Abstract
The stratum corneum (SC) is the outermost layer of skin that functions as a barrier and protects against environmental influences and transepidermal water loss. Its unique morphology consists of keratin-enriched corneocytes embedded in a distinctive mixture of lipids containing mainly ceramides, free fatty acids, and cholesterol. Ceramides are sphingolipids consisting of sphingoid bases, which are linked to fatty acids by an amide bond. Typical sphingoid bases in the skin are composed of dihydrosphingosine (dS), sphingosine (S), phytosphingosine (P), and 6-hydroxysphingosine (H), and the fatty acid acyl chains are composed of non-hydroxy fatty acid (N), α-hydroxy fatty acid (A), ω-hydroxy fatty acid (O), and esterified ω-hydroxy fatty acid (E). The 16 ceramide classes include several combinations of sphingoid bases and fatty acid acyl chains. Among them, N-type ceramides are the most abundant in the SC. Mass spectrometry (MS)/MS analysis of N-type ceramides using chip-based direct infusion nanoelectrospray-ion trap mass spectrometry generated the characteristic fragmentation pattern of both acyl and sphingoid units, which could be applied to structural identification of ceramides. Based on the MS/MS fragmentation patterns of N-type ceramides, comprehensive fragmentation schemes were proposed. In addition, mass fragmentation patterns, which are specific to the sphingoid backbone of N-type ceramides, were found in higher m/z regions of tandem mass spectra. These characteristic and general fragmentation patterns were used to identify N-type ceramides in human SC. Based on established MS/MS fragmentation patterns of N-type ceramides, 52 ceramides (including different classes of NS, NdS, NP, and NH) were identified in human SC. The MS/MS fragmentation patterns of N-type ceramides were characterized by interpreting their product ion scan mass spectra. This information may be used to identify N-type ceramides in the SC of human, rat, and mouse skin.
Similar content being viewed by others
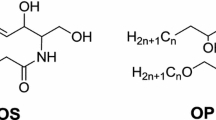
Avoid common mistakes on your manuscript.
Introduction
Ceramides, a subclass of sphingolipids, have an enormous structural diversity, resulting in a large number of ceramide species [1]. Ceramides are found in all tissues of the human body, but the greatest diversity is found in the skin or, more precisely, in the uppermost layer of the epidermis, i.e., the stratum corneum (SC) [2]. This layer of the epidermis consists of enucleate cells or corneocytes, which are embedded in a matrix of primarily ceramides (>50 % by weight), cholesterol and free fatty acids (between 15 and 20 wt.%), as well as minor fractions of triacylglycerols and phospholipids [3–6]. Ceramides belong to the sphingolipids, and in human SC, four types of sphingoid bases, which are linked to fatty acids by an amide bond. These include sphingosine (S), phytosphingosine (P), 6-hydroxysphingosine (H), and dihydrosphingosine (dS). The free fatty acids can be non-hydroxylated (N), α-hydroxylated (A), or ω-hydroxylated (O), of which the latter can additionally be esterified to a fatty acid (E) [7]. Among the four types of fatty acid bases, N-type ceramide is the most abundant in human SC (Fig. 1) [2]. All ceramide classes consist of several subspecies that differ in the chain length of the sphingoid base and amide-linked fatty acid. The most common chain length for the sphingoid base is C18, with a range of C12 to C22 [1]. The chain length of the amide-linked fatty acid varies from C16 to C40.
