Abstract
The objective of this study was to set-up a pilot plant and to evaluate its effectiveness for biological nitrogen and organic matter removal from tannery wastewater in Ethiopia. A pilot wastewater treatment plant consisting of a predenitrification-nitrification process was constructed and operated for 6 months. This was fed with a raw tannery wastewater obtained from the Modjo Tannery located 70 km south of the capital, Addis Ababa. Up to 98% total nitrogen and chemical oxygen demand, and 95% ammonium nitrogen removal efficiencies were achieved in the system. The average effluent ammonium nitrogen ranged from 8.4 mg l−1 to 86.0 mg l−1, whereas the average effluent for nitrate nitrogen ranged from 2.9 mg l−1 to 4.4 mg l−1. The average values of denitrification and nitrification rates determined by nitrate and ammonium uptake rates (NUR and AUR) were 8.0 mg NO3-N [g volatile suspended solids (VSS)]−1 h−1 and 5.4 mg NH4-N (g VSS)−1 h−1, respectively, demonstrating that the treatment processes of the pilot plant were effective. Further studies of the effect of chromium III on AUR showed 50% inhibition at a concentration of 85 mg l−1, indicating that this metal was not causing process inhibition during performance operations. Thus, the predenitrification-nitrification process was found to be efficient for simultaneous removal of nitrogen and organic substrates from tannery wastewaters.
Similar content being viewed by others
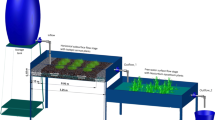
Explore related subjects
Discover the latest articles, news and stories from top researchers in related subjects.Avoid common mistakes on your manuscript.
Introduction
Tannery wastewaters are very complex and are characterised by high contents of organic and inorganic nitrogenous compounds, chromium (III), sulphides (S2−), suspended and dissolved solids [United Nations Environment Program (UNEP) 1991]. The discharge of too high nitrogen concentrations into the receiving waters without proper treatment would lead to eutrophication, i.e. algal outbreaks and/or fish death in rivers, lakes and coastal areas (Cheremisinoff 1996; Metcalf and Eddy 1991; Mussati et al. 2002; UNEP 1991). The predominant N fraction in tannery wastewaters is organic N emanating from the breakdown of nitrogenous compounds from processed hides and skin. Ammonia nitrogen is extremely toxic to fish and other aquatic life at high concentrations (UNEP 1991).
In Ethiopia, there are more than 20 tanning industries, concentrated mainly in the southern central part of the country. More than 90% of the existing tanning industries in Ethiopia do not have any type of wastewater treatment and discharge their wastewaters directly into nearby water bodies and open land. Recently, however, effluent regulations and guidelines have been developed as part of the Environmental Policy of the Federal Government of Ethiopia (GOE 2002). Consequently, discharge standards for nitrogen necessitate treatment of wastewaters from chrome tanneries that are mainly undertaken through processes of nitrification and denitrification.
Biological denitrification is the dissimilatory reduction of nitrate to nitrogen gas by heterotrophic bacteria using nitrate as an electron acceptor and degradable organic matter as carbon source under anoxic conditions. Nitrification is the biological oxidation of ammonia to nitrite/nitrate by two specialised groups of autotrophic bacteria that takes place under aerobic conditions (Focht and Verstraete 1977; Kuenen and Robertson 1988).
Different biological systems comprising different configurations have been used to efficiently remove carbon and nitrogen pollution from combined municipal and industrial wastewater types (Banas et al. 1999; Borzacconi et al. 1999; Collivignarelli and Bertanza 1999; Gupta and Sharma 1996; Henze 1991; Pochana and Keller 1999; Stevens et al. 1999). Little is known, however, about their effectiveness to treat wastewaters generated by tanning industries. A high content of organic compounds together with the presence of biological inhibitors such as chromium and sulphide in tannery wastewaters affect the treatment processes (Weimann et al. 1998; Wiegant et al. 1999).
The specific objectives of this study were, therefore, (1) to set-up a pilot plant for predenitrification and nitrification processes and test its effectiveness for simultaneous nitrogen and organic matter removal from tannery wastewaters, and (2) to evaluate the nitrification and denitrification potential of the pilot plant using nitrate uptake rate (NUR), and ammonia uptake rate (AUR) in the presence of different concentrations of chromium III as toxicant. In this study, evaluation of the treatment efficiencies and process performances of the pilot plant during the 6 months of plant operation are presented.
