Abstract
We evaluated the effect of conspecific abundance and habitat quality of leks on the territorial behaviour of males in an exploded lekking species, the Little Bustard (Tetrax tetrax). The hypothesis that males more intensely defend territories with higher conspecific abundance and better habitat quality was evaluated experimentally analysing the agonistic response of experimental males to male decoys placed on their displaying areas. Decoy experiments showed that the intensity of display territory defence by little bustard males is density dependent. The time experimental males took to return to their display sites after decoy placement decreased with abundance of both males and females. The strength of their final response was positively associated to local male and female abundance in the vicinity of their display sites. Habitat quality also influenced males’ display territory defence since the intensity of male response increased with the degree of natural vegetation cover. Habitat quality was particularly relevant in explaining variation of experimental males’ snort call rate, which decreased with the degree in plough cover and increased with the number of fields in the lekking area. Snort call rate decreased with the level of male aggregation and was lowest in males exhibiting the strongest aggressive response to decoys. These results add new evidence for the density dependence of species’ breeding territorial behaviour, supporting density-dependent models of lek formation and reinforcing the role of resources defence in exploded lek mating systems.
Similar content being viewed by others
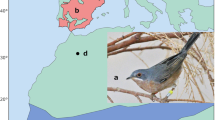
Avoid common mistakes on your manuscript.
Introduction
Increasing evidence supports the idea that density dependence affects breeding territorial behaviour (Bessa-Gomes et al. 2004; Bretagnolle et al. 2008; Fasce et al. 2011). In polygynous species, relevant components of mating system dynamics such as display rate, male attractiveness, territory occupancy and male spatial distribution can be influenced by conspecific abundance and related parameters like operational sex ratio (Ligon 1999). In these species, female density may strongly influence male behaviour and breeding strategy (Kokko and Rankin 2006), and density-dependent regulation may occur through direct behavioural interference (López-Sepulcre and Kokko 2005; Bretagnolle et al. 2008). In fact, intra-sexual male competition is particularly strong in polygynous species (Orians 1969; Emlen and Oring 1977; Wittenberger 1979). In these species, males may even fight each other to gain access to females either through the control of territory resources, the attainment of high hierarchical position or both (Clutton-Brock et al. 1982; Gosling et al. 1987; Festa-Bianchet et al. 1990; Mysterud et al. 2005; Magaña et al. 2011).
Leks are a type of polygynous mating system in which clustered males defend small territories that females visit only with the purpose of mating (Höglund and Alatalo 1995). According to Bradbury (1981), a classical lek can be defined as a non-resource-based mating system in which females select mates among males, whose contribution to their offspring fitness in terms of direct benefits is very small. Very often, males show complex morphological and/or behavioural traits specifically evolved to attract females (Andersson 1994; Höglund and Alatalo 1995). However, in the so-called exploded lekking species, male territories are only loosely aggregated (Gilliard 1969; Emlen and Oring 1977; Höglund and Alatalo 1995), and consequently males can hold large territories containing resources potentially exploitable by breeding females (Höglund and Alatalo 1995; Ligon 1999; Morales et al 2001). In these species, inter-male agonistic interactions may play a role not only in hierarchy establishment and female monopolization, but also in territory defence (Olea et al. 2010; Ponjoan et al. 2012).
In this paper, we evaluate the effect of conspecific density and habitat quality on male territorial behaviour in an exploded lekking bird, the Little Bustard (Tetrax tetrax). We use little bustard male decoys to test experimentally the hypothesis that males defend territories more intensely when conspecific densities are higher and habitat quality is better, analysing the agonistic response to decoys placed on male displaying areas. According to this hypothesis, we predicted that the intensity of display territory defence should be higher in leks (1) with greater conspecific density (and thus higher potential for inter-male agonistic interactions) and (2) of better habitat quality (i.e. containing more resources).
Material and methods
Study species
The little bustard is a medium-sized steppe bird with a typical exploded lek mating system (Morales et al. 2001) that exhibits a strong territorial behaviour during the breeding season ( Schulz 1986; Jiguet et al. 2002; Jiguet and Bretagnolle 2006). It is a sexually dimorphic species whose breeding males exhibit a conspicuous black and white neck plumage design, while females are rather discrete (Cramp and Simmons 1980). Male little bustards select breeding territories that provide food and habitat for display and shelter (Jiguet et al. 2000; Morales et al. 2008; Traba et al. 2008; Delgado et al. 2010; Ponjoan et al. 2012). Differences in male attractiveness, as well as in display and ranging behaviour, have been related to age, male phenotypic traits and habitat availability (Jiguet et al. 2000; Jiguet and Bretagnolle 2006; Ponjoan et al. 2012; Jiguet and Bretagnolle 2014). Male sexual display can be split into three categories according to Jiguet and Bretagnolle (2001): snort calls (involved in male–male interactions) and wing flashes and jumps (which are more complex displays), both mainly associated to inter-sexual signalling.
The little bustard principally inhabits natural and cereal steppes of the Western Palearctic (Del Hoyo et al. 1996). During the breeding season, males have preference for extensive and heterogeneous agricultural landscapes and occupy mainly fallow agricultural fields and natural vegetation as display sites (Martinez 1994; Wolff et al. 2002; Morales et al. 2005; Delgado et al. 2010). In fact, the presence of fallows, natural vegetation and field margins in mosaic cereal landscapes is so relevant for the species’ reproduction (see recent results by Delgado et al. 2010; Silva 2010; Lapiedra et al. 2011; Ponjoan et al. 2012; Morales et al. 2013; Tarjuelo et al. 2013) that their availability can be used as a landscape-scale habitat quality index.