Various techniques have been applied to identify ceramide species in the SC. For example, HPTLC is commonly used in skin lipid research to identify ceramide subclasses in human SC [8, 9]. However, this approach is time-consuming and requires a large quantity of material, which is not always available. Liquid chromatography coupled to mass spectrometry (MS) has also been used for ceramide analysis [1, 2, 10–15]. Although beneficial to comprehensive analysis, the chromatographic step limits throughput, especially with a large number of samples [16]. Recently, direct infusion of lipid extracts into a tandem mass spectrometer without additional separation of the individual molecular species has been developed and applied to rapid analysis of phospholipids, triacylglycerols, and ceramides [17–20]. Shotgun lipidomic method was more than 10-fold faster than traditional methods because no chromatographic separation was required [20]. At this time, ceramide species in skin SC samples have not been analyzed using direct infusion methods. In addition, it is important to characterize mass fragmentation patterns specific to ceramide types to determine the structure of skin ceramides. Previously, Hinder et al. [14] reported the fragmentation pathway of NP-type ceramide using collision-induced dissociation in negative ion mode. The collision-induced dissociation pathways of NS-type ceramides after electrospray ionization were also partly elucidated by Han [21] and Hsu and Turk [13]. Additionally, the fragmentation scheme of NdS-type ceramide was proposed by Raith and Neubert [22]. At present, the comprehensive mass fragmentation patterns, as well as the exact structure of each fragment ion, of the four major N-type ceramide species remain unknown.
In this study, we characterized the MS/MS fragmentation pattern of four major N-type ceramides by interpreting their product ion scan mass spectra. We also developed a simple and rapid method of identifying and profiling N-type ceramide species in human SC using chip-based direct infusion nanoelectrospray-ion trap mass spectrometry. These methods were successfully applied to identification of N-type ceramides in human SC, and the MS/MS fragmentation patterns of N-type ceramides were characterized.
Experimental
Reagents
Ammonium acetate and chloroform were obtained from Sigma Chemical Co. (St. Louis, MO, USA). Methanol was purchased from Merck (Darmstadt, Germany). All chemicals and solvents were of analytical grade and commercially available. Synthetic ceramides CER[N(24:1)dS(18:0)], CER[N(18:0)dS(18:0)], CER[N(17:0)S(18:1)], CER[N(24:1)S(18:1)], CER[N(16:0)P(18:0)], and CER[N(24:0)P(18:0)] were purchased from Avanti Polar Lipids (Alabaster, AL, USA) or Matreya (Pleasant Gap, PA, USA).
Ceramide analysis and data processing
Ceramide profiling was performed on a LTQ XL mass spectrometer (Thermo Fischer Scientific, West Palm Beach, FL, USA) equipped with a robotic nanoflow ion source TriVersa Nanomate (Advion Biosciences, Ithaca, NY, USA) using nanoelectrospray chips with 5.5-μm-diameter spraying nozzles. The ion source was controlled using the Chipsoft 8.3.1 software (Advion Biosciences). Ionization voltage was −1.4 kV in negative mode, and backpressure was set at 0.4 psi. Ion transfer capillary temperature was 200 °C, and tube voltage was −100 V. Under these settings, 10 μL of the analyte could be electrosprayed for more than 50 min. For the analysis, 5 μL of samples was loaded onto 96-well plates (Eppendorf, Hamburg, Germany) of the TriVersa Nanomate ion source. Each sample was analyzed for 2 min. The data collection method involved a full scan (scan range: m/z 400–1,000) and a data-dependent MS/MS scan of the most abundant ions. Standard spectra were scanned in low-resolution mode with 30 eV CID voltage to obtain specific MS/MS fragmentations. All spectra were recorded with the Thermo Xcalibur software (version 2.1., Thermo Fisher Scientific), and skin ceramides were tentatively identified based on their specific MS/MS fragmentation patterns.
