Abstract
We used Lagrangian numerical simulations to examine the trans-Pacific dispersal processes of loggerhead turtle hatchlings. Ten thousand simulated particles were released from each of the three nesting regions in Japan and tracked for 5 years. Results showed many particles moving eastward, drifting in the Kuroshio Current followed by the Kuroshio Extension Current. However, no particles reached Baja California, a known feeding area, through passive processes, indicating that trans-Pacific transportation requires active swimming by turtles. The duration of the trans-Pacific dispersal was estimated to be at least 1.6–3.4 years, with some turtles drifting in the Kuroshio Countercurrent and remaining in the western Pacific even after 5 years. This indicates that as revealed by previous genetic studies, not all loggerheads always disperse along a trans-Pacific route. The findings showed that survival and expected growth rates varied widely according to ambient temperatures during drifting, which in turn depended on nesting location.
Similar content being viewed by others
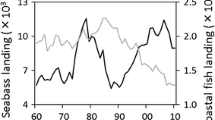
Avoid common mistakes on your manuscript.
Introduction
Sea turtles are known to make long-distance migrations at various stages in their life history. For example, adult female turtles travel hundreds or thousands of kilometers from nesting to objective foraging sites using active swimming (Luschi et al. 2003), while hatchling sea turtles are believed to disperse over long distances by drifting passively in ocean currents from the beach where they emerge to coastal areas where they forage during a period termed the lost year (Carr and Meylan 1980; Carr 1987; Hamner 1988; Musick and Limpus 1997). This period remains the least understood stage in sea turtle life history (Witherington 2002).
The loggerhead sea turtle (Caretta caretta) is widely distributed in temperate, subtropical, and tropical waters (Dodd 1988). In the North Pacific, nesting concentrations of loggerhead turtles occur in Japan (Nishimura 1967; Uchida and Nishiwaki 1982), but are notably absent from the central and eastern Pacific, where large numbers of juveniles have been observed (e.g., Pitman 1990). From studies of size distribution, the smallest loggerhead turtles observed in the central North Pacific and in coastal areas off Baja California are known to be 12.3 cm (Zug et al. 1995; Parker et al. 2005) and 26.6 cm in straight carapace length (SCL), respectively (Pitman 1990; Gardner and Nichols 2001; Seminoff et al. 2004; Koch et al. 2006; Peckham et al. 2008), with respective ages estimated at approximately 1.0 and 4.2 years (Chaloupka 1998). Previous studies using molecular genetic analyses have concluded that the juvenile loggerhead turtles found in the North Pacific Current and around Baja California are primarily of Japanese origin (Bowen et al. 1995; Hatase et al. 2002a), indicating that hatchling turtles undergo trans-Pacific dispersal from the Japanese beaches where they emerge to East Pacific feeding areas. However, the dispersal routes used by hatchlings, the actual duration of trans-Pacific dispersal in the North Pacific Ocean, and variations in these factors are still not known as well as the dispersal regimes of other species of sea turtle or regions.
Polovina et al. (2006) released loggerheads reared for 1–3 years off the coast of Japan and then tracked them with an Argos-linked satellite. The results of that study showed that some released turtles were able to migrate through the Kuroshio Current, followed by the Kuroshio Extension (defined by Sverdrup et al. 1942). However, these turtles had already grown to sizes ranging from 25.6 to 64.8 cm SCL, and hence the results do not seem to reflect hatchling behavior, although Japanese hatchlings probably make use of the Kuroshio Current followed by the Kuroshio Extension.
Numerical simulation modeling of hatchling dispersal is a useful tool for improving understanding of the general processes that may affect dispersal processes. A numerical model of North Atlantic general circulation has been applied to loggerhead turtles to clarify the trajectory of their trans-Atlantic drift (Hays and Marsh 1997). Although in numerical simulations of hatchling dispersal, constraints may occur due to the simplification of biological and ecological aspects of the model, for example, that the turtles never swim and that the diffusion speed of turtles does not change over the simulation period, this kind of study has been recently popularized to determine hatchling dispersal in the ocean (e.g., Hays et al. 2010; Putman et al. 2010a). Additionally, due to rapid improvements in computer technology, high-resolution, global-scale numerical modeling has been developed and applied to a few fish species to clarify larval transport mechanisms in the open ocean (Kim et al. 2007; Zenimoto et al. 2009; Kitagawa et al. 2010). Such large-scale, high-performance simulations are essential to examine the transocean transport of hatchlings to better understand their ecology.
