Abstract
Sleep abnormalities in idiopathic Parkinson’s disease (PD) frequently consist in a reduction of total sleep time and efficacy and subsequent excessive daytime sleepiness. As it remains unclear whether these phenomena are part of the disease itself or result from pharmacological treatment, animal models for investigating the pathophysiology of sleep alterations in PD may add knowledge to this research area. In the present study, we investigate whether changes in circadian motor activity occur in 6-OHDA-lesioning model for PD, and allow a screening for disturbed sleep–waking behaviour. Activity measurements of six male Wistar rats with 6-OHDA-lesions in the medial forebrain bundle and six controls were carried out in two consecutive 12:12 h light–dark (LD) cycles. A computer-based video-analysis system, recording the animals’ movement tracks was used. Distance travelled and number of transitions between movement periods and resting periods were determined. Although 6-OHDA-lesioned animals show a reduced locomotor activity compared to non-lesioned rats, the circadian distribution basically remained intact. However, some lesioning effects were more pronounced in the resting phase than in the activity phase, possibly paralleling nocturnal akinesia in PD. In order to further elucidate the described phenomena, it will be necessary to perform studies combining sleep recordings with locomotor activity measurements.
Similar content being viewed by others
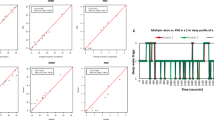
Avoid common mistakes on your manuscript.
Introduction
Sleep abnormalities in idiopathic Parkinson’s disease (PD) are a common complaint (Lees et al. 1988; Rye 2004) and consist of a reduction of total sleep time and slow-wave sleep as well as REM-sleep alterations (Wetter et al. 2001). Sleep fragmentation prevails in a majority of PD patients (van Hilten et al. 1994). Also excessive daytime sleepiness, controversially discussed as “sleep attacks”, is a problem frequently occurring in these patients (Frucht et al. 1999; Clarenbach et al. 2000). Whether these phenomena are part of the disease itself or results of pharmacological treatment is still unclear (Arnulf et al. 2002; Rye 2004). Therefore, animal models for investigating the pathophysiology of sleep alterations in PD may add knowledge to this research area.
A widely used model for investigations in PD is the unilateral 6-hydroxy-dopamine (6-OHDA) lesioning model of the medial forebrain bundle (Schwarting and Huston 1996). The present study was designed to measure spontaneous locomotor activity in this model over the course of time. We aimed at investigating whether changes in the circadian distribution of motor activity occur, as indicator for impaired sleep–waking behaviour.
Materials and methods
Animal experiments were conducted in accordance with NIH principles of laboratory animal care and were approved by the Government of Lower Saxony, Germany. The experiments were performed on 12 young adult male Wistar rats with a body weight of approximately 300 g at the time of lesioning. Before surgery the animals were kept in pairs and after surgery individually, with visual and olfactory contact to each other, in a ventilated, sound-attenuated room. Animals were subjected to a 12:12 h light–dark cycle (LD cycle, lights on at 0400 hours) with a room temperature of 22±1°C. Illumination intensity at the light period was 150 and 5 lux red-light in the dark (Isobe and Nishino 2001). Food and water were available ad libitum.
6-OHDA lesioning
Animals were pretreated with desipramine hydrochloride (DMI; 25 mg/kg, i.p.; 12.5 mg/ml; 0.2 ml/100 g of animal weight) 20 min before anaesthesia. Surgery was performed under deep intraperitoneal ketamin/xylazin anaesthesia (40 mg/kg body weight and 5 mg/kg body weight, respectively). The animal was positioned in a sterotaxic operation frame (TSE, Germany). Through a burr hole (position: A +2.8 mm and L +2.0 mm relative to the bregma) a metal canula (∅: 0.47 mm; deep +8.6 mm relative to the dura) was introduced close to the medial forebrain bundle. Thirty to forty-five minutes after pretreatment with DMI, 6-OHDA (4 μl of 3.75 mg/ml 6-OHDA, Sigma, dissolved in 0.1% ascorbic acid) was instilled at a rate of 0.5 μl/min. The needle remained in situ for further 4 min and was then slowly retracted. For sham lesioning 0.1% ascorbic acid alone was injected. The 6-OHDA-lesion was evaluated with amphetamine-induced (AMP; Sigma 2 mg/kg i.p.) rotational testing on day 23 post-lesioning (i.e. after activity measurements). Animals were placed in an automated rotameter bowl (TSE, Germany), and left and right full-body turns were counted. AMP net rotations over a period of 60 min, starting 30 min after injection were counted and the mean number of rotations per minute was calculated.