Sampling and sample preparation
Lipids of SC were obtained from healthy volunteers (20–33 years old, seven males and six females, Korean). All individuals were enrolled with informed consent. Skin lipids were obtained from each inner forearm (a total of four locations per sample) using the cup method modified from the procedure of Bonté et al. [23]. The skin surface was cleaned with 30 % ethanol, and five tape strippings using D-squame standard tape (Cuderm, Dallas, TX, USA, diameter, 2.2 cm) under constant pressure (180 g/cm2) were performed prior to solvent extraction to avoid sebum contamination. For solvent extraction, an 18-mm-diameter (40.72 cm2 for the four contact locations) glass cylinder was used. The solvent system used for the first extraction was 5 mL of cyclohexane/ethanol (1:4; v/v), and 5 mL of cyclohexane/ethanol (1:1, v/v) was used for the second extraction. The solvent extracts were combined and dried under a nitrogen stream at 50 °C. Total lipid extracts were then fractionated into three fractions, namely neutral lipids, free fatty acids, and ceramides using silicic acid column chromatography. Half of the dried extracts were reconstituted in 200 μL of chloroform and loaded onto silicic acid columns which were preconditioned with chloroform. After sample loading, each column was washed with 20 mL of chloroform to elute the neutral lipids, with 20 mL of chloroform containing 0.2 % acetic acid to elute free fatty acids, and finally with 30 mL of methanol to elute ceramides. The ceramide fractions were dried under nitrogen and stored at −70 °C until use. To measure ceramides, the ceramide fractions were dissolved in 100 μL of methanol/chloroform (9:1, v/v) and diluted 10-fold with methanol/chloroform (9:1, v/v) containing 7.5 mM ammonium acetate. Aliquots were subjected to the Nanomate-LTQ system to identify N-type ceramides in the samples.
Results and discussion
MS/MS identification of N-type ceramide
The targeted MS/MS analysis obtained by Nanomate-LTQ provided specific fragment ion information for both the acyl and sphingoid units, which can be used for structural identification of ceramides. The identification procedures for synthetic ceramide standards [N(17:0)S(18:1) (NS, MW = 551), N(24:0)dS(18:0) (NdS, MW = 651), and N(16:0)P(18:0) (NP, MW = 555)] were as follows: NH-type ceramide is commercially unavailable; therefore, the fragmentation pattern of this ceramide was proposed based on the MS/MS spectrum of N(20:0)H(17:1) ceramide (NH, MW = 693), which was found in the stratum corneum of human skin [2]. All N-type ceramides species were detected as mainly acetate adducts [M + CH3COO]− (>95 %) due to the ammonium acetate component of the reconstitution solvent system. The MS/MS spectra of these acetate adducts generated deprotonated molecular ions [M-H]−. Product ion scan spectra of these pseudomolecular ions [M-H]− provided informative fragmentation patterns for the structural identification of N-type ceramide species (Fig. 2).
Characterization of NS-type ceramide mass fragmentation patterns
The proposed fragmentation pathway of the non-hydroxy ceramide (NS type) standard is illustrated in Scheme 1 and described below. These patterns can be used to identify molecular species containing different acyl amide constituents. The MS/MS spectrum of ceramide N(17:0)S(18:1) obtained by fragmenting the pseudomolecular ion [M-H]− with m/z 550.5 through collision yielded an abundant product ions at m/z 520.7, which suggested that a CH2O group (a1) was lost and that cleavage induced the formation of an ion with the charge retained at the fatty acid with m/z 251.2 (a3). Ceramide NS can also lose both the CH2O group and water, resulting in a product ion at m/z 502.7 (a2). Cleavage at the C2–C3 (see structure a in Scheme 1 for numbering of the carbon atoms) bond resulted in a characteristic ion at m/z 312.3 (b1) with the charge on the fatty acid moiety, and a subsequent loss of water resulted in a product ion at m/z 294.3 (b2). Cleavage at the C2–C3 bond from pseudomolecular ion c resulted in a characteristic ion at m/z 310.3 (c1) with the charge on the fatty acid moiety. A prevalent neutral loss of water could occur at two different positions (c and f) and occurred with double bond formation (m/z 532.6). One double bond could form between C2 and C3 (d1), and subsequent cleavage at this location resulted in a product ion at m/z 263.3 (d2) and 268.3 (d3) with the charge on the sphingoid moiety and fatty acyl moiety, respectively. With a double bond at this position, a rearrangement (e) can further take place, resulting in a product ion at m/z 269.3 (e1), which is the fatty acid itself. A second possible position was located between C1 and C2 (f1), and cleavage of this position resulted in a product ion with m/z 237.3 and a charge located at the sphingoid base (f2).