Materials and methods
Experimental set-up
The predenitrification-nitrification pilot process plant was developed and installed on the premises of the Department of Biology, Addis Ababa University, and its performance was monitored for 6 months from March to August 2002. Figure 1 shows the design of the pilot plant, which consists of an influent feeding tank, a denitrification reactor of 100 l, equipped with a vertical stirrer (RGL 2, Heidolph, Kelheim, Germany), an aerobic activated sludge unit of 200 l and a 50 l sedimentation (clarifier) tank. The process was adjusted in such a way that influent wastewater was pumped from the influent collection tank into a denitrification tank at a flow rate of 5 l h−1 using a peristaltic pump (PD 5206, Heidolph) to maintain the nominal hydraulic residence time (HRT) of the anoxic and aerobic reactors at 20 h and 40 h, respectively. The mixed liquor from the activated sludge, and sludge from the effluent were recycled to the head of the denitrification reactor at rates of 100–200% and 100–150% by a peristaltic pump and airlift pump, respectively (Table 1).
Process start-up
The denitrification and the aerobic reactors were initially seeded with a mixture (90:10) of acclimatised waste from the Ethiopia tannery wastewater treatment plant and from the Kaliti municipal waste treatment pond system. This was used as a starter inoculum of microorganisms and substrate to activate the process. The system was subsequently fed with combined raw tannery wastewaters (soaking, pickling and tanning processes) that were sampled from the Modjo Tannery, 70 km away from the pilot treatment site installed at the University.
The process performances consisted of four feeds of 30, 28, 26 and 33 days, respectively, over the study period. The operational parameters of the pilot plant for the four experimental feeds and average values of mixed liquor suspended solids (MLSS), mixed liquor volatile suspended solids (MLVSS), sludge volume index (SVI) and dissolved oxygen (DO) are given in Table 1. After 1 year of the process performance, experiments were carried out with increased organic loading rates from 1.1 kg chemical oxygen demand (COD) m−3 day−1 to 2.0 kg COD m−3 day−1 in order to evaluate whether the system could possibly withstand high loading perturbations over time.
NUR and AUR measurements
In order to determine the denitrification and nitrification capacity of the system, NUR and AUR batch experiments of the sludge were determined according to Kristensen et al. (1992). Two 2-l capacity plastic lab scale reactors (R1 and R2) were used for the NUR and AUR batch experiments where 1.5 l activated sludge sampled from the pilot plant was added to each reactor. Tannery wastewater was added to R1 as an internal carbon source whereas acetate was added to R2 as an external source of carbon, which was used as a reference. All experiments were conducted four times. The NUR experiments were carried out under anoxic conditions by flushing the reactors with N2 gas.
The effect of chromium on AUR
The inhibitory effect of chromium on the nitrification activities of sludge from the pilot plant was determined by AUR experiments in four batches where chromium III was added at concentrations of 50, 85, 170, 255 mg l−1, respectively. The other reactor (R1) without the addition of chromium was used as a reference for calculating the actual nitrification rate of the sludge. Toxicity was quantified in terms of the reduction in AUR of the microorganisms in the activated sludge versus that in the control. In all cases, AUR (nitrification rate) and NUR (denitrification rate) were calculated as a change of ammonia nitrogen [mg NH4-N g volatile suspended solids (VSS)−1 h−1] and nitrate nitrogen (mg NO3-N g VSS−1 h−1), respectively. In both the NUR and AUR experiments, samples were taken after 1, 15, 30, 60, 120 and 180 min and filtered immediately through Whatman filter paper.
Analyses
The raw tannery wastewater samples were analysed twice a month for a number of parameters to assess its biological treatability. The analyses of the combined raw wastewaters were made on composite samples immediately after sampling. COD, total N, PO43−, NH4-N, NO3-N, NO2-N, S2− and SO42− were all measured calorimetrically using a spectrophotometer (DR/2010, Hach, Loveland, Co.) according to Hach instructions. Standard methods (APHA 1992) were used to determine BOD5, suspended solids (SS) and VSS. Total chromium (Tot-Cr) was determined using Buck Scientific Model 210 VGP atomic absorption according to standard methods (APHA 1992). Total dissolved solids (TDS), conductivity and salinity of the wastewater samples were measured using a conductivity meter (CO 150, Hach). DO was regularly monitored using a DO probe (YSI 5905, Yellow Springs Instruments, Yellow Springs, Ohio). Temperature and pH were also measured on a routine basis throughout the experimental period. SVI was determined using a 1-l measuring cylinder over 30 min of settling time.
