Abstract
While both sperm number and quality are now recognized to be important in determining the outcome of sperm competition, very few studies have experimentally assessed the influence of these two parameters simultaneously. We studied the effect of sperm quality and number on competitive fertilization success in an internal-fertilizing fish, the guppy (Poecilia reticulata), which is characterized by high levels of sperm competition. We artificially inseminated virgin females with varying proportion of sperm from two competing males, while holding constant the total number of sperm transferred to the female. Sperm morphology and sperm swimming velocity were also determined prior to insemination. The paternity outcome of sperm competition trials was assessed through molecular analyses of the resulting offspring using polymorphic microsatellite loci. We found that both sperm number and sperm velocity affected the outcome of sperm competition, with males that contributed more and faster sperm achieving a greater paternity share.
Similar content being viewed by others
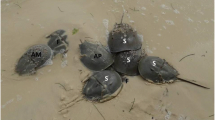
Avoid common mistakes on your manuscript.
Introduction
Sperm number is widely recognized to affect male reproductive success especially in sexually promiscuous species where sperm competition occurs. Sperm competition is widespread in fish and occurs when ejaculates of different males compete for the fertilization of a given set of eggs (Parker 1970). Sperm competition has been classically viewed as a raffle in which the probability of fertilization is strongly related to the number of sperm a male contributes to the competition (Parker 1990). Support for this idea has been found in several taxa (for a review, see Wedell et al. 2002). Evidence comes from comparative studies (reviewed in Birkhead and Møller 1998), as well as from selection experiment (Hosken et al. 2001), where a positive association between the degree of polyandry, and hence sperm competition, and relative testis size has been found. A second line of evidence comes from empirical studies in which it has been demonstrated that for some species, the higher the risk of sperm competition, the larger the quantity of sperm allocated to a given mating. That, for example, males copulating in the presence of rival males increase the amount of sperm transferred has been strongly substantiated by a number of studies (e.g. Gage 1991; Gage and Barnard 1996; Marconato and Shapiro 1996; Pizzari et al. 2003; Pound and Gage 2004), supporting the sperm competition risk hypothesis (Parker 1998).
Recently, it has been pointed out that sperm number is not sufficient to explain the variance in sperm competition success among males (Snook 2005). Apart from the possible influences of the genetic background in which sperm compete (i.e. female–male genetic interactions), several different sperm traits, other than sperm number, have been proposed to account for the different fertilizing abilities among males. Together, these sperm traits are called ‘sperm quality traits’ and include characteristics such as sperm morphology and velocity. A growing body of work has revealed that sperm velocity is associated with fertilization success in, for example, sea urchins (Levitan 2000), fish (Burness et al. 2004; Gage et al. 2004; Liljedal et al. 2008; Rurangwa et al. 2004; Skjaeraasen et al. 2009; Gasparini et al. 2010a), birds (Birkhead et al. 1999; Denk et al. 2005) and mammals (Malo et al. 2005; Moore and Akhondi 1996). Attention has also been given to sperm morphology, principally because it is believed to influence sperm velocity. However, results from both experimental and comparative studies (in which sperm traits were related to the level of sperm competition) yielded contrasting results (see for example Anderson et al. 2005; Briskie and Montgomerie 1992; Gage et al. 2004; Garcia-Gonzalez and Simmons 2007; Schulte-Hostedde and Millar 2004; Simmons et al. 2003).
The above evidence makes clear that both sperm number and sperm quality traits (in particular sperm velocity) play an important role in the outcome of sperm competition and, in turn, affect male reproductive success. What has been poorly investigated is the role of sperm quality and sperm number simultaneously. Indeed, in the majority of works, sperm quality traits revealed their importance in sperm competition trails in which competitors contributed the same number of sperm. Despite the potential importance of this topic for our understanding of the evolution of ejaculate traits and of the mechanisms of sperm competition, only two studies, as far as we know, have investigated the relative role of sperm quality in experiments which varied the number of sperm inseminated. These studies, performed in external fertilizer fish, yielded contrasting results (Gage et al. 2004; Stoltz and Neff 2006).
To investigate what role sperm number and sperm quality play in the outcome of sperm competition, the guppy is particularly suited for several reasons. Poecilia reticulata is a species of live-bearing freshwater fish with internal fertilization characterized by especially high level of sperm competition (Houde 1997; Magurran 2005), and therefore a high selective pressure on sperm traits is expected due to the fitness returns on a male's reproductive success. It has been shown that more highly coloured males achieve greater paternity in sperm competition trials even when the number of sperm contributed by competitors was held constant (Evans et al. 2003b), indicating that paternity in this species is not determined by sperm number alone. Males have also been shown to vary in their sperm quality according to male phenotype (Locatello et al. 2006; Pitcher et al. 2007), and sperm velocity shows a certain degree of plasticity as it can be rapidly modulated by individuals according to the perceived mating opportunity (Gasparini et al. 2009). Interestingly, by modulating copula duration (Pilastro et al. 2007), guppy females can limit the number of sperm received during consensual matings as a means of favouring the most attractive males (Pilastro et al. 2004). Surprisingly, despite the huge attention given to post-copulatory sexual selection and its mechanisms in this species (Houde 1997; Magurran 2005), specific studies aimed at understanding whether, and to what extent, sperm number and quality influence fertilization ability are still lacking, even though studies focused on sperm quality traits, in particular sperm velocity, assume that these traits have effects on male reproductive success (Evans 2009; Gasparini et al. 2009; Locatello et al. 2006; Pitcher et al. 2007; Skinner and Watt 2007).