Study areas
The study was carried out in four cereal steppe sites of the Iberian Peninsula—two in the Central Plateau (Campo de Calatrava (38° 50′ N, 4° 33′ W, 610 m asl, 5,374 ha) and Valdetorres del Jarama (40° 40′ N, 3° 25′ W, 700 m asl, 1,500 ha)) and two in the Ebro valley basin (Bellmunt (41° 47′ N, 0° 57′ E, 380 m asl, 1,769 ha) and Belianes (41° 35′ N, 0° 59′ E, 380 m asl, 2,583 ha)). The four study areas present little natural vegetation cover and are dominated by dry cereal crops (more than 50 % of their surface). Leguminous crops, ploughed fields, vineyards, olive or almond groves, young and long-term fallows, and pasturelands were present in the study sites, as well as other minority habitats such as fruit orchards and urban areas. Mean field size (±SD) was 1.68 ± 3.5 ha in Valdetorres del Jarama, 2.93 ± 5.83 ha in Campo de Calatrava, 1.15 ± 1.44 ha in Bellmunt and 1.62 ± 3.83 ha in Belianes. These areas are within strongholds of Iberian little bustard populations and support different population densities—Valdetorres del Jarama, 1.9 males/km2; Campo de Calatrava, 3.2 males/km2; Bellmunt, 3.5 males/km2; Belianes, 4 males/km2 (MBM, unpublished data). These density values range from low to moderate within the Iberian context (Silva et al. 2014), but they do not sensibly differ from the average value of the Spanish population (García de la Morena et al. 2006).
Data collection
Bird surveys
In each study site, we surveyed breeding little bustard males by means of car transects during the mating season peak (late April-mid May; Cramp and Simmons 1980; Morales et al. 2008) every 1–2 weeks. The aim of these transects was to know the number of occupied male territories in each study area, which provided context information about the distribution of territorial males during the experimental period (see below). Transects were covered at low speed (20 km/h) using local track networks and stopping every 500 m. All visually and acoustically detected males were recorded on 1:10,000 maps. Censuses were conducted on consecutive days to cover the entire area, from dawn to the next 3 h, and during the last 3 h before dusk, coinciding with the males’ daily activity peaks (Schulz 1986). After each census, potential double counts were eliminated by cross-checking maps made by different surveyors covering a study site, paying special attention to the few individuals that flew from one census sector to another. The high density of tracks and the breeding males’ high conspicuousness allowed detection of nearly all territorial males present in the four study sites (for further details on the survey method, see Morales et al. 2008; Delgado et al. 2010). This procedure allowed us to choose experimental males (see below) among territorial ones only, as well as to confirm that most males were also territorial (see also Delgado et al. 2010; Ponjoan et al. 2012). Male locations were plotted on a digitalized map of each study area and used to later calculate the distance (in metres) from each decoy placement point to the nearest non-experimental male (see below and Table 1). For these operations, we used the application for Geographical Information Systems Arcview 3.2.
Decoy experiments
We carried out 82 experiments placing little bustard breeding male decoys in displaying male territories (33, 11, 21 and 17 in Campo de Calatrava, Valdetorres del Jarama, Bellmunt and Belianes, respectively). Decoys were made of expanded polystyrene foam modelled in a pre-shaped plaster matrix and painted with matte paint (Jiguet and Bretagnolle 2006). We used the same decoys used by Jiguet and Bretagnolle (2006) in similar experiments demonstrating that decoys were recognized as conspecifics by experimental males. All decoys corresponded to the M1 phenotypic type (symmetric V-neck collar and black and white breast bands of equal widths), which produces the strongest behavioural response in both males and females (Jiguet and Bretagnolle 2006).
Experiments were carried out between April 26 and May 31 in 2004, a period including the peak of the male displaying period (ca. May 15 in Central Spain; Delgado et al 2010), and during the highest activity hours (from dawn to the next 3 h and during the last 3 h before dusk). The same experimental procedure was followed in the four study sites. Observations in each experiment were made from a fixed, good visibility (detectability within observation buffers could be considered close to 100 %, see below), distant point (more than 300 m far from the decoy) to avoid interference with experimental males. Experimental individuals were selected from territorial males recorded during surveys, trying to obtain a gradient in surrounding male density, from more densely neighbour territory-surrounded males to more sparsely surrounded ones. Before the onset of the experiment, each experimental male was identified individually according to plumage characteristics following Arroyo and Bretagnolle (1999). These phenotypic traits (see Fig. S1) have been consistently used in little bustard individually based studies both in French and Spanish populations (Jiguet et al. 2000, 2002; Jiguet and Ollivier 2002; Traba et al. 2008; Delgado et al. 2010; Morales et al. 2013; Jiguet and Bretagnolle 2014). Each experimental male was subjected only once to the experiment.
Before the start of each experiment, the surroundings of the experimental male were carefully scanned, and all males and females detected within a 200 m radius buffer around the experimental male display position recorded on fine-scale habitat maps (see below). Fine-scale maps were always compared with the study area territory maps to check whether detected males corresponded to occupied territories. We used this buffer size (12.6 ha) because (1) it is small enough to allow the detection of all individuals present through careful and repeated telescope scanning and (2) it is large enough as to potentially include several male display territories, being comparable in size to displaying arenas during the breeding season (Ponjoan et al. 2012). After characterizing the experimental male from plumage traits, we placed the decoy in the location where the experimental male was displaying. The experimental male always flew or walked away from his display location when the decoy was placed. Once the experimental male returned, his identity was confirmed according to previously recorded plumage characteristics. In order to avoid confusion of real return events with replacement by other males, any male showing a discrepancy between before- and after-experiment recorded traits was discarded.
