Abstract
In the paper we present our results for element sensitive high-energy X-ray tomography and high-speed thermal neutron tomography applied to geological samples. The tuneable monochromatic X-ray beam was used for element sensitive tomography of geological samples containing heavy elements using the K-edge dichromatic scanning technique. This type of NDT (non-destructive testing) allowed us to reveal the quantity and location of uranium, lead and mercury in Oklo samples (natural nuclear reactor) and Cinnabar stones. Fast 3-D thermal neutron imaging of dynamical processes was successfully tested at the high flux neutron tomography station of the ILL. The qualitative investigation of water repellents and consolidants applied on sandstones of Bray and limestones of Maastricht were the first samples to be studied with the new imaging technique.
Similar content being viewed by others
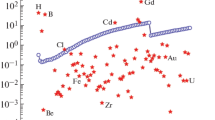
Avoid common mistakes on your manuscript.
Introduction
The interesting properties of X-rays for imaging have been well known since they were discovered about 100 years ago, in 1895, by W.H. Röntgen. Since 1973, X-ray tomography has become popular with the invention of the CT-scanner by Hounsfield (1973). X-ray imaging is still evolving, e.g. phase-contrast imaging, submicron tomography. Also, on the technological side, many developments were realised, e.g. X-ray optics, micro focus X-ray tubes, high resolution scintillators. More information about recent development in tomography can be read in the paper by Rau and others (2001). Here, we focus on computed tomography (CT) with a high-energy (70–116 keV), tuneable monochromatic X-ray tomography applied to geological samples. With this type of X-ray beam it is possible to identify both location and quantity of specific elements in geological objects, something that is not possible with a polychromatic or broad spectrum X-ray beam.
Synchrotron radiation facilities are the ideal instrument for this kind of research due to their enormous X-ray production yield. On the other hand, these facilities show some disadvantages: there are only 16 facilities in Europe and beam time has to be planned a long time in advance. Our research was done at the high-energy beam-line at the European Synchrotron Radiation Facility (ESRF) in France to study the uranium distribution in a rock sample from the natural fission reactor Oklo in Gabon.
In contrast to X-ray imaging, the possibilities of neutrons for imaging are less known. There are indeed fewer neutron sources and they are much more complicated. The most used facilities for neutron imaging are nuclear research reactors, followed by neutron spallation sources, which are accelerator-driven sources. Because the properties of neutrons differ widely from X-rays, a complementary range of applications can be found. Due to the different nature of interactions and the complementary Z-dependent cross sections, X-rays are mainly used to monitor samples that contain light elements whereas neutrons are interesting probes for the investigation of samples containing light elements such as hydrogen, lithium, boron and aluminium.
Both, monochromatic X-ray and neutron imaging techniques use the exponential attenuation law:
with μ the energy-dependent linear attenuation coefficient and I0 the intensity of the beam without attenuation.
The differences between X-rays and neutrons, relevant to imaging, are shown in Table 1.
Experimental set-up used for computed tomography
The standard procedure for tomography is to measure the attenuation of radiation through a sample from different orientations. The recorded patterns are used to reconstruct the 3-D attenuation distribution for the whole sample. A completely automated transportable measurement set-up was developed, of which a schematic presentation can be seen in Fig. 1. After interacting with the sample, the X-rays are converted to visible light in a scintillating screen. To protect the CCD camera from damaging radiation, the scintillator (YAG) is observed via a surface coated mirror. The entire system is shielded with lead and lead glass. The spatial image resolution is 12 μm.
In cases of neutron tomography, a second mirror is used to reduce the γ and neutron background radiation in the direction of the CCD camera. The neutron-to-visible light converter, manufactured by Applied Scintillation Technologies, consisted of a dispersion of ZnS(Ag) and 6LiF in an acrylic binder. The detection mechanism is based on the 6Li(n,α)3H nuclear interaction. The α’s and the tritons interact with the phosphor to create scintillation events that can be detected by the CCD camera. The conversion screen emits a visible light spectrum with a maximum intensity at 460 nm. More information can be found on the Web at http://www.appscintech.com/products/neutron-protection-screens.html. The advantage of this converter is the relatively low sensitivity to background γ radiation. This ZnSLiF layer is deposited onto a reflecting aluminium plate to increase the intrinsic efficiency. The thickness of the converter is 420 µm. To increase the resolution, thinner scintillators were tested, see Baechler and others (2001).