Thus, the objectives of the present study were to investigate the transport processes of loggerhead sea turtle hatchlings in the North Pacific using numerical simulations and sea surface temperature (SST) data analysis, to determine their trans-Pacific drift time, and to examine differences in transport and dispersal processes between nesting beaches.
Materials and methods
Circulation model
Our Lagrangian study of loggerhead turtle hatchling transport was based on a high-resolution circulation field model developed by the Japan Agency for Marine–Earth Science and Technology (Masumoto et al. 2004). The high-resolution ocean model for the Earth simulator (hereafter referred to as OFES) is based on the Modular Ocean Model, and the model domain covers a near-global region extending from 75°S to 75°N except for the Arctic Ocean, with a horizontal grid spacing of 1/10°. The model has 54 vertical levels, with the distance among levels varying from 5 m at the surface to 330 m at the maximum depth of 6,065 m. The model topography is constructed from the 1/30 bathymetry dataset created by the Ocean Circulation and Climate Advanced Model project at the Southampton Oceanography Centre. Monthly mean wind stress, averaged from 1999 to 2003 from the NCEP/NCAR reanalysis data (NOAA National Meteorological Center), was used for climatological seasonal integration. Detailed descriptions of the basic settings of the model are given by Masumoto et al. (2004), Kim et al. (2007), and Sasaki et al. (2008).
Particle tracking
To estimate the amount of hatchling transport to the eastern Pacific, simulated particles were released close to three major nesting beaches around Japan and tracked for 5 years (1,800 days) as passive tracers. Loggerhead nesting mainly occurs between late May and August (Uchida and Nishiwaki 1982), and the incubation period of the eggs ranges between 46 and 82 days, depending on temperature (Matsuzawa et al. 2002). For simplification, particles were released on 1 August 1999. The start positions of the numerical simulation were set at three different sites close to loggerhead nesting regions: off Okinawa (26°N, 128°E), on the east side of the Kuroshio (i.e., inside the North Pacific Subtropical gyre), off Yakushima (30°N, 130°E), close to the Kuroshio, and off Wakayama (33°N, 135°E), on the west side of the Kuroshio (i.e., outside the gyre). Ten thousand particles were released from each of these positions (Fig. 1). Transport processes were simulated for 1999–2003 using the velocity fields of the OFES. For this simulation, we made two fundamental assumptions. The first was that the turtles never swim actively, only undertaking passive drifting, as neonate loggerhead turtles inhabiting oceanic fronts have been found to be largely inactive and to be closely associated with floating material (Witherington 2002). The second was that the diffusion speed of turtles never changes with their growth throughout the simulation period, since no data are available on turtle drift speed in the open ocean, which changes with growth.
In this study, particles entering areas where the SST was below 4.5°C, which is lethal for this species (Schwartz 1978), and landed particles were considered to have suffered mortality. This model did not consider the predation mortality of turtles due to a lack of data on open ocean predation. The survival rate was defined as the total particle number minus the number of particles suffering mortality.
To examine the distribution of drifting particles, the North Pacific (120°E–120°W) was divided into six areas (areas I–VI; Fig. 1). In addition, to investigate differences in the drift velocity of particles between these six areas, the velocity per year of all surviving particles remaining within each area was calculated. We then pooled the 5 years of data and calculated the mean annual velocity of particles in each area.
Analysis of SST
To examine the ambient temperatures experienced by hatchlings during 5 years of transport from August 1 (Fig. 1), SST data on a 1.0° mesh were downloaded from the NOAA Optimum Interpolation (OI) SST V2 Web site (http://www.esrl.noaa.gov/psd/data/gridded/data.noaa.oisst.v2.html). The OI SST analysis is produced weekly on a 1.0° grid. The analysis uses in situ and satellite SSTs plus SSTs simulated by sea ice cover. The NOAA OI.v2 SST monthly fields are derived by linearly interpolating the weekly OI version 2 fields to daily fields, then averaging the daily values over 1 month. Monthly fields are presented in the same format and spatial resolution as weekly fields. Precise information is shown on the Web site (http://www.emc.ncep.noaa.gov/research/cmb/sst_analysis/).
To examine the ambient temperatures experienced by turtles during dispersal, particles were divided into four groups in an east–west direction based on their distributions 1 year (360 days) after release: east, central-east, central-west, and west. Then, mean daily ambient temperatures were calculated for each group. The ambient temperature was shown as a running mean for 30 days, as the SST data are monthly.