After placing the decoy (start of the experiment), the display site was surveyed for a maximum time of 1 h, and the experiment was stopped if the experimental male attacked the decoy. During the course of the experiment, the number of other males (including young and sub-adults) and females within the 200 m radius buffer continued to be monitored and any movement was recorded on the detailed habitat map. The maximum numbers of different males and females detected within the buffer were used as estimates of, respectively, male and female abundance in the later analyses (see below and Table 1). Regarding the experimental male behavioural response to the decoy, the following data were recorded: return time (minutes from experiment start to experimental male return) and snort call rate (calculated as the number of snort calls recorded throughout the experiment divided by total experimental male observation time in minutes). Wing flashes and jumps were also recorded, but not used in subsequent analyses due to their low frequency and correspondingly small sample size. We classified the final male response at the end of the experiment into the following five ordinal categories of increasing strength: (0) male did not return to his lekking area at all (male not returned); (1) male returned to his lekking area, but stayed away without showing any agonistic behaviour against the decoy (male returned); (2) the experimental male showed clear agonistic behaviour towards the decoy but never stayed closer than 10 m (male stayed away); (3) experimental male showed clear agonistic behaviour and stood at less than 10 m from the decoy (male stayed close); and (4) male attacked the decoy (male attacked decoy).
Habitat data
We mapped all habitats covering fields within experimental buffers. Habitats were classified according to little bustard habitat requirements (Martinez 1994; Morales et al. 2008)—herbaceous crops (cereals, leguminous and stubble fields), ploughs (ploughed fields without vegetation), natural vegetation (young fallows, that is, unploughed fields not cultivated the previous year and covered with herbaceous annual vegetation, long term fallows and pastureland), groves (vineyards, olive and almond tree groves) and others (minority crops and other habitats covering less than 10 % of each study site). We also recorded the number of fields within the 200 m radius as a measure of field border density (the more fields in the landscape, the higher border density), since field boundaries are known to function as refuge for wild plants and arthropods in arable landscapes (Belfrage et al. 2005; Gabriel et al. 2005; Romero et al. 2008), thus containing valuable resources for the little bustard and other farmland birds.
Data analysis
A principal component analysis (PCA) was performed on the relative proportions (in percent) of each habitat type (herbaceous crops, natural vegetation, groves, ploughs and others) within the 200 m radius around the experimental male positions in order to obtain a reduced number of non-correlated factors. These factors could be used as independent variables in subsequent analyses, thus avoiding colinearity in models and describing gradients of variation in habitat composition features of the agricultural landscape surrounding experimental male territories (for a similar approach, see Delgado et al. 2009; Sanza et al. 2012). A single PCA was carried out for all study sites combined. A covariance matrix was used due to its suitability for variables measured in comparable units and keeping in mind that the differences in variance between variables made an important contribution to their interpretation (Quinn and Keough 2002). These PCA factors and the rest of the variables used in subsequent analyses are summarised in Table 1.
In order to evaluate the influence of male and female abundance, along with habitat quality in terms of structure and composition, we selected three variables measuring the response of experimental males to decoys: return time, male final response and snort call rate. Potential differences between study sites in the three response variables were tested by means of general linear model (GLM) analyses in which study site was introduced as categorical factor along with the continuous explanatory variables presented in Table 1. In the models for return time and snort call rate, study site was introduced as a random factor, while in the model for final behaviour (an ordinal multinomial model, see below), it was treated as a fixed factor. Since none of the response variables varied significantly between study sites, we decided not to include this factor in subsequent analyses.
We used a type III GLM analysis (which allows testing the effect of individual variables while controlling for the effect of all other) with normal error distribution and identity link function to analyse return time in relation to male and female abundances, distance to the nearest male, number of fields and the first two components of the PCA describing habitat variation. Date (elapsing since April 1, so that date 1 = April 1) was also included to control for potential temporal effects. Continuous variables were correspondingly transformed in order to attain normality (Table 1). To avoid problems related to multicolinearity, we performed a Pearson correlation matrix analysis using all explanatory variables. All pairs of variables showed correlation coefficients smaller than 0.5 (Table S1), and thus multicolinearity was not considered relevant in the modelling procedure. We adopted a multi-model inference approach based on the Akaike Information Criterion corrected for small samples (AICc; see Burnham and Anderson 2002). Although the model yielding the lowest AICc value can be considered the best fit among all possible ones, those models whose ∆AICc (AICc i–lowestAICc) value is ≤2 are similarly plausible (Burnham and Anderson 2002). Therefore, we followed the procedure described by Burnham and Anderson (2002) to obtain a final averaged model in which parameters for each variable are averaged across the range of models with ∆AICc ≤ 2. Akaike weights (w i ), which represent the relative probability for a model I to be the best fit among selected ones, were calculated for this subset of models. Model-averaged parameter estimates (β prom) and their unconditional standard errors (USE) were calculated, along with the sum across selected models of each variable’s Akaike weights (Σw i ), which indicates the relative importance of each variable in the average model. In order to evaluate the significance of these variables, we generated a random predictor (normally distributed and non-correlated with the response variable) which was included in the model selection process along with the rest of variables. The model selection procedure was repeated 100 times so that variables whose Σw i was above the 95 % confidence interval (IC) for the average Σw i of the random predictor were considered significant (for similar approximations, see Whittingham et al. 2005; Gray et al. 2009).