Results
Average influent and effluent characteristics of the wastewaters for the four experimental feeds are given in Table 2. The influents were characterised by high alkalinity content with a resulting pH value of above 10.0 due to the chemicals used in leather processing. Influent total N and COD concentrations ranged from 927.0 mg l−1 to 2,140.0 mg l−1 and 9,583.0 mg l−1 to 13,515.0 mg l−1, respectively, whereas influent ammonium N varied from 149.0 mg l−1 to 178.0 mg l−1. Sulphide and Tot-Cr concentrations were in the range of 466.3–794.0 mg l−1 and 23.3–42.5 mg l−1, respectively, during the process feeding stages. The data in Table 2 show that tannery effluents are rich in nitrogen, especially organic nitrogen, but very poor in phosphorus.
As can be seen from Table 2, high values of effluent COD, BOD5, total nitrogen and ammonium nitrogen were recorded for the first two experimental feed phases, but were substantially decreased in the last two successive feeds. The effluent total N and ammonium N concentrations varied between 17.5–166.7 mg l−1 and 8.4–86.0 mg l−1, respectively, whereas the NO3-N concentrations ranged from 2.9 mg l−1 to 4.4 mg l−1. Similarly, effluent COD and BOD5 concentrations were in the range of 157.0–527.2 mg l−1 and 26.7–127.3 mg l−1, respectively. The effluent pH was also observed to decrease in successive feeds and was between 7.6 and 7.8. There was a substantial decrease in Tot-Cr (0.2–4.2 mg l−1) and sulphide (0.7–18.0 mg l−1).
The pilot plant treatment efficiencies for the four experimental feeds are reported in Table 3. The pilot plant showed high total N and COD removal efficiencies of 98% in the last experimental feed. The removal rates varied between 82–98% for total N and 95–98% for COD. The higher COD removal in the pilot plant processes was associated with higher denitrification efficiency. BOD5, S2− and Tot-Cr were almost completely removed during all runs (Table 3).
The average ammonium N removal rate was progressively increased from 50% for the first and second feeds to 95% removal efficiency in the fourth experimental feed phase. The latter was achieved at an organic loading rate of 0.72 kg COD m−3 day−1 (Fig. 2) and the corresponding average effluent ammonium nitrogen concentration was 8.4 mg l−1. At high organic loading rate, ammonium nitrogen removal rate was low and effluent ammonium N concentrations were high (>60 mg l−1). To evaluate whether the system could possibly withstand higher loading perturbations over time, an experiment was performed at increased COD loading rates of 1.1–2.0 kg COD m−3 day−1 after a year of the study period. At a COD loading rate of 1.5 kg COD m−3 day−1, ammonium nitrogen removal rate was 70%. As the organic loading rate increased to 2.0 kg COD m−3 day−1, the corresponding ammonium nitrogen removal rate was only 40%. Similarly, an inverse relationship was also noted between DO and ammonium nitrogen concentrations in the system (Fig. 3). The measured DO concentrations of the oxic reactor varied from 1.2 mg O2 l−1 to 6.0 mg O2 l−1 during the experimental period.
NUR measurements
The denitrifying capacity of the biological processes of the pilot plant determined by NUR experiments is shown in Fig. 4. NUR experiments gave results ranging from 6.5 mg NO3-N g VSS−1 h−1 to 9.3 mg NO3-N g VSS−1 h−1 for the test wastewater used as an internal carbon source and 5.7–7.7 mg NO3-N g VSS−1 h−1 when acetate was used as external carbon source. The average denitrification rate with internal carbon source was found to be 8.0 mg NO3-N g VSS−1 h−1, which indicates the denitrification potential of the pilot plant. The NUR test with the addition of acetate as external carbon source showed an average denitrification rate of 6.7 mg NO3-N g VSS−1 h−1.
The effect of chromium on AUR
The values of nitrification rates measured with the AUR experiments ranged from 4.3 mg NH4-N g VSS−1 h−1 to 6.4 mg NH4-N g VSS−1 h−1. The highest nitrification rates were reported in runs III and IV, indicating the efficiencies of the sludge of the pilot plant. Figure 5 shows the effect of chromium III on ammonium uptake rate using activated sludge from the pilot plant. The nitrification rate slowly decreased over the four runs for the different chromium III concentrations. At chromium concentration of 50 mg l−1, the AUR was almost similar to the reference test. The toxic effects of chromium III increased with increasing concentrations of the metal, and 50% inhibition was observed at a concentration of around 85 mg l−1.