Results
Almost all of the particles released off Yakushima (close to the Kuroshio) were directly transported by the Kuroshio Current followed by the Kuroshio Extension to waters east of 140°E and north of 35°N in less than 30 days after release (Fig. 2a). Then, the particles were all transported eastward in waters between 35°N and 40°N. The survival rate decreased to about 80.7% from 120 to 240 days after release (Fig. 3) because of low ambient temperatures during the winter. A year after being released off Yakushima, 4,264 particles were past the date line (Table 1). In the second year, 6,833 particles were transported to the east of the date line, but were around areas IV and V (180°–140°W) at their farthest extent. After 5 years, the particles had reached a maximum of around 124°W, but no particles had reached the coastal areas of Baja California (waters of 120°–110°W), which is one of the known foraging areas of the species. The survival rate slightly decreased to 78.3% during the period from 1 to 5 years after release.
Tracks from 0 to 180 days after release from the three nesting sites. The location of particles is plotted in the upper layer as days elapsed. Thus, the tracks in the earlier period are overlaid if the particles remain in the same place, showing how the particles were transported by the Kuroshio Current and the Kuroshio Countercurrent, and generated in the cyclonic eddy
Particles released off Okinawa (east side of the Kuroshio) drifted around Okinawa Island before entering the Kuroshio, and some of them were carried southward (Fig. 2b). On entering the Kuroshio, most particles were transported by the Kuroshio to the waters east of 140°N (Fig. 2b). The distribution of the particles 1 year after release was roughly divided into two groups: a group that was transported eastward (Areas II–V, 7,313 particles; Table 1), with 2,704 of these particles crossing the date line, and a group that drifted in the Kuroshio Countercurrent or anticyclonic eddies in the western Pacific (Area I, 971 particles; Table 1). After the second year, the particles of the former group were dispersed in a similar way to the particles released off Yakushima. The latter group drifted in the subtropical gyre in the western Pacific. The survival rate decreased to less than 80% in the first year, before gradually decreasing to 76.2% in the fifth year (Table 1).
Many of the particles released off Wakayama (west side of the Kuroshio) landed within about 10 days of their release, causing the survival rate to rapidly decrease to below 20% by 90 days and to 15.5% 150 days after release (Fig. 3). On entering the Kuroshio, most particles were entrained into and drifted in the cyclonic eddy generated around the Kuroshio frontal area (Fig. 2c). Few particles were transported to the east, and only 40 particles had crossed the date line 1 year after their release (Fig. 4). The survival rate was maintained at the same level after 150 days (Fig. 3).
When viewed regionally, the mean annual velocity of particles was found to decrease as the particles drifted eastward (Fig. 5). Differences were observed in the distribution of drifted particles each year among the three release areas (Table 1).
Figure 6 shows the mean ambient temperatures for particles in the east and west groups during the initial 2 years after their release. Ambient temperatures for the particles in the central-east and central-west groups were generally between those of the east and west groups. As a whole, the ambient temperatures fluctuated seasonally. For the particles released off Yakushima, the ambient water temperatures for the east group reached almost 13 ± 3.8°C around 200 days (wintertime) after release (Fig. 6). Little difference was observed between the east and west groups. In contrast, for the particles released off Okinawa, the lowest temperatures for the east and west groups were still above 20 and 16°C around 200 days, respectively, much higher than the ambient temperatures experienced by the Yakushima particles. For the particles released off Wakayama, the lowest temperatures for the east and west groups were around 16 and 18°C, respectively.
Discussion
Since tracking coin-sized hatchlings under natural conditions over long periods is still impossible (Luschi et al. 2003), ontogenetic changes in their distribution and growth rates remain largely unknown, and thus knowledge on the ecology of hatchlings is constructed from field observations (e.g., Carr 1987; Witherington 2002) and genetic studies (e.g., Bowen et al. 1995) on a piecemeal basis. Therefore, although the present simulation model was based in part on limited knowledge of turtle behavior and diffusion processes, the present study contributes greatly to the improved understanding of the ecology of loggerhead turtle hatchlings in the North Pacific Ocean, showing the transport and dispersal process, ambient temperatures, survival rates of loggerhead turtle hatchlings (simulated particles), and the wide variations in these factors among nesting locations (starting points of the simulation). However, future studies on the simulation including the concepts of predation mortality and hatchling behavior in the open ocean are needed to more understand the ecology of hatchlings.