Several characteristic fragments related to the fatty acid were observed, namely a3, b1, b2, c1, d3, and e1. Furthermore, d2 and f2 were characteristic fragment ions related to sphingosine. These results agreed with the findings of Hsu and Turk [13], Han [21], and Vietzke et al. [10]. Based on this ceramide NS fragmentation scheme, the composition of NS-type ceramide in human SC can be examined.
Characterization of the NdS-type ceramide mass fragmentation patterns
The proposed fragmentation pathway of NdS type ceramide is illustrated in Scheme 2 and described below. The MS/MS spectrum of the ceramide N(24:0)dS(18:0) standard by fragmenting the pseudomolecular ion [M-H]− with m/z 650.7 through collision yielded a product ions at m/z 602.8, suggesting that a CH2O group and water (a1) were lost and that cleavage at this location induced the formation of an ion with the charge retained at the fatty acid with m/z 349.5 (a2). Cleavage at the C2–C3 (see structure a in Scheme 2 for numbering of carbon atoms) bond resulted in a characteristic ion at m/z 410.5 (b1) with the charge on the fatty acid moiety, and a subsequent loss of water resulted in a product ion at m/z 392.6 (b2). Cleavage at the C2–C3 bond from pseudomolecular ion c also resulted in a characteristic ion at m/z 408.5 (c1) with the charge on the fatty acid moiety. A prevalent neutral loss of water could occur at two different positions (c and f) with a double bond formation (m/z 632.8). One double bond could be formed between C2 and C3 (d1), and a subsequent cleavage resulted in a product ion at m/z 366.5 (d2) with the charge on the fatty acyl moiety. With a double bond at this position (d1), a rearrangement (e) could further take place, resulting in a product ion at m/z 367.5 (e1), which is the fatty acid itself. A second possible position was located between C1 and C2 (f1), and cleavage at this location resulted in a product ion with m/z 239.3 and the charge located at the sphingoid base (f2). Ceramide N(24:0)dS(18:0) also showed abundant product ions at m/z 618.8, suggesting that loss of the methanol molecule (g1) and cleavage induced the formation of an ion with the charge retained at the sphingoid base with m/z 268.3 (g2).
Several characteristic fragments related to the fatty acid were observed, namely a2, b2, c1, d2, and e1. Furthermore, f2 and g2 were characteristic fragment ions related to sphingosine. These results are in agreement with the findings of Hsu and Turk [13] and Raith and Neubert [22].
Characterization of the NP-type ceramide mass fragmentation patterns
The MS/MS spectrum of ceramide N(16:0)P(18:0) by fragmenting the pseudomolecular ion [M-H]− with m/z 554.6 through collision showed a characteristic fragmentation pattern. Cleavage at the C2–C3 (see structure a in Scheme 3 for numbering of carbon atoms) bond resulted in a characteristic ion at m/z 298.3 (a1) with the charge on the fatty acid moiety, and a subsequent loss of water resulted in a product ion at m/z 280.3 (a2). NP-type ceramide could also show cleavage of the fatty acid chain, and this fragment ion showed a neutral loss of water (a3, m/z 298.3) and further loss of a CH2OH radical (a4, m/z 267.3). Cleavage at the C2–C3 bond from pseudomolecular ion b also resulted in a characteristic ion at m/z 296.3 (b1) with the charge on the fatty acid moiety. A prevalent neutral loss of water could occur at two different positions (b and d) with a double bond formation (m/z 536.7 and 518.7). Two double bonds due to the loss of two water molecules could form at the C2–C3 and C4–C5 bonds (c1, m/z 518.7), and a subsequent rearrangement (c2) could further take place, resulting in a product ion at m/z 255.3 (c3), which is the fatty acid itself. A second possible position was located between C1 and C2 (d1), and cleavage at this location resulted in a product ion with m/z 310.3 and a charge located at the fatty acyl moiety (d2). Ceramide NP (d) also showed abundant product ions at m/z 522.5 (d3), suggestive of loss of methanol molecule. Two double bonds due to the loss of two water molecules from d could also form at the C1–C2 and C4–C5 bonds (e1, m/z 518.7), and cleavage at the C2–C3 bond induced the formation of an ion with the charge retained at the sphingoid base with m/z 237.3 (e2). Several characteristic fragments related to the fatty acid were observed, namely a1, a2, a4, b1, c3, and d. Furthermore, e2 was a characteristic fragment ions related to sphingosine. These results are in agreement with the findings of Hsu and Turk [13], Hinder et al. [14], Raith and Neubert [22], and Vietzke et al. [10]. Hinder et al. [14] proposed a structure of this fragment ion (m/z 267.3), which was produced from N(18:0)P(18:0) ceramide; however, the m/z value of their structure was 266.3.