Data acquisition and analysis
Activity measurements were performed beginning on day 21 post-lesion, using a computer-based video-analysis system (Videomot, TSE, Germany). The animals were placed in topless acrylic glass observation cages with a ground area of 35×35 cm2 and a height of 50 cm directly after lesioning (to allow habituation). Water and food were available ad libitum. Video recordings of four animals (two non-lesioned, two lesioned) at a time were carried out for two consecutive 12:12 h LD cycles, beginning with lights-off. A video camera was placed above the four cages and connected to a standard PC computer system. Camera sensitivity was adjusted to the changes in light conditions. Each of the four animals’ position was calculated on-line at intervals of 2 s with the video analysis system and stored as a movement track on hard disk. Analysis of the animals’ movement tracks was then performed off-line after completion of data acquisition. We defined the following parameters of locomotor activity:
Distance (D): The length of the animals’ movement tracks (in m) as sum of the distances between consecutive tracking points. The following parameters were calculated for all individual animals: total distance (TD) in one LD cycle, distance covered in a 12:12 LD period, in intervals of 2 h (D/2 h) for the entire recording and the ratio D/12 h L vs D/12 h D.
Transitions (T): Due to head and chest movements (breathing), the animals’ tracking points vary slightly without actual change of the animals’ position. Therefore, we defined a locomotion period as prevailing only when the distance between two consecutive tracking points exceeded a threshold of 1 cm. By this threshold also movements through grooming and rearing were counted as movement time. In resting periods the distance between consecutive tracking points was below this threshold. The number of transitions from a movement period to a resting period were counted. The following parameters were calculated for all individual animals: total number of T in one LD cycle (TT), T in 12 h light period (T/12 h L), in 12 h dark period (T/12 h D), in intervals of 2 h (T/2 h) and the ratio T/12 h L vs T/12 h D.
Statistical analysis
For all the above described parameters intraindividual means of the two consecutive recording days were calculated to reduce the influence of accidental disturbances. Group means were calculated and statistical analysis of possible 6-OHDA-lesion-related changes in all determined parameters were performed by use of a two-way ANOVA with group (lesioned vs control) and condition (light vs dark) or 2 h interval 1–12 as factors to be compared. When statistically significant, all pairwise multiple comparison procedures (Tukey-Test) were used to find the factors contributing to these effects (analysis software Sigma Plot 7.101 and Sigma Stat 2.03 for Windows, SPSS Inc.,).
For purposes of determining whether there were changes in the circadian distribution of locomotor activity an iterative least squares 3-parameter-cosine fit (Regression Wizard, Sigma Plot 7.101 for Windows, SPSS Inc.,) was performed for distance covered and transitions in 2 h blocks for each individual animal, thus allowing the calculation of the three parameters offset, amplitude and cycle duration. For these parameters, as well as light/dark ratio, for distance and transitions, respectively, means were calculated and compared between the two groups by use of a one-way ANOVA.
Histology and immunocytochemistry
At the end of the experiment animals were transcardially perfused under deep ketamine anaesthesia (100 mg/kg body weight) with 100 ml followed by 200 ml of paraformaldehyde (4% in PBS). Brains were fixed in the same solution, cryoprotected and frozen. Thirty micrometre coronal sections were cut on a cryostat and divided into series. After incubation in 3% hydrogen peroxide in PBS and blocking with 3% normal goat serum, separate sections were incubated overnight in polyclonal rabbit anti-TH (TH; 1:500; Biotrend).
Results
Rotametry and histology
Whereas no rotational behaviour was observed in the sham-lesioned animals after injection of amphetamine, the 6-OHDA-lesioned animals showed 8.4±1.4 rotations/min, indicating a severe depletion of striatal dopaminergic enervation. This could be confirmed by histology, which in TH-staining showed complete depletion of dopaminergic terminals ipsilateral to the injection of 6-OHDA in the lesioned animals.
Activity measurements
Before the start of the experiment and intermittently in the running experiment animals were observed in parallel to video tracking. Following patterns were seen:
1. Animal is moving continuously: high distance, but no transition.
2. Animal is exploring, grooming, rearing: low distance, high number of transitions.
3. Animal is not moving (sleeping, quit wakefulness, eating, drinking): no distance, no transitions.
These observations were not further quantified, but used to interpret the findings our measurements.