To our knowledge, this is the first study to investigate the transport of sea turtle hatchlings in the Pacific Ocean using Lagrangian numerical simulations on a basin scale (about 11,000 km) over 5 years. The present study contains higher resolution than previous simulation studies (Hays and Marsh 1997; Hays et al. 2010) and covers a larger scale than studies from the Mediterranean (Hays et al. 2010) and the Gulf of Mexico (Putman et al. 2010a). This simulation model could be applied to other species of sea turtles and other marine taxa, as well as to other regions. Therefore, the further application of this model would contribute to improving the understanding of the ecologies of marine taxa. Satellite-tracked drifter buoys provide another method to infer hatchling drift scenarios. As particle tracking models have a finite resolution (in our case 1/10°), they cannot capture the full dynamics of ocean currents and hence, directly tracked buoys provide a good alternative method to assess drift patterns (Monzón-Argüello et al. 2010).
The drift velocity of particles in the westernmost part of the Pacific Ocean (area I) was considerably faster than those in other areas (II–VI) (Fig. 5). In fact, about 48% of the surviving particles released off Yakushima were transported more than 4,500 km to the area east of the date line in the first year (Table 1). This transportation period to the date line coincides with the 1.0 years estimated age of the smallest turtle captured around the date line (Zug et al. 1995; Parker et al. 2005), according to a growth model for juvenile loggerheads in the North Pacific (Chaloupka 1998). Furthermore, in the second year, 75% of hatchlings crossed the date line (Table 1). This rapid transportation from the west would be accomplished by the Kuroshio Current, followed by the Kuroshio Extension. Thus, these currents play an important role in transporting loggerhead hatchlings from nesting beaches for trans-Pacific dispersal. The drift velocity of particles decreased with eastward movement (Fig. 5). For example, although 38% of particles reached waters from 160° to 140°W in the second year, most of these particles remained within waters between 140° and 130° even in the fifth year because the velocity of ocean currents is much lower in waters east of the date line compared to those of the Kuroshio or the Kuroshio Extension. Generally, the volume transport of the Kuroshio is 65 Sv, while that of the North Pacific Current around 150°W is only 5 Sv (Sverdrup et al. 1942). Furthermore, the North Pacific Current splits into the southward California Current and the northward Alaska Current. Consequently, in our simulation, no particles reached the coastal area of Baja California. This suggests that the trans-Pacific dispersal of loggerhead turtles to Baja could not be achieved only through passive processes (drift), and indicates that turtles must swim actively to reach the waters off Baja California, and even more so to reach the Gulf of California.
Previous studies have recorded a minimum size record of 26.6 cm SCL for a loggerhead turtle seen off Baja California (Pitman 1990; Gardner and Nichols 2001; Seminoff et al. 2004; Koch et al. 2006; Peckham et al. 2008). A turtle of this size is estimated to be 4.2 years old according to a growth model (Chaloupka 1998). Neonate loggerhead turtles inhabiting oceanic fronts have been found to be largely inactive and to be closely associated with floating material (Witherington 2002). In addition, post-hatchling loggerhead turtles did not swim with forelimb stroking, instead employing energy-saving swimming with a hindlimb kick for 95% of their swimming until attaining 400 g in body weight thereafter progressively replacing this with more active swimming in yearling animals (1,500 g) (Davenport and Clough 1986). North Pacific loggerhead turtle body weights of 400 and 1,500 g are estimated to correspond to lengths of about 12.3 and 19.5 cm SCL, respectively, according to a growth model (Uchida 1967) and hence, to ages of about 1.0 and 3.1 years, respectively (Chaloupka 1998). Based on this, loggerheads seem to be passively transported by the Kuroshio Current followed by the Kuroshio Extension for 1.0–3.1 years. Particles released off Yakushima reached a maximum distance of 35°N, 160°W in 1.0 years, which is about 4,500 km away from Baja, and reached 30°N, 135°W in 3.1 years, which is about 2,200 km away from Baja. According to satellite tracking results (Polovina et al. 2006), juvenile loggerheads released off the Japan coast (25.6–64.8 cm SCL) moved at speeds of 23.5 cm s−1 (20.3 km day−1) on average, east of the date line. If 1.0–3.1-year-old turtles actively swim at this speed and use the following current eastward, in theory, hatchlings could reach the coastal areas off Baja California in 1.6–3.4 years at the earliest, although this assumed swimming speed is likely to be an overestimate, due to the much smaller size of turtles leaving Japan as hatchlings than those used by Polovina et al. (2006). If these small turtles do not swim actively, the possibility exists that they arrive following rapid direct drift, which might not be captured by the particle tracking model, but could be revealed by occasional drifter buoys that travel very fast in straight lines across oceans (e.g., Monzón-Argüello et al. 2010). These facts indicate the possibility that actual growth rates of juvenile loggerhead turtles in the north Pacific are somewhat higher than those estimated by Chaloupka (1998) and may be more similar to those estimated for the North Atlantic by Bjorndal et al. (2003).