Characterization of the NH-type ceramide mass fragmentation patterns
NH-type ceramide is commercially unavailable; therefore, the fragmentation pattern of this ceramide was proposed based on the MS/MS spectrum of NH-type ceramide (MW = 693), which is found in the stratum corneum of human skin. The proposed fragmentation pathway of NH type ceramide is illustrated in Scheme 4 and described below. The MS/MS spectrum of the ceramide N(26:0)H(18:1) by fragmenting the pseudomolecular ion [M-H]− with m/z 692.5 through collision yielded product ions at m/z 662.8, suggesting that a CH2O molecule (a1) was lost. Ceramide NH could also show both loss of a CH2O group and water, resulting in a product ion at m/z 644.7 (a2). Cleavage at the C2–C3 (see structure a in Scheme 2 for numbering of carbon atoms) bond resulted in a weak ion at m/z 438.5 (b1) with the charge on the fatty acid moiety, and a subsequent loss of water resulted in a product ion at m/z 420.5 (b2). Cleavage at the C2–C3 bond from pseudomolecular ion c also resulted in a characteristic ion at m/z 436.5 (c1) with the charge on the fatty acid moiety. A prevalent neutral loss of water could occur at two different positions (c and f) with double bond formation (m/z 674.8). One double bond could form between C2 and C3 (d1), and a subsequent cleavage resulted in a product ion at m/z 394.5 (d2) with the charge on the fatty acyl moiety. With a double bond at this position (d1), a rearrangement (e) could further take place, resulting in a product ion at m/z 395.5 (e1), which is the fatty acid itself. A second possible double-bond position was located between C1 and C2 (f1), and cleavage at this location resulted in a product ion with m/z 253.2 and a charge located at the sphingoid base (f2). Several characteristic fragments related to the fatty acid were observed, including b1, b2, c1, d2, and e1. Furthermore, f2 was a characteristic fragment ion related to sphingosine. These results are in agreement with the findings of t’Kindt et al. [2].
Suggested fragmentation pathways of CER[NH] standard [N(26:0)H(18:1)] by means of collision induced dissociation in negative ion mode. This scheme has been prepared considering previous work [2]
General MS/MS fragmentation pattern of N-type ceramides
Comparison of the fragment pattern of N-type ceramides with different sphingoid backbones in the m/z region of the pseudomolecular ion of the MS/MS spectrum revealed general fragmentation patterns of N-type ceramides (Fig. 3). The MS/MS spectrum of NS- and NH-type ceramide resulted in a series of fragment ion masses with differences of 18, 30, and 48 Da, which originated from the loss of water, CH2O group, and CH2O and water, respectively, from their deprotonated molecular ion. Although both NS- and NH-type ceramides showed the same fragmentation pattern in higher m/z regions of the tandem mass spectrum, these two ceramides were distinguished based on the m/z values of their deprotonated pseudomolecular ion. The MS/MS spectrum of NdS-type ceramides showed a series of fragment ion masses with differences of 18, 32, and 48 Da, which originated from the loss of water, methanol, and CH2O and water, respectively, from their deprotonated molecular ion. The MS/MS spectrum of NP-type ceramides also resulted in characteristic fragment ion masses with differences of 18, 32, and 36 Da, which originated from the loss of water, CH2O group, and two water molecules, respectively, from their deprotonated molecular ion. Based on these characteristic and general MS/MS fragmentation patterns, we determined the sphingoid backbone of N-type ceramides. These characteristic fragmentations of N-type ceramides were also confirmed on N-type ceramides having different acyl chain (Fig. 4). This pattern may be used to identify the sphingoid backbone of N-type ceramides in the SC of human, rat, and mouse skin.