Distance (D)
For one LD cycle the total distance (TD) was shorter in 6-OHDA-lesioned animals (377±25 m) than in control animals (647±31 m, two-way ANOVA, P<0.001, see Table 1). In both groups the TD was subjected to clear circadian changes, in the sense that within the groups distance was markedly reduced in the light/resting phase (control: 196±16 m; lesioned: 85±9 m; two-way ANOVA, P<0.001) compared to the dark/activity phase (control: 450±16 m; lesioned: 293±16 m; two-way ANOVA, P<0.001, with statistical difference for lesioned vs control, as well as light vs dark as compared factors). The circadian distribution is shown in Fig. 1a, a two-way ANOVA with time (i.e. blocks of 2 h) and with lesioned vs control as factors revealed significant differences between both groups for nearly all intervals (see Fig. 1a, statistical significances marked with an asterisk, tendencies shown with P value). The ratio of distance in light/resting phase vs dark/activity phase was significantly lower in the lesioned group (0.29±0.01) than in the control group (0.43±0.02; one-way ANOVA, P<0.001). Results of the cosine fit are displayed in Fig. 1a and Table 1, there were no changes in cycle duration.
Circadian distribution of distance covered and number of transitions of two consecutive light–dark cycles (12:12 h, lights on at 400 hours) for lesioned (n=6, empty circles) and control animals (n=6, filled circles). Data represent group means over 2 h ± SEM. Statistical significant differences between groups revealed by a two-way ANOVA (see Materials and methods section) are marked with an asterisk, tendencies are shown with P value. a Distance covered. Control animals cover significantly longer distances than lesioned animals, circadian distribution (cycle duration), is similar. Thus distance over time can be fitted with a cosine curve for both investigated groups (broken lines). b Number of transitions. Significant difference between controls and lesioned animals is seen prevailing in the animals resting period
Transitions (T)
The number of transitions over the entire LD cycle was reduced in lesioned animals (514±28) compared to the control group (642±23; two-way ANOVA, P<0.001). As for TD, the distribution of transitions over time followed circadian changes within each group, with significantly higher T/12 h D (activity phase, controls: 419±10; lesioned group 378±16, ANOVA, P<0.001) than in the resting phase (T/12 h L; controls: 223±13; lesioned group: 136±10 ANOVA, P<0.001). There was only one interval, where there was a significant difference in transitions between both groups in the activity phase, whereas most intervals differed in the resting phase (see Fig. 1b, statistical significances marked with an asterisk, tendencies shown with P value). The ratio of transitions in light/resting phase vs dark/activity phase was significantly lower in the lesioned group (0.36±0.02) than in the control group (0.54±0.03; one-way ANOVA, P<0.001). Results of the cosine fit are displayed in Fig. 1b and Table 1.
Discussion
Although animals lesioned with 6-OHDA in the medial forebrain bundle of the striato-nigral system show a reduced motor activity compared to non-lesioned rats, we could demonstrate that the circadian distribution of covered distance remained unchanged. In phases of high motor activity (e.g. exploratory behaviour) we observed a large number of transitions between resting and moving, whereas in phases of low motor activity (e.g. sleeping, quiet wakefulness, but also food intake) fewer transitions occurred. Thus the combined observation of transitions and covered distance allow an impression not only of locomotor activity, but also of activation in general.
In this study, we used a commercially available system for the analysis of video recordings of freely moving animals (TSE-Systems, Bad Homburg, Germany) to measure circadian changes in locomotor activity. This is a new application of this method, which in the past has been more widely used for the investigation of locomotor behaviour in shorter observation periods [e.g. for studies with the open field (Siemiatkowski et al. 2000) or elevated plus maze (Ebner et al. 2004)]. The presented method does not allow a definite distinction between wakefulness and sleep, however, Storch et al. 2004 recently validated in a comparative study between EEG recordings and activity measures, that activity patterns of mice represent a reliable and sensitive measurement of quantitative aspects of sleep–wake behaviour. Furthermore, in a sleep disturbance resembling the one seen in human parkinsonism, a change in the circadian distribution of covered distance and transitions would be expected (i.e. increased activity in the resting phase and reduced activity in the activity phase due to “daytime sleepiness”).
Little is known about circadian changes of motor activity in animal models for PD so far. To our knowledge this is the first study concentrating on impairments of circadian locomotor activity in the 6-OHDA rat model for PD using lesions in the medial forebrain bundle of the striato-nigral system (A9) of rats. Bilateral lesions of the medial forebrain bundle lead to a massive impairment of the animals, requiring tube feeding (Winkler 2002) and are therefore not suitable for observations of circadian locomotor activity. Ben and Bruguerolle (2000) telemetrically measured motor activity after bilateral striatal lesions with 6-OHDA, which although bilateral, rather are a model for slight to moderate PD, mainly affecting the striato-nigral pathway and revealed a decrease in the offset of circadian rhythm, with an unchanged cycle duration, similar to our findings in unilaterally medial forebrain bundle lesioned animals.