Experimental male final response was analysed by means of a type III GLM with ordinal multinomial error distribution and logit link function in which male and female abundances, distance to the nearest male, number of fields, the two first habitat PCA factors and date were again used as explanatory variables, correspondingly transformed to attain normality (Table 1). The modelling approach followed the same procedure described in the return time analysis.
Finally, we analysed factors affecting experimental male snort call frequency by means of a type III GLM with normal error distribution and identity link function. Explanatory variables and their transformations were the same used in previous analyses (Table 1). Again an AICc-based model averaging approach was employed. In addition, in order to explore the relationship between snort call rate and intensity of display territory defence, we analysed snort call rate in relation to final response by means of a one-way GLM in which the latter variable was used as a categorical factor (excluding not returned males). All analyses were performed in STATISTICA 8.0 (StatSoft 2005).
Results
The PCA performed on the relative proportions of the five original habitat categories was highly explanatory, with the first two factors accounting for 86.89 % of variance in the data (Table 2). These two factors were normally distributed (Kolmogorov–Smirnov test, respectively, for F1 and F2: d = 0.12, p > 0.05 and d = 0.11, p > 0.05). The first factor (habitat F1) explained 53.30 % of the total variance and opposed ploughs and natural vegetation to herbaceous crops and can be interpreted as a gradient from areas dominated by herbaceous cultures (cereals, legumes, etc.) to areas dominated by ploughed land and natural vegetation (basically long-term fallows). The second factor (habitat F2) explained 33.59 % of variance and opposed natural vegetation to ploughs, and thus can be interpreted as a gradient from areas dominated by long-term fallows to ploughed fields, dominated by bare ground.
The multi-model inference procedure for return time of experimental males selected seven competing models with ∆AICc ≤ 2 (Table 3). The number of fields was the only variable that was not included in at least one of the selected models. According to their summed Σw i , the variables with the largest effects on the response variable were male abundance, habitat F2 and female abundance, followed by distance to the nearest male, habitat F1 and date. However, only male abundance, habitat F2 and female abundance were above the 95 % confidence interval for the average Σw i of the random predictor (Fig. 1a). Male and female abundances were negatively related to return time, while the effect of habitat F2 was positive (Table 3).
Sum of Akaike weights (Σw i ) of the variables included in those models with ∆AICc ≤ 2 analysing experimental males’ response to decoys. Solid lines indicate the mean Σw i values for random factors modeled 100 times. Dashed lines indicate the upper limit of each random factor’s 95 % confidence interval. a Results for male return time. b Results for intensity of male final response. c Results for male snort call rate
Regarding the final response of experimental males, the model selection process yielded 11 competing models with ∆AICc ≤ 2 (Table 4). All initial variables were present in at least one model, with male and female abundances, date and habitat F2 as the most weighting ones, followed by distance to the nearest male, habitat F1 and number of fields. However, only male and female abundance, date and habitat F2 were above the 95 % confidence interval for the average Σw i of the random predictor (Fig. 1b). Date and abundance of both males and females increased the final response, while habitat F2 decreased such response.
Finally, the analysis of snort call rate selected nine models (Table 5), excluding female abundance and habitat F1 from the final set. The number of fields, distance to the nearest male and habitat F2 were the most relevant variables according to their Σw i , followed by male abundance, date, habitat F1 and female abundance. Only the first three variables were clearly significant (although male abundance was marginally above the 95 % confidence interval for the average Σw i of the random predictor; see Fig. 1c), with number of fields and distance to the nearest male positively, and habitat F2 negatively related to snort call rate (Table 5). Snort call rate varied significantly among males exhibiting different final responses (F 3, 62 = 5.71, p < 0.01), with males that finally attacked the decoy yielding the lowest snort call rate (Tuckey’s HSD post hoc test, p < 0.01; see Fig. 2).
Differences in mean snort call rate (number of snort calls observed during the experiment, divided by total experimental observation time in minutes) of experimental males between the five intensity levels of response to decoy. Error bars denote 95 % confidence intervals. 1 Male returned, 2 male stayed away, 3 male stayed close, and 4 male attacked decoy
Discussion
The results of our decoy experiments with little bustards support the density dependence of the intensity of male territory defence in exploded leks. The time experimental males took to return to their display sites after decoy placement decreased with abundance of both males and females within the 200 m radius buffers. The strength of experimental males’ final response globally increased with male and female abundance in lekking areas. The significance of date in this model indicates that the final response of males intensifies as the mating season progresses, probably because the potential cost of territory loss or displacement for any particular male was higher in later dates because the later experiments took place closer to the mating season peak.