Results of monochromatic X-rays for element sensitive tomography
Principle
Heavy elements have large photon absorption discontinuities around the energies corresponding to the binding energy of the atomic electrons with the nucleus. These discontinuities are called absorption edges. The abrupt change of the X-ray attenuation around the edge, together with the fact that the K-shell electron binding energy is different for every element, makes it possible to perform element-sensitive radiographs.
High-energy X-rays have two advantages. For energies below 511 keV, the X-ray absorption coefficient decreases with energy. Therefore, hard X-rays can penetrate thicker or denser samples than low energy X-rays. Heavy elements have K-edges at high energy with large discontinuities, which makes them extremely suitable for element sensitive tomography based on dual energy scanning. Another advantage of monochromatic X-ray beams in comparison with white or polychromatic beams is the absence of beam hardening. Hardening of the beam leads to artefacts that are very difficult to correct.
By using a crystal monochromator it is possible to change the photon energy in a continuous way by changing the Bragg angle.
Synchrotron radiation source ESRF
For all experiments at the ESRF, a super-conducting wavelength shifter at ID15 was used. There are three permanent filters in the beam (i.e. 0.7 mm C, 4.0 mm Be, 4.1 mm Al). Beam intensity was 5.13×1013 photons s-1 mrad–2, 0.1% energy bandwidth, 0.1 A at 95 keV. The energy resolution was 1 keV. The polychromatic X-ray beam was converted into a monochromatic beam by means of two 5-mm-thick asymmetrically cut Si crystals operating in fixed Laue-Laue mode.
Element sensitive X-ray microtomography applied to Oklo
A number of samples from the natural fission reactor Oklo in Gabon were studied with tuneable monochromatic X-ray tomography to determine the uranium and lead distribution. With X-ray absorption tomography it is not possible to separate the isotope 235U from the isotope 238U. Therefore the same Oklo samples were also scanned with position-sensitive prompt gamma activation analysis (PGAA). Natural uranium has always the same isotopic composition: 99.27% uranium-238, 0.72% uranium-235 and traces of uranium-234. The uranium samples from the Oklo mine showed a lack of 235U, which can only be explained by some process other than simple radioactive decay. In 1972, a French physicist Francis Perrin formulated the theory of the first nuclear reactor, Smellie (1995).
Figure 2 shows a radiography of the Oklo stone with monochromatic X-rays at 87 keV, together with the result of an element-sensitive tomography reconstruction of the U-distribution (b) and the Pb-distribution (c), respectively. The lead in the sample is generated by the radioactive decay of 238U. A PGAA scan of the same Oklo sample shows the relative distribution of the 235U and the relative distribution of the 238U (both relative to the maximum concentration in the sample). In natural uranium-containing samples both bars should look identical. This is clearly not the case for this Oklo sample, which therefore proves this sample was part of a natural nuclear reactor.
Sample from a natural nuclear reactor of Oklo in Gabon. a Radiograph at 87 keV, b 3-D reconstruction of the uranium distribution, c 3-D reconstruction of the lead distribution, d bars show the individual distributions of 235U and 238U (respectively, left and right) measured with PGAA. Resolution of the PGAA-scan was 1 mm. Colour of the images corresponds to density, while darker means higher density. Absolute values are not indicated
Element-sensitive X-ray micro tomography applied to Cinnabar stone
The second sample submitted to element-sensitive tomography is a cinnabar stone from a mercury mine in Tuscany (Italy). Using a monochromatic X-ray beam with energy just above the K-edge of mercury, the absorption contrast is very high. This allows to clearly identify the mercury (the layer on top of the sample). With a dual energy scan, above and below the K-edge of mercury, the mercury in the stone can be visualised and its exact mercury density and quantity determined (Fig. 3b). Indeed, after reconstruction, atomic density ρ(Hg), the attenuation coefficient µ(E)/ρ for mercury and the sample dimension are known.
Some commercial X-ray devices also use a dual energy technique to identify objects within samples by changing the high-voltage on the X-ray tube. Additionally, dedicated image processing is used to separate different objects superimposed on one another in the projected image. The measured densities are compared with library values of the densities of known materials. This method is nowadays commonly used in airports for the detection of weapons and explosives.