Our simulation demonstrated that the distribution of particles over the simulation period was markedly different among release points. For example, the distribution of the particles released off Okinawa was roughly divided into two groups 180 days after release. One group was transported eastward, while in the other group, a considerable number of particles drifted in the Kuroshio Countercurrent in the western Pacific (Fig. 2b); almost all particles released from Yakushima were transported eastward (Fig. 2a). Consequently, even 5 years after release, 30.2% of surviving particles remained in the western North Pacific (areas I–III; Table 1). This is compatible to findings that loggerhead turtles captured in the central North Pacific vary in size from 12.3 to 74.0 cm SCL (Zug et al. 1995; Parker et al. 2005), which represents an estimated age range of 1.0–10 years or more. In addition, turtles ranging from 50 to 60 cm SCL were often observed in eastern coastal areas of Japan (Narazaki et al. 2010) and in the western North Pacific (Yokota et al. 2006), which corresponds to smaller sizes in the distribution of turtles observed in coastal areas off Baja California (Gardner and Nichols 2001; Koch et al. 2006; Peckham et al. 2008).
These facts and our simulation results led us to hypothesize that not all loggerhead turtles in the North Pacific always make the trans-Pacific dispersals revealed by previous genetic studies (Bowen et al. 1995; Hatase et al. 2002a) and that trans-Pacific dispersal is not necessary to the life history of Japanese loggerhead turtles. Spending their lives in an oceanic habitat without entering the neritic zone might organize the population of pelagic-feeding female turtles nesting in Japan (Hatase et al. 2002b). Our results indicate that simulated particles encounter the Kuroshio Countercurrent within 180 days. As hatchlings grow into juvenile turtles and their swimming ability improves, they are hypothesized to show directional swimming to help them stay in broadly favorable areas (Lohmann et al. 2001), indicating that they might modify their direction of movement to avoid drifting in the Kuroshio Countercurrent. However, even after 180 days, the estimated size of loggerhead turtles is still only about 8.3 cm SCL, according to the growth model described by Chaloupka (1998). Hence, during their first years of life, the assumption of passive drift is probably valid (Hays et al. 2010). However, further investigations are needed to determine whether all post-hatchling loggerhead turtles born in Japan show directional swimming at the bifurcation point of the Kuroshio Countercurrent and then migrate directly eastward without entering the countercurrent.
Our simulation is the first study demonstrating that the empirical temperature experienced by hatchlings throughout their transportation is essential when considering their ecology. The mean ambient water temperatures for particles in the east group reached 13 ± 3.8°C around 200 days (wintertime) after release (Fig. 6). Loggerhead hatchlings can react naturally in temperatures above 4.5°C (Schwartz 1978), while adult loggerhead turtles undertake dormancy at temperatures below 15°C (Ogren and McVea 1995) and become floaters at temperatures of less than 9.5°C (Schwartz 1978). Therefore, the cold tolerance of loggerhead hatchlings is likely to be essential for their survival and to allow them to complete trans-Pacific dispersal. However, whether this cold tolerance is maintained over long winter periods remains unknown, and further study into cold tolerance over long periods is needed.
Differences in passive transportation route led to differences in the temperatures experienced by particles during the simulation period. For particles released off Okinawa, the lowest temperatures for the east and west groups were over 20 and 16°C, respectively, at around 200 days, much higher than the temperatures encountered off Yakushima. This is probably because large numbers of particles drifted in the Kuroshio Countercurrent in the subtropical gyre of the North Pacific. Such differences in ambient temperature will lead to large differences in the accumulated ambient temperatures to which the turtles are exposed, and to consequent differences in growth rate during the juvenile stage, as shown for hawksbill (Eretmochelys imbricata) (Kobayashi and Shimizu 2010) and snapping turtles (Chelydra serpentina) (Williamson et al. 1989; Rhen and Lang 1999).