Discussion
This study examined the characteristics of tannery wastewaters and its biological treatability in pilot plant operations, the activity of the sludge and the effects of toxicants on process efficiency. The process plant showed increased total N removal efficiencies of 82, 94, 95 and 98%, respectively, for the four experimental feeds despite of high chromium and sulphide content in the influent. A similar removal efficiency of 90–98% was reported (Hirata et al. 2001) from industrial wastewaters discharged from metal recovery processes. High COD removal efficiencies (95–98%) were also registered for the pilot process plant throughout the experimental feed phases.
From the sludge activity of the process plant (Fig. 4), it was observed that denitrification rates using influent tannery wastewater as internal carbon source were 20–40% higher compared with values obtained with acetate as an added carbon source. The higher denitrification rate with tannery wastewater as internal carbon source indicates that tannery wastewaters contain a broad range of easily biodegradable organic matter that can maintain active denitrifying consortia in the system, thus ensuring complete denitrification capacity of the system without the need for any external carbon source. Earlier reports (Kajuwa and Klapwijk 2000; Kristensen et al. 1992) show that NUR has effectively been used to determine the denitrification capability of a treatment system.
An interesting point to note was that ammonium N removal efficiencies of 46–68% for the first three consecutive feeds increased significantly to 95% in the fourth feed. These results were consistent with the results of laboratory measurements of potential AUR. It was reported (Hirata et al. 2001) that ammonia removal efficiency of 80% to 92% was achieved as a result of acclimatising microorganisms with increasing residence time. Longer retention times retain abundant nitrifiers in the process.
The nitrification rates of the pilot plant in the beginning probably suffered due to an insufficient acclimatisation period for the nitrifying consortia to stabilise themselves in the system. This period was perhaps in turn affected by organic loading rates; low DO levels and system malfunction resulting from foaming of the wastewater. At the beginning of the experiment, excessive foam formation in the aerated tank caused by influent surfactants resulted in the escape of activated sludge solids to the effluent, which increased the sludge-wasting rate, thereby a decrease in sludge residence time. Similarly, when the DO level in the reactor was less than 2.0 mg l−1, the nitrification rate was only 25% and ammonium oxidation increased with increasing DO concentrations in the system (Fig. 3). Numerous reports (Cao et al. 2002; Dangcong et al. 2001; Philips et al. 2002) have indicated that in order to ensure that DO is not a limiting factor in the nitrification reaction, a level not less than 2.0 mg l−1 must be maintained.
Variations in organic loading rate to the process plant were also observed to have an effect on ammonium nitrogen removal rate. At a COD loading rate of more than 1 kg COD m−3 day−1, ammonium nitrogen removal rate was only around 50–60% (Fig. 2). Higher organic loads accelerate the heterotrophic growth rate and the sludge quantities produced in the system, thus decreasing the fraction of nitrifiers present in an activated sludge system (Gupta and Gupta 2001; Jokela et al. 2002; Kristensen et al. 1992). Thus, the contribution of autotrophic nitrification would be minimal.
The system was also fed with increased organic loading rates from 1.1 kg COD m−3 day−1 to 2.0 kg COD m−3 day−1 to evaluate whether it could possibly withstand higher loading perturbations over time after 1 year of plant operations. At a COD loading rate of 1.5 kg COD m−3 day−1, the ammonium nitrogen removal rate was 70%. As the organic loading rate increased to 2.0 kg COD m−3 day−1, the corresponding ammonium removal rate was only 40%. The result showed that ammonia oxidation increased slightly, by 10%, when COD loading rate was increased from the original loading rate by 50%. When the loading rate increased by 100%, the removal rate again decreased, verifying that high organic loading to the aeration tank affected the nitrification efficiency of the system.
Nitrification, like most bacterial processes, is affected by pH and temperature conditions. Different studies indicate the applicable range of pH and temperature to be about 6.5–8.6 and 5–30°C, respectively (Cheremisinoff 1996; Cloete and Muyima 1997; Grunditz et al. 1998; Im et al. 2001). In this study, the pH and the temperature of the nitrification reactor varied from 7.5 to 8.2 and 15°C to 20°C, respectively, indicating that these parameters were within the normal range of operating conditions in the system.