More results on monochromatic X-ray tomography can be found in Bonse and others (1986), Jolie and others (1999), Materna and others (1999), Masschaele and others (2001a).
Results of neutrons for geology and structural engineering
Deterioration of natural building stones is a widely spread phenomenon. To minimise the rate of stone decay, water repellents are applied in order to reduce or prevent water penetration into the stones. Due to weathering, the cement between the grains of natural building stones is dissolved. At this point a consolidant will be applied, whose major function will be to re-establish cohesion between particles. Consolidants are intended to strengthen weakened stone and slow down the rate of surface loss by binding loosened grains. Because every type of natural building stone has its own petrophysical characteristics, they will react differently on the various restoration products available on the market. Determination of the penetration depth of restoration products into natural building stones is crucial if application of conservation products is planned. Several test methods, such as measuring of the water droplet absorption time, visual observation of colour changes on wetting and drying, water vapour permeability or strength tests (Young and others 1999), try to determine the penetration depth by measuring superficial characteristics. Because water lowers the contrast between fluids and stone, water repellents and consolidants are hard to visualise with X-rays; therefore, thermal neutron radiography was tested.
For this study, porous local Belgian natural building stones, such as sandstone of Bray and limestone of Maastricht, were selected. Both types were chosen because of their high porosity and their pure (mono-) mineralogical composition. The sandstone of Bray is a quartz arenite of Upper-Landenian age (Palaeocene, Tertiary). This natural building stone was used for the construction of different important monuments in Aulné, Binche, Bray and Mons (all Province of Hainaut, Belgium). The limestone of Maastricht (Sibberstone) is a yellow highly porous bioclastic limestone (Formation of Maastricht, Maastrichtian, Upper Cretaceous) that can be found in southern Limbourg (Belgium) and in the region of Maastricht (the Netherlands).
The measurements were made at the 58 MW nuclear reactor of the ILL in Grenoble (France). The tomography station has the highest neutron intensity for neutron imaging in the world. The specifications are detailed in Table 2.
Although the radiographical spatial resolution is not optimal due to the large beam divergence, high-speed thermal neutron radiography and tomography are ideal tools to study dynamical processes, provided that the neutron beam flux is high.
More information about the neutron tomography can be found in Masschaele and others (2001b) and on the Web site http://.ssf.ugent.be/linac/linac/tomography/resultsILL.htm.
3-D neutron imaging to monitor fluid movement in building materials
Neutron radiography has been used in the past for the visualisation of fluids in porous media by a number of research teams: Prazak and others (1990), Jasti and Fogler (1992), Pel and others (1993). In a first test, we demonstrated that the uptake of water repellents and consolidants inside the stone could be visualised with neutrons. And secondly, due to the very high neutron flux, we showed that tomography of these processes is possible.
A Maastricht limestone sample with a porosity of 52.1% had been treated with the water repellent Hydro 10 (produced by FTB Restoration, Belgium). The sample size was 38 mm3. Its right side was treated with water repellent. The neutron radiography aimed at testing if water also penetrated into the treated part of the limestone (Fig. 4). Consecutive pictures at known time intervals allowed us to determine the speed of water uptake.
Figure 5 plots water level and water uptake speed as a function of time. Water uptake speed was retrieved by differentiating a fifth order polynomial fit through the water level data points. Even after 2 h water could not be detected in the treated part of the limestone. This could either mean that the sensitivity of the technique is not sufficient or that indeed no water penetrated into the treated part of the stone. Although at first sight this seems qualitative, the neutron imaging technique can also perform quantitative analyses. But more experiments are needed in order to establish a solid standard technique.
Figure 6 indicates the result of the 3-D evolution of the water level in a Ytong building stone (autoclaved aerated concrete). These results were obtained after tomographic reconstruction with the software package Octopus. More information about Octopus can be found in Dierick and Masschaele (2002) and on the Web at http://ssf.rug.ac.bc/linac.
In this type of building stone, the outside material has a higher water uptake speed than the interior part. This result suggests we need to be careful with visual inspection of water absorption in stones as their outside aspect cannot be used to draw conclusions about the bulk absorption. To our knowledge this is the first time that fluid movement has been visualised with neutrons in 3-D.