Our simulation also demonstrated that the survival rates of particles over the simulation period differed among release points. Many of the particles released off Wakayama landed, so the survival rate decreased rapidly after release (Fig. 3). Predation risks and energy requirements may increase with the distance from a nesting beach to a main current (Putman et al. 2010b). Putman et al. (2010b) also reported the possibility that the number of hatchlings being returned to coastal waters by filaments shed from the outer edges of a main current would increase. Wakayama is farther from the Kuroshio Current, to which hatchlings in this region migrate, than are the other two sites. Moreover, off Wakayama, branch currents generated from the Kuroshio Current to coastal areas prevail (Toda 1992; Nomura 2004). Therefore, the environmental conditions between the nesting beach and the main current system lead to a decrease in loggerhead nest density (Putman et al. 2010b). In fact, the nesting frequency of loggerhead turtles in Wakayama (Minabe Senri and Minabe Iwashiro beaches) is historically much lower than that in Yakushima (Inakahama and Maehama beaches) (Kamezaki et al. 2003), although comparison with Okinawa is difficult due to the lack of nesting data recorded for the whole nesting season over the years. Even when particles entered the Kuroshio, most drifted in the cyclonic eddy generated around the Kuroshio frontal area. Therefore, particles transported to the east were sparse compared to those released from the other two sites. In the cyclonic eddy, nutrients are supplied to the euphotic layer by upwelling, accelerating primary production and subsequent copepod production (Lee et al. 1981; Yoder et al. 1981; Kimura et al. 1997; Nakata et al. 2000). Therefore, many hatchling predators may accumulate in eddies, and predation risk may be enhanced.
In conclusion, our numerical simulation model predicts that the transportation and distribution of loggerhead turtle hatchlings in the North Pacific vary widely among nesting beaches. A considerable number of hatchlings are transported to waters east of the date line by drifting with the Kuroshio Current, followed by the Kuroshio Extension, while some hatchlings drift in the Kuroshio Countercurrent and remain in the western Pacific. Differences in dispersal route will lead to differences in the accumulated ambient temperatures to which the turtles are exposed and to consequent differences in growth rates during the juvenile stage. Severe environmental conditions between the nesting beach and the main current system lead to increased hatchling mortality.
References
Bjorndal KA, Bolten AB, Dellinger T, Delgado C, Martins HR (2003) Compensatory growth in oceanic loggerhead sea turtles: response to a stochastic environment. Ecology 84:1237–1249
Bowen BW, Abreugrobois FA, Balazs GH, Kamezaki N, Limpus CJ, Ferl RJ (1995) Trans-Pacific migrations of the loggerhead turtle (Caretta caretta) demonstrated with mitochondrial DNA markers. Proc Natl Acad Sci USA 92:3731–3734
Carr AF (1987) New perspectives on the pelagic stage of sea turtle development. Conserv Biol 1:103–121
Carr AF, Meylan AB (1980) Evidence of passive migration of green turtle hatchlings in sargassum. Copeia 1980:366–368
Chaloupka M (1998) Polyphasic growth in pelagic loggerhead sea turtles. Copeia 1998:516–518
Davenport J, Clough W (1986) Swimming and diving in young loggerhead sea turtles (Caretta caretta L.). Copeia 1986:53–57
Dodd CK Jr (1988) Synopsis of the biological data on the loggerhead sea turtle Caretta caretta (Linnaeus 1758). US Fish Wildl Serv Biol Rep 88(14), US Department of the Interior, Washington, DC
Gardner SC, Nichols WJ (2001) Assessment of sea turtle mortality rates in the Bahia Magdalena region, Baja California Sur, Mexico. Chel Cons Biol 4:197–199
Hamner WM (1988) The lost year of the sea turtle. Trends Ecol Evol 3:116–118
Hatase H, Kinoshita M, Bando T, Kamezaki N, Sato K, Matsuzawa Y, Goto K, Omuta K, Nakashima Y, Takeshita H, Sakamoto W (2002a) Population structure of loggerhead turtles, Caretta caretta, nesting in Japan: bottlenecks on the Pacific population. Mar Biol 141:299–305