Therefore, our results add new evidence to the view that density dependence modulates breeding territorial behaviour (Sutherland 1996; Bessa-Gomes et al. 2004; Kokko and Rankin 2006) and are thus consistent with previous findings in different species of vertebrates (Grant 1997; Kokko et al. 2004; Soutullo et al. 2006; Bretagnolle et al. 2008; Fasce et al. 2011). Density dependence may be particularly important in the breeding behaviour of polygynous species, in which male intra-sexual competition is especially intense (Emlen and Oring 1977; Wittenberger 1979), giving rise to direct behavioural interference and aggression among males (Clutton-Brock et al. 1982; Gosling et al. 1987; Festa-Bianchet et al. 1990; Mysterud et al. 2005; Magaña et al. 2011). In lekking species, density dependence has a relevant role in mechanisms of lek formation and lek size regulation, as well as female attendance and female choice (Beehler and Foster 1988; Widemo and Owens 1995; Höglund and Alatalo 1995). In the particular case of the little bustard, a multiannual study by Villers (2010) in west-central France found that lek attendance, display rate, male attractiveness and spacing patterns varied with local population density. In the present study, however, we specifically studied experimentally the density dependence of territory defence by little bustard males. The positive relationship between the intensity of display territory defence and local abundance of both males and females suggests that experimental males in leks holding larger male and female numbers try to recover their territory as soon as possible in order not to lose potential access to nearby females and to avoid territory loss to neighbouring competing males. The latter may be either territorial or satellite males, although the probability of a satellite male actually engaging in a territorial dispute with an experimental territorial one can be considered very low since the proportion of satellite males in our populations is small (see “Material and methods” section), their behaviour is rather elusive (MBM, personal observation) and because satellites do not stay around territory holders of a particular lek, but move between different leks over the season (Jiguet et al. 2000; Ponjoan et al. 2012). Consequently, the potential effects of satellite males on the behavioural response of experimental males can overall be considered reduced.
Male territorial response appears to occur despite the potential costs of engaging in new agonistic interactions (including aggressions to potential competitors like the decoy). Therefore, the potential benefits of recovering their lek positions sooner can be expected to be higher than the costs. This would be consistent with density-dependent models of lek formation such as the female preference and hotshot models (Bradbury 1981; Beehler and Foster 1988; Gibson et al. 1990; Höglund and Alatalo 1995), according to which the expected per capita male mating success should increase with lek size so that both males and females tend to concentrate in larger leks (Widemo and Owens 1995). However, as argued by different authors (Beehler and Foster 1988; Kokko et al. 1998; Hernandez et al. 1999; Jiguet and Bretagnolle 2006), the female preference model predicts an optimal lek size promoting lek stability. In fact, the decoy experiments performed by Jiguet and Bretagnolle (2006) in little bustard populations of western France indicated that mate-searching females favoured an optimal lek size of four males. Our experimental design does not allow testing for optimal lek size in our populations, but the fact that the maximum number of males within experimental buffers was five points out to that direction.
Conspecific local abundance and date were not the only factors modulating the intensity of display territory defence, since habitat F2 turned out to be significant in both models, presenting a positive effect on return time and a negative one on the strength of final response. This suggests that the global response of experimental males was weaker as the dominance of bare soil habitat increased and natural vegetation cover (pastures and fallows of varying age) decreased. These results indicate that the intensity of display territory defence increases with territory quality, independently of the number of conspecifics present. Males displaying in areas with lower cover of ploughs and greater cover of natural vegetation tended to return faster to their territories and exhibited a stronger final response to the decoy. Natural, basically herbaceous, permanent and semi-permanent vegetation patches are a key habitat for breeding little bustards in extensive mosaic cereal steppes, both for displaying males and for nesting and chick rearing females (Martinez 1994; Delgado et al. 2010; Silva 2010; Lapiedra et al. 2011; Ponjoan et al. 2012; Morales et al. 2013; Tarjuelo et al. 2013), where they can find the critical resources required by each sex (mainly food for males and food and shelter for females and families; Jiguet et al. 2000, 2002; Morales et al. 2008; Traba et al. 2008; Faria et al. 2012a, b). Therefore, the proportion of cover of natural vegetation in the lekking areas can be used as a proxy of lek habitat quality.
These relationships with habitat quality suggest that resources play a role in the little bustard mating system as reported for several exploded lekking species (Höglund and Alatalo 1995; Ligon 1999; Kotrschal and Taborsky 2010). In fact, little bustard males are known to occupy food-rich territories in order to afford the costs of mating activity (Jiguet et al. 2000, 2002; Traba et al. 2008; Faria et al. 2012b), and females may forage in these territories while visiting leks, and may even nest in them (Morales et al. 2013), although they do not seem to use their quality as a cue for mate choice, at least in western France (Jiguet et al. 2000, 2002). Results presented here support the existence of active resource defence by lekking little bustard males. Previous works have suggested that the little bustard mating system might vary according to population density and resource availability, shifting from exploded leks to resource-based leks and even solitary polygyny if population density declines and resources diminish or are sparsely distributed (Jiguet et al. 2000; Morales et al. 2001). Such variability has also been observed in other lekking species like the fallow deer (Appolonio et al. 1989; Clutton-Brock and Parker 1992). In the case of our study species, that would be likely to happen in very intensive agricultural habitats with low bustard density (Jiguet et al. 2000; Morales et al. 2001). In our moderately intensive study areas, little bustard density ranges from low to moderate and this could be related with the significant effect of habitat quality found in this study, although the lack of correlation between habitat variables and female abundance (Table S1) suggests that female attendance at this stage may not be driven by habitat. Likewise, the effect of habitat quality on territorial defence could be weaker or null in more homogenous, grassland-dominated landscapes harbouring high-density populations like those found in south-western Iberia (Silva et al. 2014). In any case, testing for the existence of a truly resourced-based polygynous system requires a specific experimental design to evaluate whether resource control by males increases their mating success or correlates with female choice (Jiguet et al. 2002).