The aim of this study was to examine the influence of sperm number and quality (velocity and morphology) on the outcome of sperm competition in this species. Virgin females were artificially inseminated using different numbers of sperm from two competing males, in proportions that represent the range of sperm transferred during natural mating. Sperm morphology and sperm swimming velocity were also assessed before insemination. DNA microsatellite markers were used to assign paternity to the resulting offspring.
Methods
Study population
The fish used were descendants of wild-caught fish from the lower part of Tacarigua River in Trinidad (national grid reference PS 787 804). Males were reared in stock tanks with a 1:1 sex ratio, whereas virgin females were kept in single-sex tanks. All tanks were maintained under the same conditions of temperature (26 ± 1°C) and light (12:12-h light/dark cycle), and fish were fed a mixed diet of brine shrimp nauplii (Artemia salina) and commercially prepared flake food (Duplarin).
Sperm collection and measurement of male colour pattern
Fully sexually mature males were randomly chosen from stock tanks. Following a 3-day isolation period, to ensure the complete replenishment of sperm reserves (Pilastro et al. 2004), sperm were collected from each male following an established procedure (Evans et al. 2003b). Briefly, each male was anaesthetized in a water bath containing MS-222 and placed on a slide under a stereomicroscope. A gentle abdominal pressure allowed the release of sperm in a drop of saline solution (NaCl 0.9%). Sperm in this species are packaged in discrete units, called sperm bundles, that can be easily collected with a pipette. Sperm bundles were split into different aliquots for subsequent analyses (sperm quality assays, see below) and for the artificial insemination of females. Following sperm extraction, a digital photo was taken of each male alongside a reference ruler (Nikon CoolPix 4500). We used image analysis software (UTHSCSA Image Tool, University of Texas Health Science Centre, San Antonio, TX, USA; http://ddsdx.uthscsa.edu/dig/download.html) to measure standard length (SL; from the snout to the base of the caudal fin), body area (including caudal fin) and the area of coloured spots. Coloration included patches of different colour: orange and yellow (hereafter orange), melanistic (black), and blue and green (iridescent). The relative area (percentage of body area) of each colour spot was used in the analyses. Using the absolute area of colour spots yielded nearly identical results in the subsequent analyses, as body size shows little variation in this population, and absolute and relative size of colour spots are very similar (correlation between absolute and relative area of colour spots: orange, r = 0.97; melanistic, r = 0.94; iridescent, r = 0.97; n = 34). A tissue sample was collected from the caudal fin of each male and stored appropriately for subsequent molecular analysis.
Artificial insemination
Each sperm competition trial involved two males and one virgin female. Each experimental female was artificially inseminated with a constant total amount of sperm bundles (20 bundles, containing on average 21,000 ± 7,000 SD sperm), but in different proportions between two competing males according to the experimental group: (1) 10 sperm bundles from each male, (2) 8 vs. 12 sperm bundles, and (3) 4 vs. 16 sperm bundles. The actual number of sperm inseminated was calculated a posteriori from the estimated number of sperm contained in the bundles of each male (see below). Males were randomly assigned to the three experimental groups and arbitrarily labelled, within each pair, as male A and B. The number of sperm used for artificial inseminations lies in the natural range of variation of sperm bundles transferred during cooperative and coercive matings (Pilastro et al. 2004, 2008; range 2–33 sperm bundles, see Pilastro and Bisazza 1999).
The use of artificial insemination allowed us to control the number of sperm inseminated and to avoid other confounding factors, such as the order of mating or any maternal effects. We used virgin females to avoid sperm storage from previous matings. After anaesthetization with MS-222, the sperm bundles previously collected from the males were transferred into the female's gonoduct with a plastic micropipette (Drummond). Immediately after insemination, females were revived in a 5-l plastic container (containing freshwater, gravel, live and plastic plants) where they remained isolated until parturition. After birth, tissue samples from the female's caudal fin and from the whole newborn's body (humanely killed with an excess of MS-222) were collected and stored appropriately for paternity analysis.
Sperm quality assays and sperm count
Previous studies demonstrated that each sperm bundle contains an approximately equal number of sperm and do not correlate with male colouration or body size (Evans et al. 2003b) or age (Gasparini et al. 2010b). Therefore, counting the number of sperm bundles is straightforward to control the number of sperm cells. However, to improve the accuracy of our estimate of the number of sperm inseminated, we collected 20 sperm bundles from those remained from each experimental male after the artificial insemination and estimated the actual number of sperm per bundle using a haematocytometer (Evans et al. 2003b; Gasparini et al. 2010b). Sperm bundles contained on average 21,000 ± 7,000 SD sperm (range, 10,000–33,000). The proportion of sperm inseminated by male B was then calculated from the number of bundles used for AI and the estimated number of sperm per bundle for each male. The proportion of the actual sperm inseminated ranged between 0.106 and 0.812 (mean proportion = 0.471 ± 0.051 SE) and was highly correlated with the proportion of bundles inseminated (r = 0.91, P < 0.0001, n = 17). Not surprisingly, the two values yielded nearly identical results as predictors of sperm competition success in the following analyses. Considering that the proportion of sperm inseminated is a more direct estimate of the number of sperm from the two males competing for fertilising the eggs, we presented only the results obtained with this predictor.