Identification of N-type ceramides in the stratum corneum of human skin
Direct infusion of lipid extracts into a tandem mass spectrometer has been used for ceramide analysis in various sample sources such as bovine brain [2, 13], rat [24], and Jurkat T cell [25]. At this time, this direct infusion method has not been applied to ceramide profiling in skin SC.
In this study, the chip-based direct infusion nanoelectrospray-ion trap mass spectrometry was successfully applied to identify N-type ceramides in the human SC. A typical mass spectrum of human SC extracts is shown in Fig. 5. MS/MS/MS spectra were obtained from the product ion scan of the acetate adduct ion [M + CH3COO]−. Based on established mass fragmentation patterns of N-type ceramides, several N-type ceramides in human SC were identified (Tables 1, 2, 3, and 4). Analyzing the fragments revealed N-type ceramide molecules that differed in sphingosine and amide-linked fatty acid chain lengths. For example, a NS-type ceramide species with a monoisotopic mass of 708.7 Da (42 carbon atoms) contained both the expected and most abundant ratio N24/S18, as well as N26/S16, N25/S17, and N22/S20. Furthermore, several subspecies were detected with an odd number of carbon atoms in both chains, including N25/S17, N19/P17, N19/P19, N23/P17, N25/P17, and N23/H17. This observation agrees with a previously report by Hinder et al. [14].
An overview of the total carbon chain lengths of all N-type ceramide subclasses is listed in Fig. 6. The calculated total chain length distribution (Tables 1, 2, 3, and 4) of N-type ceramides (excluding NdS-type ceramides) is in agreement with a previous report by van Smeden et al. [26], t’Kindt et al. [2], and Vietzke et al. [10]. The relative distribution of NS-type ceramide (C40 to C52) in Korean SC (Fig. 6a) is similar to that in Belgian SC [2]. NP-type ceramides with a total number of carbon atoms between C33 and C51 (Fig. 6b) and NH type ceramides between C33 and C53 were detected (Fig. 6c). This is in line with a previous study [2], in which NP-type ceramides were found with 34–52 carbon atoms and NH-type ceramides between C32 and C52. These slight differences may originate from inter-individual variations [14]. NdS- and NH-type ceramides are of identical molecular weight; therefore, they produced the same m/z ion in mass spectra (Fig. 6d). In general, NH-type ceramide is more abundant than NdS-type ceramide [2], and the intensity of NH-type specific fragment ions is stronger than that of NdS-type specific fragment ions in the product ion spectra, which resulted in NH-type ceramide identification compared to NdS-type ceramide. Based on these results, it is possible that NdS-type ceramide profiling in human SC using direct infusion electrospray ion trap mass spectrometry may not be possible because of the presence of abundant NH-type ceramide.
Conclusion
In this study, the molecular structures of the subspecies of the four N-type ceramide classes (NS-, NdS-, NP-, and NH-type) were analyzed using chip-based direct infusion nanoelectrospray-ion trap mass spectrometry. The product ions of native N-type ceramides were associated with fragments of the precursors using the fragmentation schemes derived from tandem mass spectra of N-type ceramides. Based on the MS/MS fragmentation pattern of these ceramides, an N-type ceramide MS/MS library was generated. We also applied this N-type ceramide MS/MS library to determine the exact structure of N-type ceramides extracted from human SC. In contrast to the relatively simple ceramide standards, ceramides of the SC require intensive investigation. However, this method could be used to identify N-type ceramides in human SC.