Hatase H, Takai N, Matsuzawa Y, Sakamoto W, Omuta K, Goto K, Arai N, Fujiwara T (2002b) Size-related differences in feeding habitat use of adult female loggerhead turtles Caretta caretta around Japan determined by stable isotope analyses and satellite telemetry. Mar Ecol Prog Ser 233:273–281
Hays GC, Marsh R (1997) Estimating the age of juvenile loggerhead sea turtles in the North Atlantic. Can J Zool 75:40–46
Hays GC, Fossette S, Katselidis AK, Mariani P, Schofield G (2010) Ontogenic development of migration: lagrangian drift trajectories suggest a new paradigm for sea turtles. J R Soc Interface 7:1319–1327
Kamezaki N, Matsuzawa Y, Abe O et al (2003) Loggerhead turtles nesting in Japan. In: Bolten AB, Witherington B (eds) Loggerhead sea turtles. Smithisonian Books, Washington DC, pp 210–218
Kim H, Kimura S, Shinoda A, Kitagawa T, Sasai Y, Sasaki H (2007) Effect of El Niño on migration and larval transport of the Japanese eel (Anguilla japonica). ICES J Mar Sci 64:1387–1395
Kimura S, Kasai A, Nakata H, Sugimoto T, Simpson JH, Cheok JVS (1997) Biological productivity of meso-scale eddies caused by frontal disturbances in the Kuroshio. Ices J Mar Sci 54:179–192
Kitagawa T, Kato Y, Miller MJ, Sasai Y, Sasaki H, Kimura S (2010) The restricted spawning area and season of Pacific bluefin tuna facilitate use of nursery areas: a modeling approach to larval and juvenile dispersal processes. J Exp Mar Biol Ecol 393:23–31
Kobayashi M, Shimizu T (2010) Development of stock enhancement technology for hawksbill turtle. Aquabiology 190:436–442 (in Japanese with English abstract)
Koch V, Nichols WJ, Peckham H, de la Toba V (2006) Estimates of sea turtle mortality from poaching and bycatch in Bahia Magdalena, Baja California Sur, Mexico. Biol Conserv 128:327–334
Lee T, Atkinson L, Legeckis R (1981) Observations of a Gulf Stream frontal eddy on the Georgia continental shelf, April 1977. Deep Sea Res 28:347–378
Lohmann KJ, Cain SD, Dodge SA, Lohmann CMF (2001) Regional magnetic fields as navigational markers for sea turtles. Science 294:364–366
Luschi P, Hays GC, Papi F (2003) A review of long-distance movements by marine turtles, and the possible role of ocean currents. Oikos 103:293–302
Masumoto Y, Sasaki H, Kagimoto T, Komori N, Ishida A, Sasai Y, Miyama T, Motoi T, Mitsudera H, Takahashi K, Sakuma H, Yamagata T (2004) A fifty-year eddy-resolving simulation of the world ocean: preliminary outcomes of OFES (OGCM for the earth simulator). J Earth Simulator 1:35–56
Matsuzawa Y, Sato K, Sakamoto W, Bjorndal KA (2002) Seasonal fluctuations in sand temperature: effects on the incubation period and mortality of loggerhead sea turtle (Caretta caretta) pre-emergent hatchlings in Minabe, Japan. Mar Biol 140:639–646
Monzón-Argüello C, López-Jurado LF, Rico C, Marco A, López P, Hays GC, Lee PLM (2010) Evidence from genetic and Lagrangian drifter data for transatlantic transport of small juvenile green turtles. J Biogeogr 37:1752–1766
Musick JA, Limpus CJ (1997) Habitat utilization and migration in juvenile sea turtles. In: Lutz PL, Musick JA (eds) The biology of sea turtles. CRC Press, Boca Raton, pp 137–163
Nakata H, Kimura S, Okazaki Y, Kasai A (2000) Implications of meso-scale eddies caused by frontal disturbances of the Kuroshio current for anchovy recruitment. ICES J Mar Sci 57:143–151
Narazaki T, Takuma S, Murasaki H, Sato K, Miyazaki N (2010) Report of by-catch survey at Sanriku coastal water in 2005–2009. Umigame Newsletter Japan 83:25
Nishimura S (1967) The loggerhead turtles in Japan and neighboring waters (Testudinata: Cheloniidae). Publ Seto Mar Biol Lab 15:19–35
Nomura K (2004) Kii Peninsula. In: The Ministry of the Environment, Japanese Coral Reef Society (eds) Coral reefs of Japan, The Ministry of the Environment, Japan, pp 250–257
Ogren L, McVea CJ (1995) Apparent hibernation by sea turtles in North American Waters. Bjorndal KA (ed), Biology and conservation of sea turtles (revised edition): Smithsonian Institution Press, Washington, pp 127–132
Parker DM, Cooke WJ, Balazs GH (2005) Diet of oceanic loggerhead sea turtles (Caretta caretta) in the central North Pacific. Fish Bull 103:142–152
Peckham SH, Maldonado Diaz D, Koch V, Mancini A, Gaos A, Tinker MT, Nichols WJ (2008) High mortality of loggerhead turtles due to bycatch, human consumption and strandings at Baja California Sur, Mexico, 2003 to 2007. Endanger Species Res 5:171–183