AUR investigations with different concentrations of chromium III showed 50% inhibition at a concentration of around 85 mg l−1. Chromium III is readily precipitated from solution between pH 6 and 10 (Basu et al. 1997; Kotás and Stasicka 2000), thus making it less available to biological systems in high pH sludge. Consequently, the concentration of this metal in the activated sludge solution is very low, and may not affect the nitrifying population in the reactor. AUR experiments in the presence of chromium III showed that this metal did not cause process inhibition during pilot plant operations. It has been demonstrated that information from AUR can be used to identify possible inhibitory effects from wastewaters (Kristensen et al. 1992; Madoni et al. 1999).
The SVI and SS in the activated sludge were in the range of 38–65 ml g−1 MLSS and 2.0–4.0 g l−1, respectively, in the completely aerated tank during pilot plant performances (Table 1). These parameters demonstrate the well-settling properties of the activated sludge and good formation of activated sludge biomass. It is also reported that well-settling activated sludge is characterized by an SVI value of less than 100 ml g−1, whereas sludge with bulking problems usually has an SVI value greater than 200 ml g−1 (Cloete and Muyima 1997; Gupta and Sharma 1996).
It was interesting to note that the effluent values achieved towards the fourth experimental feeds of the pilot plant (Table 2) were in agreement with the permissible limits for discharge of tannery effluents into surface water bodies adopted in many countries compiled by the United Nations Industrial Organization (UNIDO) (Bosnic et al. 2000).
From the overall results of the process plant, it can thus be concluded that the predenitrification-nitrification process has high nitrogen removal capability. This process favoured the growth of high consortia of denitrifiers in the anoxic tank and encouraged the consortia to attain high denitrification efficiency; it also improved the overall nitrification efficiency of the system. More than 90% COD and 95% S2− were removed in the predenitrification anoxic basin. Chromium III, the heavy metal available in tannery wastewater, was likely precipitated or adsorbed by the sludge in the first stage, thus causing no harm to the nitrifying populations. Therefore, it was found to be operationally efficient for simultaneous removal of nitrogen, organic substrates and other pollutants from tannery wastewaters.
References
APHA (1992) Standard methods for the examination of water and wastewater, 18th edn. American Public Health Association, Washington D.C.
Banas J, Plaza E, Styka W, Trela J (1999) SBR technology used for advanced combined municipal and tannery wastewater treatment with high receiving water standards. Water Sci Technol 40:451–458
Basu M, Bhattacharya S, Paul AK (1997) Isolation and characterization of chromium resistant bacteria from tannery effluents. Bull Environ Contam Toxicol 58:535–542
Borzacconi L, Ottonello G, Castello E, Pelaez H, Gazzola A, Vinas M (1999) Denitrification in a carbon and nitrogen removal system for leachate treatment: performance of an up flow sludge blanket (USB) reactor. Water Sci Technol 40:145–151
Bosnic M, Buljan J, Daneils RP (2000) Pollutants in tannery effluents: limits for discharge into water bodies and sewers. Regional program for pollution control in the tanning industry in South East Asia, US/RAS/92/120. United Nations Industrial Development Organization, Vienna, pp 17–26
Cao GM, Zhao QZ, Sun XB, Zhang T (2002) Characterization of nitrifying and denitrifying bacteria co-immobilized in PVA and kinetics of model of biological nitrogen removal by co-immobilized cells. Enzyme Microb Technol 30:49–55
Cheremisinoff NP (1996) Biotechnology for waste and wastewater treatment. Noyes, Park Ridge, N.J.