To assess sperm morphology, sperm cells were incubated with the vital staining 1% Rose Bengal for about 20 min. Digital photos of the sperm samples were then taken at ×1,000 magnification and photographed with a digital camera (Olympus DP10, Japan). Using image analysis software, we measured the length (to the nearest 0.01 μm) of the head, midpiece and flagellum (in micrometres) of ten sperm for each male.
Sperm velocity was analysed using a Hamilton–Thorne computer-aided semen analyser (IVOS, Hamilton–Thorne Research, Beverly, MA, USA) and included three commonly used parameters: VAP (average path velocity, micrometres per second), VSL (straight line velocity, micrometres per second) and VCL (curvilinear velocity, micrometres per second). VAP and VSL are highly correlated in guppies (Gasparini et al. 2010b; this sample, r = 0.99, n = 34, P < 0.001), and only VAP and VCL (r = 0.75, n = 34, P < 0.001) were therefore considered. Immediately after sperm collection, we placed 20 sperm bundles into 10 μl of extender medium (207 mM NaCl, 5.4 mM KCl, 1.3 mM CaCl2, 0.49 mM MgCl2, 0.41 mM MgSO4, 10 mM Tris, pH 7.5) in which sperm remain quiescent (Gardiner 1978). The sample was maintained in this solution at 3–5°C until needed (about 3 h of collection), at which point it was warmed to 26°C (this temperature corresponds to the water temperature at which fish are maintained) and activated with a 40-μl solution of 150 mM KCl and 2 mg/ml bovine serum albumin (BSA) to avoid sperm sticking to the slide (Billard and Cosson 1990). Following activation, the sperm bundles were gently broken to induce motility, and a sample (5 μl) was placed in disposable 12-μm deep microcell chambers and immediately analysed (time between activation and analysis, <30 s). Sperm velocity analyses were based on an average of 78.29 ± 29.77 SD motile sperm per sample (range, 28–147, n = 34).
Paternity analysis
Paternity was determined using three polymorphic microsatellite loci specifically isolated for poeciliids: TTA GenBank accession number, AF164205 (Taylor et al. 1999); Pooc-G49 GenBank accession number, AF026459 (Parker et al. 1998); and Pr92 GenBank accession number, AF467906 (Becher et al. 2002). Genomic DNA was extracted using a Chelex protocol (Walsh et al. 1991) for adults and newborns. Polymerase chain reaction (PCR) amplifications were performed on a GeneAmp® PCR System 2700 Thermocycler (Applied Biosystems). The PCR was performed in 15 μl reaction volumes with 1.2 μl MgCl2, 0.525 μl dNTPs, 0.15 μl of each primer, 1.5 μl Taq buffer, 0.08 μl Taq DNA polymerase (Promega) and 2.4 μl DNA template. The cycling protocol included an initial denaturation step at 95°C for 1 min, followed by 27 cycles (10 s at 95°C for denaturation, 30 s at 52°C for annealing −60°C for Pooc-G49 and 30 s at 72°C for elongation), and a final extension for 5 min at 72°C. PCR products were visualized on a 1.8% agarose gel. The forward primers were labelled with one of three fluorescent dyes (FAM, HEX and TAMRA) for sequence analysis on an ABI 3100 sequencer (ABI PRISM, Applied Biosystems), using 400 HD ROX (Perkin-Elmer, Applied Biosystems) as a size standard (http://www.bmr-genomics.com). Allele sizes were determined using Peak Scanner Software v 1.0 (Applied Biosystems). Paternity was assigned to offspring according to allele sharing between putative sires, mother and offspring. The first two loci were highly variable in our sample (14 and 36 alleles). The third locus was less informative and was used when offspring could not be assigned unambiguously to one of the two potential sires using the first two loci. We calculated the relative share of paternity between the two males of the dyad, expressed as the proportion of offspring sired by the second male of the dyad (PB).