Pitman RL (1990) Pelagic distribution and biology of sea turtles in the Eastern Tropical Pacific. In: Richardson TH, Richardson JI, Donnelly M (comps). Proceedings of the tenth annual workshop on sea turtle biology and conservation, NOAA Tech Memo NMFS-SEFC-278, pp 143–148
Polovina JJ, Uchida I, Balazs GH, Howell E, Parker DM, Dutton P (2006) The Kuroshio extension bifurcation region: a pelagic hotspot for juvenile loggerhead sea turtles. Deep-Sea Res II 53:326–339
Putman NF, Shay TJ, Lohmann KJ (2010a) Is the geographic distribution of nesting in the Kemp’s ridley turtle shaped by the migratory needs of offspring? Integr Comp Biol 50:305–314
Putman NF, Bane JM, Lohmann KJ (2010b) Sea turtle nesting distributions and oceanographic constraints on hatchling migration. Proc R Soc B 277:3631–3637
Rhen T, Lang JW (1999) Temperature during embryonic and juvenile development influences growth in hatchling snapping turtles, Chelydra serpentine. J Therm Biol 24:33–41
Sasaki H, Nonaka M, Masumoto Y, Sasai Y, Uehara H, Sakuma H (2008) An eddy-resolving hindcast simulation of the quasiglobal ocean from 1950 to 2003 on the earth simulator. In: Ohfuchi W, Hamilton K (eds) High resolution numerical modelling of the atmosphere and ocean. Springer, New York, pp 157–185
Schwartz FJ (1978) Behavioral and tolerance responses to cold water temperatures by three species of sea turtles (Reptilia, Cheloniidae) in North Carolina. Fla Mar Res Publ 33:16–18
Seminoff JA, Resendiz A, Resendiz B, Nichols WJ (2004) Occurrence of loggerhead sea turtles (Caretta caretta) in the Gulf of California, Mexico: evidence of life-history variation in the Pacific Ocean. Herp Rev 35:24–27
Sverdrup HU, Johnson MW, Fleming RH (1942) The oceans: their physics, chemistry, and general biology. Prentice-Hall, Englewood Cliffs, New York
Toda T (1992) Double structure of the coastal front in the Kii channel, Japan during winter. J Geophys Res Oceans 97:11333–11342
Uchida I (1967) On the growth the loggerhead turtles, Caretta caretfa, under rearing conditions. Bull Jpn Sot Sci Fish 33:497–507
Uchida I, Nishiwaki M (1982) Sea turtles in the waters adjacent to Japan. In: Bjorndal KA (ed) Biology and conservation of sea turtles. Smithsonian Institution Press, Washington, DC, pp 317–319
Williamson LU, Spotila JR, Standora EA (1989) Growth, selected temperature and CTM of young snapping turtles, Chelydra serpentine. J Therm Biol 14:33–39
Witherington BE (2002) Ecology of neonate loggerhead turtles inhabiting lines of down welling near a Gulf Stream front. Mar Biol 140:843–853
Yoder J, Atkinson L, Lee T, Kim H, McLain C (1981) Role of Gulf Stream frontal eddies informing phytoplankton patches on the outer southeast shelf. Limnol Oceanogr 26:1103–1110
Yokota K, Minami H, Nobetsu T (2006) Research on mitigation of the interaction of sea turtle with pelagic longline fishery in the western North Pacific. Proceedings of 3rd International Symposium SEASTAR 2000 and Asian Bio-logging Science, pp 3–8
Zenimoto K, Kitagawa T, Miyazaki S, Sasai Y, Sasaki H, Kimura S (2009) The effects of seasonal and interannual variability of oceanic structure in the western Pacific north equatorial current on larval transport of the Japanese eel Anguilla japonica. J Fish Biol 74:1878–1890
Zug GR, Balazs GH, Wetherall JA (1995) Growth in juvenile loggerhead sea turtles (Caretta caretta) in the North Pacific pelagic habitat. Copeia 2:484–487
Acknowledgments
We would like to thank to Japan Agency for Marine–Earth Science and Technology (JAMSTEC) for allowing us to use the OFES simulation data. This study was partly supported by Kyoto University Global COE Program: Informatics Education and Research Center for Knowledge-Circulation Society.
Author information
Authors and Affiliations
Corresponding author
Additional information
Communicated by J. Houghton.
Rights and permissions
About this article
Cite this article
Okuyama, J., Kitagawa, T., Zenimoto, K. et al. Trans-Pacific dispersal of loggerhead turtle hatchlings inferred from numerical simulation modeling. Mar Biol 158, 2055–2063 (2011). https://doi.org/10.1007/s00227-011-1712-9
Received:
Accepted:
Published:
Issue Date:
DOI: https://doi.org/10.1007/s00227-011-1712-9