Managing European coasts: Past, present and future. Springer Verlag, Berlin, 387 pp
Acknowledgments
This research was performed under the auspices of the project EUROCAT (European catchments. Catchment changes and their impact on the coast), funded by the European Commission, DG Research, under contract No. EVK1-CT-2000-00044. We wish to thank Michel Meybeck (Université de Paris 6) for intuitive suggestions and constructive conversations and M. Elliott (University of Hull) for helpful comments on an earlier version of the manuscript. Comments and suggestions from three anonymous reviewers are also acknowledged.
Author information
Authors and Affiliations
Corresponding author
Rights and permissions
About this article
Cite this article
Karageorgis, A.P., Kapsimalis, V., Kontogianni, A. et al. Impact of 100-Year Human Interventions on the Deltaic Coastal Zone of the Inner Thermaikos Gulf (Greece): A DPSIR Framework Analysis. Environmental Management 38, 304–315 (2006). https://doi.org/10.1007/s00267-004-0290-8
Published:
Issue Date:
DOI: https://doi.org/10.1007/s00267-004-0290-8