The increase in snort call rate with decreasing level of male aggregation shown by our results, together with the fact that snort call rate was lowest among the males that finally attacked the decoy (see Fig. 2), suggests that territories held by higher rate calling males could be safer to aggression associated to sexual harassment and copulation disruption, which are important driving forces of female mating dynamics in lekking species (Isvaran and St. Mary 2003; Jiguet and Bretagnolle 2006; Magaña et al. 2011). Snort call rate decreased with the cover of ploughs described by habitat F2 and, contrarily, increased with the number of fields within buffers, suggesting that habitat quality influences the snort call rate of experimental males once they return to their display territories. Moreover, snort call rate was positively associated with the number of fields, which relates with border density and thus with natural herbaceous vegetation providing higher food and shelter availability (Belfrage et al. 2005; Gabriel et al. 2005; Romero et al. 2008), which indicates higher effort in territorial signalling when territory quality is higher. These results, together with the positive association of snort call rate with distance to the nearest male, may imply that snort calls have an inter-sexual function involved in the attraction of potential mates to good quality territories, contradicting the mainly intra-sexual role of snort calls proposed by Jiguet and Bretagnolle (2001). The fact that more aggregated males display a lower snort call rate may also indicate that clustered males do not need to call so frequently because small male aggregations are themselves attractive to females as described by Jiguet and Bretagnolle (2006) who found an optimal size for little bustard leks of four males. Another, non-exclusive, possibility is that close neighbours are considered by territory holders a small threat compared to distant competitors, consistent with the ‘dear enemy’ hypothesis (Temeles 1994). In any case, the function of snort calling in the little bustard (intra-sexual vs. inter-sexual) remains unclear and needs to be discerned through additional experiments.
In summary, the results of our experimental study only partially supported the general hypothesis that little bustard males defend more intensely territories with higher conspecific density. The defence was more intense in leks with higher male and female abundance. However, the intensified response to growing male aggregation was not accompanied by higher sexual display. Therefore, prediction 1 was only partially supported. In addition, a more intense territorial response was associated with higher availability of good-quality habitat, thus giving support to prediction 2. These results provide further support to the importance of resource defence in little bustard exploded leks, at least in the moderately intensified Spanish mosaic cereal steppes holding low to medium densities of the species.
In the theoretical framework of lek evolution, exploded leks represent an intermediate stage in the continuum that ranges from classical leks, in which males are highly clumped and resources are irrelevant for female choice and male maintenance, to resource-based polygyny, in which the direct benefits obtained by females and their offspring in terms of resources controlled by spatially dispersed territorial males play a role in female choice (Höglund and Alatalo 1995). Although less pronounced, male clustering in exploded leks is still important and that leads to the density dependence of male behaviour, including spatial distribution, lek attendance patterns, display rate and territory defence (Clutton-Brock and Parker 1992; Widemo and Owens 1995; Magaña et al. 2011). In addition, density dependence of male territorial defence may favour the existence of an optimal lek size preferred by females in order to minimize harassment and copulation disruption, as predicted by the female preference model of lek evolution (Beehler and Foster 1988; Jiguet and Bretagnolle 2006). Our experiments in an exploded lekking species add further support to these theoretical expectations and demonstrate the effect of conspecific abundance and habitat quality on male territorial behaviour.
References
Andersson M (1994) Sexual selection. Princeton University Press, Princeton
Appolonio M, Festa-Bianchet M, Mari F (1989) Effects of removal of successful males in a fallow deer lek. Ethology 83:320–325
Arroyo B, Bretagnolle V (1999) Field identification of individual Little bustard Tetrax tetrax males using plumage patterns. Ardeola 46:53–60
Beehler BM, Foster MS (1988) Hotshots, hotspots and female preferences in the organization of lek mating systems. Am Nat 131:203–219
Belfrage K, Björklund J, Salomonsson L (2005) The effects of farm size and organic farming on diversity of birds, pollinators, and plants in a Swedish landscape. Ambio 34:582–588
Bessa-Gomes C, Legendre S, Clobert J (2004) Alle effects, mating systems and the extinction risk in populations with two sexes. Ecol Lett 7:802–812
Bradbury JW (1981) The evolution of leks. In: Alexander RD, Tinkle DW (eds) Natural selection and social behaviour. Chiron Press, New York, pp 138–169
Bretagnolle V, Mougeot F, Thibault JC (2008) Density dependence in a recovering osprey population: demographic and behavioural processes. J Anim Ecol 77:998–1007
Burnham KP, Anderson DR (2002) Model selection and multimodel inference: a practice information-theoretic approach. Springer, New York