Statistical analysis
The association between sperm number, sperm quality traits (sperm velocity and morphology) and paternity (PB) was tested using a logistic regression with binomial error and logit-link function, in which the number of offspring sired by male B was the dependent variable, brood size was the binomial total and male body size, male coloration, sperm number and sperm quality traits were the predictors (see below). To test the effect of the predictors, we used an information theoretic (IT) approach (Burnham and Anderson 2002; Lukacs et al. 2010). To do so, we first calculated from all the possible models with zero (i.e. intercept only) to all ten predictors (n = 1,024), the Akaike information criterion corrected for small samples and binomial distribution (quasi-AICc) and the regression coefficient (b) of each predictor included in the model, using the function RSEARCH in GenStat. We then ranked the models according to their AICc values (the smallest the best). We used a model-averaging approach that considers all the possible models and weighs each model on the basis of its likelihood (Burnham and Anderson 2002), to calculate average regression coefficients and their 95% confidence intervals. Model averaging admits from the beginning of the analysis that there is substantial uncertainty as to what model is best and what combination of variables is important. Practically, for each model is calculated a likelihood to be the best model as a function of the difference from its AICc value and the minimum AICc (also called ‘Akaike weight’, see formula (3) in Lukacs et al. 2010). For each predictor, an average parameter (regression coefficient b, in the present case) is calculated by summing the parameter in each model, including those which did not selected the predictor (in this case, set = 0), weighted for the likelihood of each model (total weights sum to 1). The ‘Akaike weight’ is an estimate of the probability that a given model is the best model of all the n models. This estimator is a type of shrinkage estimator, which gives less weight to the predictors with a weak relationship to the response (Lukacs et al. 2010). Following this procedure, we obtained an average b value for each of the ten predictors. To have an indication of the relative importance of each predictor, we repeated this analysis after standardising the predictors (covariates) so that they have mean 0 and variance 1. This provides a common scale on which the effect sizes of the predictors can be compared. Regression coefficients whose 95% CI did not include 0 were considered statistically significant.
Statistical analyses were performed using SPSS v. 15.0 (SPSS Inc., Chicago, IL, USA) and GenStat v. 12 (VSN International Ltd, Hemel Hempstead, UK). All probabilities are two-tailed.
Results
Descriptive statistics of male sperm traits are reported in Table 1. A total of 17 females (group 1, n = 5; group 2, n = 5; group 3, n = 7), each inseminated with sperm collected from two different males (34 males in total), successfully gave birth. We obtained a total of 186 offspring with an average brood size of 10.94 ± 4.79 SD offspring (range, 4–21). We were able to assess the paternity of all but two of the offspring (these from two different broods) due to low quality of their genomic DNA, and our analysis was therefore performed on 184 offspring. Mean PB was 0.61 ± 0.37 SD (range, 0–1; variance = 0.134).
We conducted a logistic regression model in which the number of offspring sired by male B over the binomial total was the dependent variable, and the proportion of sperm from male B over the total number of sperm used for artificial insemination and the difference in the ten predictors relating to male body size and colour, sperm velocity (VAP and VCL) and sperm morphology (head, midpiece and total length) the predictors. Using the proportion of sperm bundles inseminated instead of the proportion of sperm inseminated did not substantially change the results (data not shown). This analysis was repeated using only predictors representing a direct measure of sperm quality (i.e. male body size and colours were excluded) and including only one measure of sperm velocity (VCL), as the two measures of sperm velocity are highly correlated (r = 0.75, P < 0.001).
Sperm number was the most important predictor of sperm competition success in both the full model and the restricted model (Table 2; Fig. 1). In the latter, regression coefficient of sperm velocity also was positive, and its 95% CI did not encompass 0. The analysis of the effect sizes (standardised regression coefficients) confirmed that the effect of sperm number was on average as twice as larger than that of sperm velocity.
Proportion of offspring sired by male B in relation to (a) the proportion of sperm inseminated from male B on the total sperm inseminated and (b) the difference in sperm velocity (VCL) between male B and male A. The size of the points is proportional to the number of offspring per brood (range, 4–21)
Discussion
Our results revealed that sperm number is the main predictor of sperm competition success in the guppy. Sperm competition success is also positively influenced, although to a lesser extent, by sperm velocity (VCL). Furthermore, IT approach suggests that other sperm quality predictors (sperm morphology, male body size and area of colour spots) were indeed not as important in determining sperm competition success, at least in our sample. The analyses based on the standardised predictors confirmed that the effect of sperm number was consistently larger, in both full and restricted models, than that of sperm velocity. Natural variation in the predictors considered was comparable with that observed in our sample (see Table 1), with the exception of sperm number, for which a larger variation is observed in natural ejaculate size (number of sperm transferred during natural copulations, Pilastro et al. 2007) than in our experimental conditions. It can therefore be expected that the effect of the number of sperm is even more important after natural copulations. This prediction is also supported by the larger variance in sperm competition success observed after natural copulations (Evans and Magurran 2001; Pitcher et al. 2003), as compared with that observed in the present study. Altogether, these results therefore allow to conclude that males that transfer more sperm during copulation and produce sperm with faster swimming speeds are expected to fertilize a greater proportion of eggs in a competitive context.