Structural information of N-type ceramide molecular species, including fatty acyl amide substituents and sphingoid moieties, were easily obtained using negative-ion nanoelectrospray tandem mass spectrometry. This platform could be a useful tracking biomarker for various skin diseases such as allergic contact dermatitis and atopic dermatitis.
References
Masukawa Y, Narita H, Shimizu E, Kondo N, Sugai Y, Oba T, Homma R, Ishikawa J, Takagi Y, Kitahara T, Takema Y, Kita K (2008) Characterization of overall ceramide species in human stratum corneum. J Lipid Res 49(7):1466–1476. doi:10.1194/jlr.M800014-JLR200
t’Kindt R, Jorge L, Dumont E, Couturon P, David F, Sandra P, Sandra K (2012) Profiling and characterizing skin ceramides using reversed-phase liquid chromatography-quadrupole time-of-flight mass spectrometry. Anal Chem 84(1):403–411. doi:10.1021/ac202646v
Weerheim A, Ponec M (2001) Determination of stratum corneum lipid profile by tape stripping in combination with high-performance thin-layer chromatography. Arch Dermatol Res 293(4):191–199
Mizutani Y, Mitsutake S, Tsuji K, Kihara A, Igarashi Y (2009) Ceramide biosynthesis in keratinocyte and its role in skin function. Biochimie 91(6):784–790. doi:10.1016/j.biochi.2009.04.001
Lampe MA, Burlingame AL, Whitney J, Williams ML, Brown BE, Roitman E, Elias PM (1983) Human stratum corneum lipids: characterization and regional variations. J Lipid Res 24(2):120–130
Holleran WM, Takagi Y, Uchida Y (2006) Epidermal sphingolipids: metabolism, function, and roles in skin disorders. FEBS Lett 580(23):5456–5466. doi:10.1016/j.febslet.2006.08.039
Motta S, Monti M, Sesana S, Caputo R, Carelli S, Ghidoni R (1993) Ceramide composition of the psoriatic scale. Biochim Biophys Acta 1182(2):147–151
Jungersted JM, Scheer H, Mempel M, Baurecht H, Cifuentes L, Hogh JK, Hellgren LI, Jemec GB, Agner T, Weidinger S (2010) Stratum corneum lipids, skin barrier function and filaggrin mutations in patients with atopic eczema. Allergy 65(7):911–918. doi:10.1111/j.1398-9995.2010.02326.x
Higuchi H, Nakamura M, Kuwano A, Kasamatsu M, Nagahata H (2005) Quantities and types of ceramides and their relationships to physical properties of the horn covering the claws of clinically normal cows and cows with subclinical laminitis. Can J Vet Res 69(2):155–158
Vietzke JP, Brandt O, Abeck D, Rapp C, Strassner M, Schreiner V, Hintze U (2001) Comparative investigation of human stratum corneum ceramides. Lipids 36(3):299–304
Sahle FF, Lange S, Dobner B, Wohlrab J, Neubert RH (2012) Development and validation of LC/ESI-MS method for the detection and quantification of exogenous ceramide NP in stratum corneum and other layers of the skin. J Pharm Biomed Anal 60:7–13. doi:10.1016/j.jpba.2011.10.032
Raith K, Neubert RHH (2000) Liquid chromatography–electrospray mass spectrometry and tandem mass spectrometry of ceramides. Anal Chim Acta 403:295–303. doi:10.1016/s0003-2670(99)00661-3
Hsu FF, Turk J (2002) Characterization of ceramides by low energy collisional-activated dissociation tandem mass spectrometry with negative-ion electrospray ionization. J Am Soc Mass Spectrom 13(5):558–570. doi:10.1016/S1044-0305(02)00358-6
Hinder A, Schmelzer CE, Rawlings AV, Neubert RH (2011) Investigation of the molecular structure of the human stratum corneum ceramides [NP] and [EOS] by mass spectrometry. Skin Pharmacol Physiol 24(3):127–135. doi:10.1159/000322303