Clutton-Brock TH, Parker GA (1992) Potential reproductive rates and the operation of sexual selection. Q Rev Biol 67:437–456
Clutton-Brock TH, Guiness FE, Albon SD (1982) Red deer: the behaviour and ecology of two sexes. University of Chicago Press, Chicago
Cramp S, Simmons KEL (1980) The birds of the Western Palearctic, vol II. Oxford University Press, Oxford
Del Hoyo J, Elliot A, Sargatal J (1996) Handbook of the birds of the world, vol III. Lynx Edicions, Barcelona
Delgado MP, Morales MB, Traba J, García de la Morena EL (2009) Determining the effects of habitat management and climate on the population trends of a declining steppe birds. Ibis 151:440–451
Delgado MP, Traba J, García de la Morena EL, Morales MB (2010) Habitat selection and density-dependent relationships in spatial occupancy by male little bustards Tetrax tetrax. Ardea 98:185–194
Emlen ST, Oring LW (1977) Ecology, sexual selection and the evolution of mating systems. Science 197:215–223
Faria N, Rabaça J, Morales MB (2012a) The importance of grazing regime in the provision of breeding habitat for grassland birds: the case of the endangered little bustard (Tetrax tetrax). J Nat Conserv 20:211–218
Faria N, Rabaça J, Morales MB (2012b) Linking plant composition and arthropod abundance to establish little bustard breeding requirements in pastureland dominated landscapes. Biodivers Conserv 21:2109–2125
Fasce P, Fasce L, Villers A, Bergese F, Bretagnolle V (2011) Long-term breeding demography and density dependence in an increasing population of Golden Eagles Aquila chrysaetos. Ibis 153:581–591
Festa-Bianchet M, Apollonio M, Mari F, Rasola G (1990) Aggression among lekking male fallow deer (Dama dama): territory effects and relationship with copulatory success. Ethology 85:236–246
Gabriel D, Thies C, Tscharntke T (2005) Local diversity of arable weeds increases with landscape complexity. Perspect Plant Ecol 7:85–93
García de la Morena EL, Bota G, Ponjoan A, Morales MB (2006) El sisón común en España. I Censo Nacional (2005). SEO⁄BirdLife, Madrid
Gibson RM, Taylor CE, Jefferson DR (1990) Lek formation by female choice: a simulation study. Behav Ecol 1:36–42
Gilliard ET (1969) Birds of paradise and bowerbirds. Weidenfeld and Nicholson, London
Gosling LM, Petrie M, Rainey ME (1987) Lekking in topi: a high cost specialist strategy. Anim Behav 35:616–618
Grant JA (1997) Territoriality. In: Godin JGJ (ed) Behavioural ecology of teleost fishes. Oxford University Press, Oxford, Oxford, pp 81–103
Gray TNE, Borey R, Hout SK, Chamman H, Collar NJ, Dolman PM (2009) Generality of models that predict the distribution of species: conservation activity and reduction of model transferability for a threatened bustard. Conserv Biol 23:433–439
Hernandez ML, Houston AI, McNamara JM (1999) Male rank and optimal lek size. Behav Ecol 10:73–79
Höglund J, Alatalo RV (1995) Leks. Princeton University Press, New York
Isvaran K, St. Mary CM (2003) When should males lek? Insights from a dynamic state variable model. Behav Ecol 14:876–886
Jiguet F, Bretagnolle V (2001) Courtship behaviour in a lekking species: individual variations and settlement tactics in male little bustard. Behav Process 55:107–118
Jiguet F, Bretagnolle V (2006) Manipulating lek size and composition using decoys: an experimental investigation of lek evolution models. Am Nat 168:758–768
Jiguet F, Bretagnolle V (2014) Sexy males and choosy females on exploded leks: correlates of male attractiveness in the Little Bustard. Behav Process 103:246–255
Jiguet F, Ollivier D (2002) Male phenotypic repeatability in the threatened Little Bustard Tetrax tetrax: a tool to estimate turnover and dispersal. Ardea 90:43–50
Jiguet F, Arroyo B, Bretagnolle V (2000) Lek mating systems: a case study in the Little Bustard Tetrax tetrax. Behav Process 51:63–82
Jiguet F, Jaulin S, Arroyo B (2002) Resource defence on exploded leks: do male little bustards, Tetrax tetrax, control resources for females? Anim Behav 63:899–905
Kokko H, Rankin DJ (2006) Lonely hearts or sex in the city? Density-dependent effects in mating systems. Philos Trans R Soc B 361:319–334
Kokko H, Sutherland WJ, Lindström J, Reynolds JD, MacKenzie A (1998) Individual mating success, lek stability, and the neglected limitations of statistical power. Anim Behav 56:755–762
Kokko H, Harris MP, Wanless S (2004) Competition for breeding sites and site-dependent population regulation in a highly colonial seabird, the common guillemot Uria aalge. J Anim Ecol 73:367–376
Kotrschal A, Taborsky B (2010) Resource defense or exploded Lek? A question of perspective. Ethology 116:1189–1198
Lapiedra O, Ponjoan A, Gamero A, Bota G, Mañosa S (2011) Brood ranging behaviour and breeding success of the threatened little bustard in an intensified cereal farmland area. Biol Conserv 144:2882–2890
Ligon JD (1999) The evolution of avian breeding systems. Oxford University Press, Oxford
López-Sepulcre A, Kokko H (2005) Territorial defense, territory size, and population regulation. Am Nat 166:317–329
Magaña M, Alonso JC, Palacín C (2011) Age-related dominance helps reduce male aggressiveness in great bustard leks. Anim Behav 82:203–221
Martinez C (1994) Habitat selection by the Little Bustard Tetrax tetrax in cultivated areas of central Spain. Biol Conserv 67:125–128
Morales MB, Jiguet F, Arroyo B (2001) Exploded leks: what bustards can teach us. Ardeola 48:85–98
Morales MB, García JT, Arroyo B (2005) Can landscape composition changes predict spatial and annual variation of little bustard male abundance? Anim Conserv 8:167–174