While statistically robust, these conclusions cannot be generalised without some caution. Firstly, the small sample size does not allow to exclude that some of the non-significant predictors may actually have an effect on sperm competition success, but their effect is too small to be visible unless a larger dataset is considered. Secondly, with small sample size, as a consequence, not all the phenotypic variation is explored. Indeed, the range of phenotypic variation in the present study is comparable, for most traits examined, to that observed in previous studies (see above), but it is actually smaller for other traits, for example, relative area of black spots and midpiece length (Table 1). Thirdly, we explored the relative importance of sperm number and quality only at one sperm concentration (20 sperm bundles, corresponding to an average of 417.8 ± 118.4 × 103 sperm). The sperm concentration used in this experiment was chosen on the basis of the data on natural ejaculates and represents the most likely condition under which sperm competition may occur in this species (Pilastro et al. 2002, 2004, 2007; Pilastro and Bisazza 1999). However, copulations can occasionally transfer much smaller and much larger ejaculates (see references above and Table 1). It cannot be excluded, for example, that at lower sperm concentrations, sperm velocity become relatively more important than sperm number. Guppy males can inseminate females at any time with respect to females' time of ovulation (Evans et al. 2003a; Pilastro et al. 2007; Pilastro and Bisazza 1999), and sperm remain viable for months in the female oviduct (Evans and Pilastro 2011). Thus, the time elapsing between insemination and fertilisation can vary, in natural conditions, between hours and several months. The relative competitive value of an ejaculate may change over the time during which competing ejaculates are stored within the reproductive tract of a female (e.g. Pizzari et al. 2008). How sperm number and sperm quality traits affect sperm competition success in the guppy, when a long time elapses between insemination and fertilisation, remains to be tested. As a final point, it has also to be stressed that in vitro sperm velocity may not reflect in vivo velocity. For example, longer sperm may not swim in vitro as fast as they do in more natural conditions due to steric constraints determined by the limited depth of the slide chamber (12 μm).
Previous studies aimed at assessing the relative importance of sperm number and quality in fishes have been limited to external fertilizers, with contrasting results. The primary determinants of sperm competition outcome is sperm velocity in the salmon Salmo salar (Gage et al. 2004), while is sperm number in the bluegill Lepomis macrochirus (Stoltz and Neff 2006). These results are probably associated with spawning ecology, as salmon spawn in flowing water while in the bluegill, gametes are released in steady water, and these differences may therefore favour different aspects of sperm traits (Stoltz and Neff 2006). Similarly, the effect of sperm number on sperm competition success differs also in other taxa, such as birds (positive correlation, Martin et al. 1974) and crickets (no correlation, Simmons et al. 2003). Sperm velocity is generally considered a good predictor of sperm competition success (Birkhead et al. 1999; Denk et al. 2005; Gage et al. 2004; Snook 2005), with a few exceptions (e.g. Dziminski et al. 2009). Moreover, a recent study (Gasparini et al. 2010a) found a positive association between sperm velocity and competitive fertilization success in the green swordtail Xiphophorus helleri, a related poeciliids fish. An effect of sperm velocity on sperm competition success in the guppy was expected, as it has been shown that colourful male guppies achieve a greater proportion of paternity in sperm competition trials when females are artificially inseminated with an equal number of sperm from two males (Evans et al. 2003b) and colourful males produce sperm with higher swimming velocity (Locatello et al. 2006; Pitcher et al. 2007; but see Skinner and Watt 2007). Results from this study add to previous evidence that pre- and post-copulatory success work together in this species.
The correlation between sperm size and sperm competition success at the intraspecific level is more controversial (Humphries et al. 2008). While interspecific comparison suggests that longer sperm evolve in response to sperm competition (e.g. Byrne et al. 2003; Gomendio and Roldan 1991; Morrow and Gage 2000), evidence from intraspecific studies suggests that sperm size is rarely correlated with sperm competition success (Gage et al. 2004; Schulte-Hostedde and Millar 2004; Simmons et al. 2003; Tomkins and Simmons 2000) and, when so, sperm size negatively affects sperm competition success (Firman and Simmons 2008a; Gage and Morrow 2003; Garcia-Gonzalez and Simmons 2007; Morrow and Gage 2001). In contrast, positive correlation between sperm size and sperm competition success has been found in species with ameboid sperm (LaMunyon and Ward 1998; Radwan 1996). In other cases, sperm size failed to predict sperm competition success. We found no significant effect of sperm morphology on sperm competition success, suggesting that guppies seem to follow the pattern found in other species with flagellate sperm. Sperm head length has been shown to be positively correlated with sperm swimming speed in another guppy population (Pitcher et al. 2007), suggesting that sperm size may be a more important predictor of sperm competition in other guppy populations. In our sample, sperm head length was not significantly associated with sperm velocity (VCL, r = −0.03, P = 0.86, n = 34; VAP, r = −0.25, P = 0.15, n = 34). Similarly, sperm head-to-tail ratio, which has been suggested to be a better predictor of sperm velocity, at least in external fertilisers (Humphries et al. 2008), does not correlate with sperm velocity measures in our sample (all r < 0.32, P > 0.18, n = 34), nor is it a significant predictor of sperm competition success (data not shown). Clearly, the role of sperm morphology in sperm competition success in the guppy deserves further investigation.