Discussion
Both monochromatic X-rays and neutrons show interesting properties for tomography applications in the field of geosciences. Element-sensitive X-ray tomography can be applied as a non-destructive testing (NDT) technique for heavy element detection, while neutron imaging is an interesting tool for the visualisation of fluid uptake in natural building stones. As neutrons and X-rays have completely different attenuation coefficients for the same elements, they are complementary to one another. In neutron tomography, the image spatial resolution is at present limited to 100 µm (Lehmann and others 1999). This high value is due to the conversion screen thickness, neutron beam divergence and neutron scattering in the samples. However, due to its NDT character, neutron tomography has a large potential to monitor fluid dynamics in various types of porous rocks such as natural building stones.
References
Baechler S, Materna T, Jolie J, Cauwels P, Crittin M, Honkimaki V, Johner HU, Masschaele B, Mondelaers W, Kern J, Piboule M (2001) Non-destructive analysis of a bulky sample from a natural fossil reactor. J Radioanal Nucl Chem 250:39
Bonse U, Johnson Q, Nichols M, Nußhardt R, Krasnicki S, Kinney J (1986) High resolution tomography with chemical specificity, Nucl Instrum Methods A246:644–648
Dierick M, Masschaele B (2002) Advanced filtered back projection algorithms. Proc World Conference on Neutron Radiography (WNCR-7)
Hounsfield GN (1973) Computerized transverse axial scanning (tomography), 1, description of system. Brit J Radiol 46:1016–1022
Jasti JK, Fogler HS (1992) Application of neutron radiography to image flow phenomena in porous media. AIChE J 38:481–488
Jolie J, Materna T, Masschaele B, Mondelaers W, Honkimaki V, Koch A, Tschentscher T (1999) Heavy element sensitive tomography using synchrotron radiation above 100 keV. Speciation, Techniques and Facilities for Radioactive Materials at Synchrotron Light Sources Eds. Nuclear Energy Agency, 249
Lehmann EH, Vontobel P, Wiezel L (1999) Properties of the radiography facility NEUTRA at SINQ and its potential for use as European reference facility. Proc 6th World Conf on Neutron Radiography, Osaka
Masschaele B, Beachler S, Cauwels P, Cloetens P, Dierick M, Jolie J, Mondelaers W (2001a) Element sensitive tomography for the determination of the metal diffusion in teeth with amalgam fillings. Radiat Phys Chem 61:625–626
Masschaele B, Beachler S, Cauwels P, Dierick M, Jolie J, Mondelaers W (2001b) First results of micro-tomography by use of a focusing neutron lens. Radiat Phys Chem 61:623–624
Masschaele B (2002) The use of monochromatic X-rays and cold neutrons for tomography. PhD Thesis, Ghent University
Materna T, Jolie J, Mondelaers W, Masschaele B, Honkimaki V, Koch A, Tschentscher T (1999) Uranium sensitive tomographies with synchrotron radiation. J Synchrotron Radiat 6:1059–1064
Pel L, Ketelaars AAJ, Adan OCG, van Well AA (1993) Determination of moisture diffusivity in porous media using scanning neutron radiography. Int J Heat Mass Transfer 36:1261–1267
Prazak J, Tywoniak J, Peterka F, Slonc T (1990) Description of transport of liquid in porous media — a study based on neutron radiography data. Int J Heat Mass Transfer 33:1105–1120
Rau C, Weitkamp T, Snigirev A, Schroer CG, Tümmler J, Lengeler B (2001) Recent developments in hard X-ray tomography. Nucl Instr Meth A 467–468:929–931
Smellie J (1995) The fossil nuclear reactors of Oklo, Gabon. Radwaste Magazine, Special Series on Natural Analogs, March 1995:21
Young ME, Murray M, Cordiner P (1999) Stone consolidants and chemical treatments in Scotland. Report to Historic Scotland
Author information
Authors and Affiliations
Corresponding author
Rights and permissions
About this article
Cite this article
Masschaele, B., Dierick, M., Van Hoorebeke, L. et al. The use of neutrons and monochromatic X-rays for non-destructive testing in geological materials. Env Geol 46, 486–492 (2004). https://doi.org/10.1007/s00254-004-1050-z
Received:
Accepted:
Published:
Issue Date:
DOI: https://doi.org/10.1007/s00254-004-1050-z