As in PD not only the nigro-striatal, but also the mesolimbic dopaminergic system is affected, lesioning of dopaminergic neurons in the ventral tegmental area (A 10) could contribute to understand changes in biological rhythms seen in PD. Isobe and Nishino (2001) found that after bilaterally 6-OHDA lesioning the A10 nuclei, locomotor activity was decreased during the activity/dark period and increased during the light period, and the cycle duration elongated. No changes in behavioural activity in the light period were seen by Sakata et al. (2002). Increased activity in the activity phase seen by others (Oades et al. 1986) was explained by the use of lower doses of 6-OHDA (Isobe and Nishino 2001).
Experiments in the 1-methyl-4-phenyl-1,2,3,6-tetrahydropyridine (MPTP) model for PD are inconsistent regarding the impact on circadian rhythms as well. MPTP-lesioning seems to abolish the circadian rhythm of vegetative parameters in dogs (Hineno et al. 1992) and selectively suppresses REM sleep in cats (Pungor et al. 1990). The impact on the circadian rhythm of motor activity was not investigated in those studies. In MPTP-treated monkeys circadian alterations of heart rate, body temperature and activity level correlated with motor impairment (Almirall et al. 2001), possibly due to changes of hormonal metabolism in chronic MPTP-treated parkinsonian monkeys (Barcia et al. 2003).
Both in untreated early patients and treated PD patients in advanced stages, nocturnal akinesia is one of the major factors leading to sleep disturbance (Laihinen et al. 1987; Lees et al. 1988). Interestingly, the lesioned animals in our study differed from controls by reduced ratios of distance and transitions between resting vs activity phase, as a possible equivalent to the nocturnal akinesia seen in PD patients. Whereas the usually low dosage of dopaminergic medication at night can explain this phenomenon in PD patients, one can speculate that the circadian rhythm of dopamine release of the intact A9—with a reduced dopamine turnover in the light phase (Hutson et al. 1984)—may lead to this observation in non-treated hemi-parkinsonian rats.
In summary one may speculate, that whereas lesions affecting mainly the A10 neurons lead to changes in circadian rhythms of motor activity, lesions in the A9 neurons primarily affect motor performance. Combined lesions, as in the MPTP-model, would then lead to a combination of those effects, but were, so far, not specifically investigated.
Although the presented method has certain limitations, it allows a screening for circadian changes in motor activity and thus quantitative changes in sleep–wake behaviour. In order to further elucidate the observed phenomena, and to assess qualitative changes in sleep–wake behaviour it is necessary to perform studies with a combination of sleep recordings and measurements of locomotor activity. Selective lesions of the A9 and A10 neurons will then have to be compared with more generalized depletion of the dopaminergic system, e.g. by MPTP.
References
Almirall H, Bautista V, Sanchez-Bahillo A, Trinidad-Herrero M (2001) Ultradian and circadian body temperature and activity rhythms in chronic MPTP treated monkeys. Neurophysiol Clin 31:161–170
Arnulf I, Konofal E, Merino-Andreu M, Houeto JL, Mesnage V, Welter ML, Lacomblez L, Golmard JL, Derenne JP, Agid Y (2002) Parkinson’s disease and sleepiness: an integral part of PD. Neurology 58:1019–1024
Barcia C, Bautista V, Sanchez-Bahillo A, Fernandez-Villalba E, Navarro-Ruis JM, Barreiro AF, Poza YPM, Herrero MT (2003) Circadian determinations of cortisol, prolactin and melatonin in chronic methyl-phenyl-tetrahydropyridine-treated monkeys. Neuroendocrinology 78:118–128
Ben V, Bruguerolle B (2000) Effects of bilateral striatal 6-OHDA lesions on circadian rhythms in the rat: a radiotelemetric study. Life Sci 67:1549–1558
Clarenbach P, Greulich W, Meinck HM, Rathey B, Schipper H, Lachenmayer L, Werner M (2000) Workshop I: Parkinson’s disease and sleep—results of the group discussion. J Neurol 247 (Suppl 4):IV/34–35