Our results in the guppy suggest that sperm competition generates a selection pressure on males both to produce competitive ejaculates and to transfer large number of sperm during copulation, in line with the pattern of intraspecific (e.g. Firman and Simmons 2008b) and interspecific (Fitzpatrick et al. 2009; Kleven et al. 2009; Snook 2005) comparisons, showing that increased sperm number and velocity are usually associated with increasing levels of sperm competition. It is interesting to note that in the guppy, females mated with a single male do not show a reduced fecundity even when only two sperm bundles are transferred, suggesting that female reproductive success is not sperm-limited (Pilastro et al. 2008). In nature, however, females usually mate with several males, and multiple paternity is the rule in this species (Hain and Neff 2007; Neff et al. 2008), and the number of sperm transferred by males during copulation is expected to have a profound effect on male fitness. Indeed, the number of sperm transferred during copulation can vary dramatically (Pilastro and Bisazza 1999), and it is, at least to some extent, under female control. Female guppies prefer to mate with males with larger colour spots (see Houde (1997) and references therein for other guppy populations and Evans et al. (2004) for the population used in this study) and are able to limit the number of sperm transferred according to their pre-copulatory mating preference (Pilastro et al. 2002, 2004), by regulating copula duration (Pilastro et al. 2007). The results of the present study, coupled with those of previous studies, indicate that females, by accepting a higher proportion of sperm from the most preferred males, will effectively bias paternity towards them. In line with this conclusion, males have been shown to adjust their sperm traits by increasing both sperm production (‘sperm priming’) and sperm velocity in response to increased mating opportunities (Bozynski and Liley 2003; Gasparini et al. 2009).
References
Anderson MJ, Nyholt J, Dixson AF (2005) Sperm competition and the evolution of sperm midpiece volume in mammals. J Zool 267:135–142
Becher SA, Russell ST, Magurran AE (2002) Isolation and characterization of polymorphic microsatellites in the Trinidadian guppy (Poecilia reticulata). Mol Ecol Notes 2:456–458
Billard R, Cosson MP (1990) The energetics of fish sperm motility. In: Gagnon C (ed) Controls of sperm motility: biological and clinical aspects. CRC Press, Boca Raton, pp 155–173
Birkhead TR, Møller AP (1998) Sperm competition and sexual selection. Academic, London
Birkhead TR, Martinez JG, Burke T, Froman DP (1999) Sperm mobility determines the outcome of sperm competition in the domestic fowl. Proc R Soc Lond B 266:1759–1764
Bozynski CC, Liley NR (2003) The effect of female presence on spermiation, and of male sexual activity on ‘ready’ sperm in the male guppy. Anim Behav 65(Part 1):53–58
Briskie JV, Montgomerie R (1992) Sperm size and sperm competition in birds. Proc R Soc Lond B 247:89–95
Burness G, Casselman SJ, Schulte-Hostedde AI, Moyes CD, Montgomerie R (2004) Sperm swimming speed and energetics vary with sperm competition risk in bluegill (Lepomis macrochirus). Behav Ecol Sociobiol 56:65–70
Burnham KP, Anderson DR (2002) Model selection and multimodel inference: a practical information-theoretic approach. Springer, New York
Byrne PG, Simmons LW, Roberts JD (2003) Sperm competition and the evolution of gamete morphology in frogs. Proc R Soc Lond B 270:2079–2086
Denk AG, Holzmann A, Peters A, Vermeirssen ELM, Kempenaers B (2005) Paternity in mallards: effects of sperm quality and female sperm selection for inbreeding avoidance. Behav Ecol 16:825–833
Dziminski MA, Roberts JD, Beveridge M, Simmons LW (2009) Sperm competitiveness in frogs: slow and steady wins the race. Proc R Soc B 276:3955–3961
Evans JP (2009) No evidence for sperm priming responses under varying sperm competition risk or intensity in guppies. Naturwissen 96:771–779
Evans JP, Magurran AE (2001) Patterns of sperm precedence and predictors of paternity in the Trinidadian guppy. Proc R Soc B 268(1468):719–724
Evans JP, Pilastro A (2011) Postcopulatory sexual selection. In: Evans JP, Pilastro A, Schlupp I (ed) Ecology and evolution of poeciliid fishes. Chicago University Press, Chicago (in press)
Evans JP, Pilastro A, Ramnarine IW (2003a) Sperm transfer through forced matings and its evolutionary implications in natural guppy (Poecilia reticulata) populations. Biol J Linn Soc 78:605–612
Evans JP, Zane L, Francescato S, Pilastro A (2003b) Directional postcopulatory sexual selection revealed by artificial insemination. Nature 421:360–363
Evans JP, Bisazza A, Pilastro A (2004) Female mating preferences for colourful males in a population of guppies subject to high predation. J Fish Biol 65:1154–1159