Morales MB, Traba J, Carriles E, Delgado MP, la García D, de la Morena EL (2008) Sexual differences in microhabitat selection of breeding little bustards Tetrax tetrax: ecological segregation based on vegetation structure. Acta Oecol 34:345–353
Morales MB, Traba J, Delgado MP, García de la Morena EL (2013) The use of fallows by nesting little bustard Tetrax tetrax females: implications for conservation in mosaic cereal farmland. Ardeola 60:85–97
Mysterud A, Solberg EJ, Yoccoz NG (2005) Ageing and reproductive effort in male moose under variable levels of intra-sexual competition. J Anim Ecol 74:742–754
Olea PP, Casas F, Redpath S, Viñuela J (2010) Bottoms up: great bustards use the sun to maximise signal efficacy. Behav Ecol Sociobiol 64:927–937
Orians GH (1969) On the evolution of mating systems in birds and mammals. Am Nat 103:589–603
Ponjoan A, Bota G, Mañosa S (2012) Ranging behaviour of little bustard males, Tetrax tetrax, in the lekking grounds. Behav Process 91:35–40
Quinn GP, Keough MJ (2002) Experimental design and data analysis for biologists. Cambridge University Press, Cambridge
Romero A, Chamorro L, Sans FX (2008) Weed diversity in crop edges and inner fields of organic and conventional dryland winter cereal crops in NE Spain. Agr Ecosyst Environ 124:97–104
Sanza MA, Traba J, Morales MB, Rivera D, Delgado MP (2012) Effects of landscape, conspecifics and heterospecifics on habitat selection by breeding farmland birds: the case of Calandra Lark (Melanocorypha calandra) and Corn Bunting (Emberiza calandra). J Ornithol 153:525–533
Schulz H (1986) Agonistic behaviour, territorial behaviour and courtship display of the Little Bustard (Tetrax tetrax). J Ornithol 127:125–204
Silva JP (2010) Factors affecting the abundance of the Little Bustard Tetrax tetrax: implications for conservation. PhD Thesis, University of Lisbon, Lisbon
Silva JP, Estanque B, Moreira F, Palmeirim JM (2014) Population density and use of grasslands by female Little Bustards during lek attendance, nesting and brood-rearing. J Ornithol 155:53–63
Soutullo A, Limiñana R, Urios V, Surroca M, Gill JA (2006) Density dependant regulation of population size in colonial breeders: allee and buffer effects in the migratory Montagu’s harrier. Oecologia 149:543–552
StatSoft Inc (2005) STATISTICA 7.1. www.statsoft.com
Sutherland WJ (1996) From individual behaviour to population ecology. Oxford University Press, Oxford
Tarjuelo R, Delgado MP, Bota G, Morales MB, Traba J, Ponjoan A, Hervás I, Mañosa S (2013) Not only habitat but also sex: factors affecting spatial distribution of Little Bustard Tetrax tetrax families. Acta Ornithol 48:119–128
Temeles EJ (1994) The role of neighbours in territorial systems: when are they ‘dear enemies’? Anim Behav 47:339–350
Traba J, Morales MB, García de la Morena EL, Delgado MP, Krištín A (2008) Selection of breeding territory by little bustard (Tetrax tetrax) males in Central Spain: the role of arthropod availability. Ecol Res 23:615–622
Villers A (2010) Écologie spatiale, processus comportementaux et dynamiques des populations d'une espèce menacée, l'outarde canepetière. PhD Thesis, Université de Paris VI, Paris
Whittingham MJ, Swetnam RD, Wilson JD, Chamberlain DE, Freckleton RP (2005) Habitat selection by yellowhammers (Emberiza citrinella) on lowland farmland at two spatial scales: implications for conservation management. J Appl Ecol 42:270–280
Widemo F, Owens YPF (1995) Lek size, male mating skew and the evolution of lekking. Nature 373:148–151
Wittenberger JF (1979) The evolution of mating systems in birds and mammals. In: Marler P, Vandenbergh JG (eds) Social behavior and communication. Springer, New York, pp 271–349
Wolff A, Paul JP, Martin JL, Bretagnolle V (2002) The benefits of extensive agriculture to birds: the case of the little bustard. J Appl Ecol 38:963–975
Acknowledgments
Particular thanks are due to Vincent Bretagnolle and Frédéric Jiguet for lending their little bustard decoys for experiments. We thank all farmers, hunting managers and gamekeepers of our study areas for allowing us to work on their properties. Salvador Luna, Laura Iglesias, Juan Bécares, Núria Pocino, Sergi Ricart, Pau Ferrer and María Castañeda collaborated during some stages of the work. FC and JM-P were supported by a JAE-Doc contract funded by Spanish Research Council and the European Social Fund (ESF), and ELG was funded by a FPU grant from the Spanish Ministry of Education. The comments of two anonymous reviewers sensibly improved the first version of the manuscript. This paper contributes to projects CGL2004-06147-C02-01, CGL2004-06147-C02-02 and CGL2009-13029/BOS of the Spanish Ministry of Science, as well as to the REMEDINAL2 network of the Community of Madrid (S-2009/AMB/1783). MBM, FC and GB contributed equally to the final outcome of the present study.
Ethical standards
The experiments here described complied with the laws of Spain and the regions (Catalonia, Castilla-La Mancha and Madrid) where they were performed.
Author information
Authors and Affiliations
Corresponding author
Additional information
Communicated by J. A. Graves
Manuel B. Morales, Fabián Casas, and Gerard Bota contributed equally to this work.
Rights and permissions
About this article
Cite this article
Morales, M.B., Casas, F., García de la Morena, E. et al. Density dependence and habitat quality modulate the intensity of display territory defence in an exploded lekking species. Behav Ecol Sociobiol 68, 1493–1504 (2014). https://doi.org/10.1007/s00265-014-1758-z
Received:
Revised:
Accepted:
Published:
Issue Date:
DOI: https://doi.org/10.1007/s00265-014-1758-z