Cloete TE, Muyima NYO (eds) (1997) Microbial community analysis: the key to the design of biological wastewater treatment systems. IAWQ No. 5, London
Collivignarelli C, Bertanza G (1999) Simultaneous nitrification–denitrification processes in activated sludge plants: performance and applicability. Water Sci Technol 40:187–194
Dangcong P, Bernet N, Delgenes JP, Moletta R (2001) Simultaneous organic carbon and nitrogen removal in an SBR controlled at low dissolved oxygen concentration. J Chem Technol Biotechnol 76:553–558. DOI 10.1002/jctb.419
Focht DD, Verstraete W (1977) Biochemical ecology of nitrification and denitrification. Adv Microb Ecol 1:135–214
Government of Ethiopia (GOE) (2002) Environmental pollution control proclamation. Nagarit Gazeta of the Federal Democratic Republic of Ethiopia, Proclamation No. 300/2002, Addis Ababa
Grunditz C, Gumaelius L, Dalhammar G (1998) Comparison of inhibition assays using nitrogen-removing bacteria: application to industrial wastewaters. Water Res 32:2995–3000
Gupta AB, Gupta SK (2001) Simultaneous carbon and nitrogen removal from high strength domestic wastewater in an aerobic RBC biofilm. Water Res 7:1714–1722
Gupta SK, Sharma R (1996) Biological oxidation of high strength nitrogenous wastewater. Water Res 30:593–600
Henze M (1991) Capabilities of biological nitrogen removal processes from wastewater. Water Sci Technol 23:669–679
Hirata A, Nakamura Y, Tsuneda S (2001) Biological nitrogen removal from industrial wastewater discharged from metal recovery processes. Water Sci Technol 44:171–180
Im JH, Woo HJ, Choi MW, Hi KB, Kim CW (2001) Simultaneous organic and nitrogen removal from municipal landfill leachate using an anerobic–aerobic system. Water Res 35:2403–2410
Jokela JPY, Kettunen RH, Sormunen KM, Rintala JA (2002) Biological nitrogen removal from municipal landfill leachate: low-cost nitrification in biofilters and laboratory scale in-situ denitrification. Water Res 36:4079–4087
Kajuwa K, Klapwijk B (2000) A method to estimate denitrification potential for predenitrification systems using NUR batch test. Water Res 33:2291–2300
Kotás J, Stasicka Z (2000) Chromium occurrence in the environment and methods of its speciation. Environ Pollut 107:263–283
Kristensen HG, Jorgensen PE, Henze M (1992) Characterisation of functional microorganism groups and substrate in activated sludge and wastewater by AUR, NUR and OUR. Water Sci Technol 25:43–57
Kuenen JG, Robertson LA (1988) Ecology of nitrification and denitrification. In: Cole JA, Ferguson SJ (eds) The nitrogen and sulfur cycles. Cambridge University Press, Cambridge, pp 161–218
Madoni P, Davoli D, Guglielmi L (1999) Response of sOUR and AUR to heavy metal contamination in activated sludge. Water Res 33:2459–2464
Metcalf and Eddy (1991) Wastewater engineering: treatment, disposal and reuse. McGraw-Hill, New York, pp 359–434
Mussati M, Gernaey K, Gani R, Jørgensen SB (2002) Performance analysis of a denitrifying wastewater treatment plant. Clean Technol Environ Policy 4:171–182. DOI 10.1007/s10098-002-0157-8
Philips S, Wyffels S, Sprengers R, Verstraete W (2002) Oxygen-limited autotrophic nitrification/denitrification by ammonia oxidisers enables upward motion towards more favourable conditions. Appl Microbiol Biotechnol 59:557–566. DOI 10.1007/s00253-002-1059-8
Pochana K, Keller J (1999) Study factors affecting simultaneous nitrification and denitrification (SND). Water Sci Technol 39:61–68
Stevens GM, Barnard JL, Rabinowitz B (1999) Optimization of nutrient removal in anoxic zones. Water Sci Technol 39:113–118
UNEP (1991) Tannery and the environment: a technical guide to reducing the environmental impact of tanning operations. UNEP/IEO, UN Publication series III, Paris
Weimann M, Schenk H, Hegemann W (1998) Anaerobic treatment of tannery wastewater with simultaneous sulphide elimination. Water Res 32:774–780
Wiegant WM, Kalker TJJ, Sontakke VN, Zwaag RR (1999) Full scale experience with tannery water management: an integrated approach. Water Sci Technol 39:169–176
Acknowledgements
This work was supported by the Swedish International Development Cooperation Agency (Sida/SAREC) through the East African Regional Programme and Research Network for Biotechnology, Biosafety and Biotechnology Policy Development (BIOEARN). The excellent technical and material support of Mr. Zeleke Teferi at the Addis Ababa Water and Sewerage Authority is highly acknowledged. Modjo and Ethiopia Tanneries deserve special thanks for their consistent support and permission to use the tannery wastewaters for this study.
Author information
Authors and Affiliations
Corresponding author
Rights and permissions
About this article
Cite this article
Leta, S., Assefa, F., Gumaelius, L. et al. Biological nitrogen and organic matter removal from tannery wastewater in pilot plant operations in Ethiopia. Appl Microbiol Biotechnol 66, 333–339 (2004). https://doi.org/10.1007/s00253-004-1715-2
Received:
Accepted:
Published:
Issue Date:
DOI: https://doi.org/10.1007/s00253-004-1715-2