Firman RC, Simmons LW (2008a) Polyandry, sperm competition, and reproductive success in mice. Behav Ecol 19:695–702
Firman RC, Simmons LW (2008b) The frequency of multiple paternity predicts variation in testes size among island populations of house mice. J Evol Biol 21:1524–1533
Fitzpatrick JL, Montgomerie R, Desjardins JK, Stiver KA, Kolm N, Balshine S (2009) Female promiscuity promotes the evolution of faster sperm in cichlid fishes. Proc Natl Acad Sci USA 106:1128–1132
Gage MJG (1991) Risk of sperm competition directly affects ejaculate size in the Mediterranean fruit fly. Anim Behav 42:1036–1037
Gage MJG, Barnard CJ (1996) Male crickets increase sperm number in relation to competition and female size. Behav Ecol Sociobiol 38:349–353
Gage MJG, Morrow EH (2003) Experimental evidence for the evolution of numerous, tiny sperm via sperm competition. Curr Biol 13:754–757
Gage MJG, Macfarlane CP, Yeates S, Ward RG, Searle JB, Parker GA (2004) Spermatozoal traits and sperm competition in Atlantic salmon: relative sperm velocity is the primary determinant of fertilization success. Curr Biol 14:44–47
Garcia-Gonzalez F, Simmons LW (2007) Shorter sperm confer higher competitive fertilization success. Evolution 61:816–824
Gardiner DM (1978) Utilization of extracellular glucose by spermatozoa of two viviparous fishes. Comp Biochem Physiol A 59A:165–168
Gasparini C, Peretti A, Pilastro A (2009) Effects of mating opportunities on sperm allocation in guppies (Poecilia reticulata). Biol Lett 5:792–794
Gasparini C, Simmons LW, Beveridge M, Evans JP (2010a) Sperm swimming velocity predicts competitive fertilization success in the green swordtail Xiphophorus helleri. PLoS ONE 5(8):e12146
Gasparini C, Marino IAM, Boschetto C, Pilastro A (2010b) Effect of male age on sperm traits and sperm competition success in the guppy (Poecilia reticulata). J Evol Biol 23:124–135
Gomendio M, Roldan ERS (1991) Sperm competition influences sperm size in mammals. Proc R Soc Lond B 243:181–185
Hain TJA, Neff BD (2007) Multiple paternity and kin recognition mechanisms in a guppy population. Mol Ecol 16:3938–3946
Hosken DJ, Garner TWJ, Ward PI (2001) Sexual conflict selects for male and female reproductive characters. Curr Biol 11:489–493
Houde AE (1997) Sex, color, and mate choice in guppies. Princeton University Press, Princeton
Humphries S, Evans JP, Simmons LW (2008) Sperm competition: linking form to function. Bmc Evol Biol 8:319
Kleven O, Fossoy F, Laskemoen T, Robertson RJ, Rudolfsen G, Lifjeld JT (2009) Comparative evidence for the evolution of sperm swimming speed by sperm competition and female sperm storage duration in passerine birds. Evolution 63:2466–2473
LaMunyon CW, Ward S (1998) Larger sperm outcompete smaller sperm in the nematode Caenorhabditis elegans. Proc R Soc Lond B 265:1997–2002
Levitan DR (2000) Sperm velocity and longevity trade off each other and influence fertilization in the sea urchin Lytechinus variegatus. Proc R Soc B 267:531–534
Liljedal S, Rudolfsen G, Folstad I (2008) Factors predicting male fertilization success in an external fertilizer. Behav Ecol Sociobiol 62:1805–1811
Locatello L, Rasotto MB, Evans JP, Pilastro A (2006) Colourful male guppies produce faster and more viable sperm. J Evol Biol 19:1595–1602
Lukacs PM, Burnham KP, Anderson DR (2010) Model selection bias and Freedman's paradox. Ann Inst Stat Math 62:117–125
Magurran AE (2005) Evolutionary ecology: the Trinidadian guppy. Oxford University Press, Oxford
Malo AF, Garde JJ, Soler AJ, Garcia AJ, Gomendio M, Roldan ERS (2005) Male fertility in natural populations of red deer is determined by sperm velocity and the proportion of normal spermatozoa. Biol Repr 72:822–829
Marconato A, Shapiro DY (1996) Sperm allocation, sperm production and fertilization rates in the bucktooth parrotfish. Anim Behav 52:971–980
Martin PA, Reimers TJ, Lodge JR, Dziuk PJ (1974) The effect of ratios and numbers of spermatozoa mixed from two males on proportions of offspring. J Repr Fertil 39:251–258
Moore HDM, Akhondi MA (1996) Fertilizing capacity of rat spermatozoa is correlated with decline in straight-line velocity measured by continuous computer-aided sperm analysis: epididymal rat spermatozoa from the proximal cauda have a greater fertilizing capacity in vitro than those from the distal cauda or vas deferens. J Androl 17:50–60
Morrow EH, Gage MJG (2000) The evolution of sperm length in moths. Proc R Soc Lond B 267:307–313
Morrow EH, Gage MJG (2001) Sperm competition experiments between lines of crickets producing different sperm lengths. Proc R Soc Lond B 268:2281–2286
Neff BD, Pitcher TE, Ramnarine IW (2008) Inter-population variation in multiple paternity and reproductive skew in the guppy. Mol Ecol 17:2975–2984
Parker GA (1970) Sperm competition and its evolutionary effect on copula duration in fly Scatophaga stercoraria. J Insect Physiol 16:1301–1328