Farwanah H, Pierstorff B, Schmelzer CE, Raith K, Neubert RH, Kolter T, Sandhoff K (2007) Separation and mass spectrometric characterization of covalently bound skin ceramides using LC/APCI-MS and Nano-ESI-MS/MS. J Chromatogr B Anal Technol Biomed Life Sci 852(1–2):562–570. doi:10.1016/j.jchromb.2007.02.030
Zhang J, Yan L, Chen W, Lin L, Song X, Yan X, Hang W, Huang B (2009) Metabonomics research of diabetic nephropathy and type 2 diabetes mellitus based on UPLC-oaTOF-MS system. Anal Chim Acta 650(1):16–22. doi:10.1016/j.aca.2009.02.027
Wang Y, Zhang H (2011) Tracking phospholipid profiling of muscle from Ctennopharyngodon idellus during storage by shotgun lipidomics. J Agric Food Chem 59(21):11635–11642. doi:10.1021/jf2030852
Shen Q, Wang Y, Gong L, Guo R, Dong W, Cheung HY (2012) Shotgun lipidomics strategy for fast analysis of phospholipids in fisheries waste and its potential in species differentiation. J Agric Food Chem 60(37):9384–9393. doi:10.1021/jf303181s
Schuhmann K, Herzog R, Schwudke D, Metelmann-Strupat W, Bornstein SR, Shevchenko A (2011) Bottom-up shotgun lipidomics by higher energy collisional dissociation on LTQ Orbitrap mass spectrometers. Anal Chem 83(14):5480–5487. doi:10.1021/ac102505f
Heiskanen LA, Suoniemi M, Ta HX, Tarasov K, Ekroos K (2013) Long-term performance and stability of molecular shotgun lipidomic analysis of human plasma samples. Anal Chem. doi:10.1021/ac401857a
Han X (2002) Characterization and direct quantitation of ceramide molecular species from lipid extracts of biological samples by electrospray ionization tandem mass spectrometry. Anal Biochem 302(2):199–212. doi:10.1006/abio.2001.5536
Raith K, Neubert RHH (1998) Structural studies on ceramides by electrospray tandem mass spectrometry. Rapid Comm Mass Spectrom 12:935–938. doi:10.1002/(sici)1097-0231(19980731)12:14<935::aid-rcm260>3.0.co;2-u
Bonté F, Saunois A, Pinguet P, Meybeck A (1997) Existence of a lipid gradient in the upper stratum corneum and its possible biological significance. Arch Dermatol Res 289(2):78–82
Jiang X, Cheng H, Yang K, Gross RW, Han X (2007) Alkaline methanolysis of lipid extracts extends shotgun lipidomics analyses to the low-abundance regime of cellular sphingolipids. Anal Biochem 371(2):135–145. doi:10.1016/j.ab.2007.08.019
Gu M, Kerwin JL, Watts JD, Aebersold R (1997) Ceramide profiling of complex lipid mixtures by electrospray ionization mass spectrometry. Anal Biochem 244(2):347–356. doi:10.1006/abio.1996.9915
van Smeden J, Hoppel L, van der Heijden R, Hankemeier T, Vreeken RJ, Bouwstra JA (2011) LC/MS analysis of stratum corneum lipids: ceramide profiling and discovery. J Lipid Res 52(6):1211–1221. doi:10.1194/jlr.M014456
Acknowledgments
This work was supported by the Korea Healthcare Technology R&D Project, Ministry of Health and Welfare (grant A103017), Republic of Korea.
Author information
Authors and Affiliations
Corresponding authors
Rights and permissions
About this article
Cite this article
Shin, JH., Shon, J.C., Lee, K. et al. A lipidomic platform establishment for structural identification of skin ceramides with non-hydroxyacyl chains. Anal Bioanal Chem 406, 1917–1932 (2014). https://doi.org/10.1007/s00216-013-7601-y
Received:
Revised:
Accepted:
Published:
Issue Date:
DOI: https://doi.org/10.1007/s00216-013-7601-y