Ebner K, Rupniak NM, Saria A, Singewald N (2004) Substance P in the medial amygdala: emotional stress-sensitive release and modulation of anxiety-related behavior in rats. Proc Natl Acad Sci USA 101:4280–4285
Frucht S, Rogers JD, Greene PE, Gordon MF, Fahn S (1999) Falling asleep at the wheel: motor vehicle mishaps in persons taking pramipexole and ropinirole. Neurology 52:1908–1910
Hineno T, Mizobuchi M, Hiratani K, Inami Y, Kakimoto Y (1992) Disappearance of circadian rhythms in Parkinson’s disease model induced by 1-methyl-4-phenyl-1,2,3,6-tetrahydropyridine in dogs. Brain Res 580:92–99
Hutson PH, Sarna GS, Curzon G (1984) Determination of daily variations of brain 5-hydroxytryptamine and dopamine turnovers and of the clearance of their acidic metabolites in conscious rats by repeated sampling of cerebrospinal fluid. J Neurochem 43:291–293
Isobe Y, Nishino H (2001) Circadian rhythm of drinking and running-wheel activity in rats with 6-hydroxydopamine lesions of the ventral tegmental area. Brain Res 899:187–192
Laihinen A, Alihanka J, Raitasuo S, Rinne UK (1987) Sleep movements and associated autonomic nervous activities in patients with Parkinson’s disease. Acta Neurol Scand 76:64–68
Lees AJ, Blackburn NA, Campbell VL (1988) The nighttime problems of Parkinson’s disease. Clin Neuropharmacol 11:512–519
Oades RD, Taghzouti K, Rivet JM, Simon H, Le Moal M (1986) Locomotor activity in relation to dopamine and noradrenaline in the nucleus accumbens, septal and frontal areas: a 6-hydroxydopamine study. Neuropsychobiology 16:37–42
Pungor K, Papp M, Kekesi K, Juhasz G (1990) A novel effect of MPTP: the selective suppression of paradoxical sleep in cats. Brain Res 525:310–314
Rye DB (2004) The two faces of Eve: dopamine’s modulation of wakefulness and sleep. Neurology 63:S2–S7
Sakata M, Sei H, Toida K, Fujihara H, Urushihara R, Morita Y (2002) Mesolimbic dopaminergic system is involved in diurnal blood pressure regulation. Brain Res 928:194–201
Schwarting RK, Huston JP (1996) The unilateral 6-hydroxydopamine lesion model in behavioral brain research. Analysis of functional deficits, recovery and treatments. [Review] [395 refs]. Prog Neurobiol 50:275–331
Siemiatkowski M, Rokicki D, Czlonkowska AI, Sienkiewicz-Jarosz H, Bidzinski A, Plaznik A (2000) Locomotor activity and a conditioned fear response: correlation with cortical and subcortical binding of the dopamine D1 receptor antagonist. Neuroreport 11:3953–3956
Storch C, Hohne A, Holsboer F, Ohl F (2004) Activity patterns as a correlate for sleep–wake behaviour in mice. J Neurosci Methods 133:173–179
van Hilten B, Hoff JI, Middelkoop HA, van der Velde EA, Kerkhof GA, Wauquier A, Kamphuisen HA, Roos RA (1994) Sleep disruption in Parkinson’s disease. Assessment by continuous activity monitoring. Arch Neurol 51:922–928
Wetter TC, Brunner H, Hogl B, Yassouridis A, Trenkwalder C, Friess E (2001) Increased alpha activity in REM sleep in de novo patients with Parkinson’s disease. Mov Disord 16:928–933
Winkler C (2002) Toward a restorative therapy for Parkinson’s disease—studies on the functional efficacy of intracerebral fetal cell transplants and GDNF delivery. Medical Department. Lund University, p 172
Acknowledgements
This work was supported by a grant of the German Federal Ministry of Education and Research (Bundesministerium für Bildung und Forschung—01GN0102). The authors thank Christine Crozier for a critical reading of the manuscript and Dr. K. Nebendahl and the team of our animal experimental facility for their support. Part of this work was performed as part of the doctoral thesis of PB.
Author information
Authors and Affiliations
Corresponding author
Rights and permissions
About this article
Cite this article
Baier, P.C., Branisa, P., Koch, R. et al. Circadian distribution of motor-activity in unilaterally 6-hydroxy-dopamine lesioned rats. Exp Brain Res 169, 283–288 (2006). https://doi.org/10.1007/s00221-005-0343-0
Received:
Accepted:
Published:
Issue Date:
DOI: https://doi.org/10.1007/s00221-005-0343-0