Parker GA (1990) Sperm competition games: raffles and roles. Proc R Soc Lond B 242:120–126
Parker GA (1998) Sperm competition and the evolution of ejaculates: towards a theory base. In: Birkhead TR, Møller AP (eds) Sperm competition and sexual selection. Academic, London, pp 1–54
Parker KM, Hughes K, Kim TJ, Hedrick PW (1998) Isolation and characterization of microsatellite loci from the Gila topminnow (Poeciliopsis o. occidentalis) and their utility in guppies (Poecilia reticulata). Mol Ecol 7:361–363
Pilastro A, Bisazza A (1999) Insemination efficiency of two alternative male mating tactics in the guppy (Poecilia reticulata). Proc R Soc Lond B 266:1887–1891
Pilastro A, Evans JP, Sartorelli S, Bisazza A (2002) Male phenotype predicts insemination success in guppies. Proc R Soc Lond B 269:1325–1330
Pilastro A, Simionato M, Bisazza A, Evans JP (2004) Cryptic female preference for colorful males in guppies. Evolution 58:665–669
Pilastro A, Mandelli M, Gasparini C, Dadda M, Bisazza A (2007) Copulation duration, insemination efficiency and male attractiveness in guppies. Anim Behav 74:321–328
Pilastro A, Gasparini C, Boschetto C, Evans JP (2008) Colorful male guppies do not provide females with fecundity benefits. Behav Ecol 19:374–381
Pitcher TE, Neff BD, Rodd FH, Rowe L (2003) Multiple mating and sequential mate choice in guppies: females trade up. Proc R Soc B 270(1524):1623–1629
Pitcher TE, Rodd FH, Rowe L (2007) Sexual colouration and sperm traits in guppies. J Fish Biol 70:165–177
Pizzari T, Cornwallis CK, Lovlie H, Jakobsson S, Birkhead TR (2003) Sophisticated sperm allocation in male fowl. Nature 426:70–74
Pizzari T, Worley K, Burke T, Froman DP (2008) Sperm competition dynamics: ejaculate fertilising efficiency changes differentially with time. Bmc Evol Biol 8:332
Pound N, Gage MJG (2004) Prudent sperm allocation in Norway rats, Rattus norvegicus: a mammalian model of adaptive ejaculate adjustment. Anim Behav 68:819–823
Radwan J (1996) Intraspecific variation in sperm competition success in the bulb mite: a role for sperm size. Proc R Soc Lond B 263:855–859
Rurangwa E, Kime DE, Ollevier F, Nash JP (2004) The measurement of sperm motility and factors affecting sperm quality in cultured fish. Aquaculture 234:1–28
Schulte-Hostedde AI, Millar JS (2004) Intraspecific variation of testis size and sperm length in the yellow-pine chipmunk (Tamias amoenus): implications for sperm competition and reproductive success. Behav Ecol Sociobiol 55:272–277
Simmons LW, Wernham J, Garcia GF, Kamien D (2003) Variation in paternity in the field cricket Teleogryllus oceanicus: no detectable influence of sperm numbers or sperm length. Behav Ecol 14:539–545
Skinner AMJ, Watt PJ (2007) Phenotypic correlates of spermatozoon quality in the guppy, Poecilia reticulata. Behav Ecol 18:47–52
Skjaeraasen JE, Mayer I, Meager JJ, Rudolfsen G, Karlsen O, Haugland T, Kleven O (2009) Sperm characteristics and competitive ability in farmed and wild cod. Mar Ecol Prog Ser 375:219–228
Snook RR (2005) Sperm in competition: not playing by the numbers. Trends Ecol Evol 20:46–53
Stoltz JA, Neff BD (2006) Sperm competition in a fish with external fertilization: the contribution of sperm number, speed and length. J Evol Biol 19:1873–1881
Taylor JS, Durkin JMH, Breden F (1999) The death of a microsatellite: a phylogenetic perspective on microsatellite interruptions. Mol Biol Evol 16:567–572
Tomkins JL, Simmons LW (2000) Sperm competition games played by dimorphic male beetles: fertilization gains with equal mating access. Proc R Soc Lond B 267:1547–1553
Walsh PS, Metzger DA, Higuchi R (1991) Chelex-100 as a medium for simple extraction of DNA for PCR-based typing from forensic material. Biotechniques 10:506–513
Wedell N, Gage MJG, Parker GA (2002) Sperm competition, male prudence and sperm-limited females. Trends Ecol Evol 17:313–320
Acknowledgements
We wish to thank four anonymous referees and Kai Lindström for their comments to various versions of this manuscript. This research was funded by grants from the University of Padova (Progetto di Ateneo CPDA031972) and the CARIPARO (Progetto di Eccellenza 2007) to A.P. The experiments were carried out in conformity with the relevant Italian laws governing the care of animals in research (D.L. 116/27-01-92, C.M.S. 8/22-04-94).
Author information
Authors and Affiliations
Corresponding author
Additional information
Communicated by K. Lindström
Rights and permissions
About this article
Cite this article
Boschetto, C., Gasparini, C. & Pilastro, A. Sperm number and velocity affect sperm competition success in the guppy (Poecilia reticulata). Behav Ecol Sociobiol 65, 813–821 (2011). https://doi.org/10.1007/s00265-010-1085-y
Received:
Revised:
Accepted:
Published:
Issue Date:
DOI: https://doi.org/10.1007/s00265-010-1085-y