Abstract
The fatty acid methyl ester (FAME) content of biodiesel fuels has traditionally been determined using gas chromatography with a polar stationary phase. In this study, a direct comparison of the separation of FAMEs present in various biodiesel samples on three polar stationary phases and one moderately polar stationary phase (with comparable column dimensions) was performed. Retention on each column was based on solubility in and polarity of the phase. Quantitative metrics describing the resolution of important FAME pairs indicate high resolution on all polar columns, yet the best resolution, particularly of geometric isomers, is achieved on the cyanopropyl column. In addition, the separation of four C18 monounsaturated isomers was optimized and the elution order determined on each column. FAME composition of various biodiesel fuel types was determined on each column to illustrate (1) chemical differences in biodiesels produced from different feedstocks and (2) chemical similarities in biodiesels of the same feedstock type produced in different locations and harvest seasons.
Similar content being viewed by others
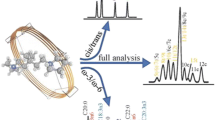
Avoid common mistakes on your manuscript.
Introduction
Renewable and sustainable biodiesel fuels are emerging as an alternative energy source in many parts of the world. Biodiesel is produced through the transesterification of fatty acids in vegetable oil or animal fat to produce the corresponding fatty acid methyl esters (FAMEs). Both the fuel quality and efficiency are dependent on the FAME content, which is in turn largely dependent on the starting material, or feedstock [1–3]. A variety of feedstocks have been used in biodiesel production, including vegetable oils (e.g., soybean, canola, coconut), animal fats (e.g., tallow), and waste oils. Each type of feedstock contains a unique fatty acid profile with varying chain length and saturation, thus leading to a unique FAME profile in the biodiesel. As such, there is a specific need for a set of conditions that can be used to elucidate adequate separation of a range of FAMEs in a variety of biodiesels. Development of a standard set of separation conditions would allow generation of unique fingerprints for direct comparison of biodiesels from different feedstocks.
Several analytical methods have been used to characterize the chemical components of biodiesel [1, 4]. The United States (ASTM 7398–11, D 6751) and Europe (EN 14214) have set standards based on gas chromatography (GC) with flame ionization detection (FID) for separation of FAMEs in biodiesel based on boiling point. In addition, GC-FID and GC-MS have been used to demonstrate that the relative concentration of FAMEs in biodiesel varies between feedstocks [5–16]. Other analysis methods such as infrared spectroscopy [17], liquid chromatography with spectroscopic [18, 19] and evaporative light-scattering detection [18], as well as supercritical fluid chromatography [20], and two-dimensional gas chromatography (GC × GC) with FID and time of flight mass spectrometry have been used to analyze biodiesel and biodiesel–diesel blended fuels [21–25]. A review of chromatographic techniques used in the analysis of biodiesel and biodiesel blends was published in 2011 [26]. While chromatography is the clear choice for the analysis of FAMEs, no study has compared column chemistry and polarity to determine ideal conditions to isolate all FAMEs present in biodiesel fuels.
Several researchers have evaluated GC for the separation of fatty acids either in standard solutions or in other complex matrices following derivatization to their methyl esters. Recently, ionic liquids have been used successfully for the separation of fatty acids in varying matrices [27–29], yet traditional GC phases have been more commonly used for routine analysis. For example, Stauffer and Byron evaluated a weakly polar phase (5 % phenyl-modified polydimethylsiloxane), for the separation of FAMEs in a biodiesel, and discovered that the order of elution was dependent on chain length (C18 follows C16), as well as degree of unsaturation (C18 follows C18:1) [6]. However, isomers of the same chain length were not separated on this weakly polar column. Yamamoto et al. investigated more moderately polar columns (50 % phenyl PDMS) for the separation of fatty acid standards and found that the order of elution was dependent on chain length (C18 follows C16), as well as degree of unsaturation (yet C18:1 now follows C18) [30]. Most isomeric unsaturated FAMEs were not separated from one another yet C18:1n9 and C18:1n11 were moderately separated, showing that increasing the polarity of the column increases the ability to separate unsaturated isomers. Others have shown that the use of a highly polar column, such as polyethylene glycol or cyanopropyl-modified polydimethylsiloxane, allowed for increased separation of isomeric unsaturated FAME mixtures [31–36]. Furthermore, Harynuk et al. evaluated the degree of modification of cyanopropyl phases using a FAME standard [33]. Interestingly, the elution order of isomeric unsaturated FAMEs was highly dependent on the degree of modification of the column (60–90 % cyanopropyl), yet could be separated on all phases [33]. In addition, a review of fast gas chromatographic techniques indicated that the separation of FAMEs has been typically based on polar column chemistries and could be completed in less than 5 min [37]. However, in each of these evaluations of the lipid sources, the column dimensions and oven conditions varied from study to study. In addition, a direct comparison of the column choice for the separation of FAMEs in biodiesels specifically has not been performed. Thus, a direct comprehensive comparison of stationary phases used for the analysis of the FAMEs present in typical biodiesel fuels is needed.
In this study, four stationary phase chemistries with comparable column dimensions were evaluated and compared for the analysis of FAMEs in a variety of biodiesel feedstocks, including soy, canola, tallow, and waste grease, obtained from various locations over a several year span. The separation of the FAMEs present in biodiesel fuels was optimized on each phase using comparable oven conditions, with special attention directed towards the separation of C18 monounsaturated isomers (both structural and geometric) to determine elution order and resolution of key pairs of FAMEs. Quantitative metrics describing the resolution of important FAME pairs are provided. The selection of a standard column for fingerprinting of FAMEs in a variety of biodiesel samples will be discussed, as well as the information that can be gleaned from the C18 isomer region of the chromatogram.
Materials and methods
Chemicals
Standard solutions of fatty acid methyl esters and biodiesels (FAME-37, trans-9, cis-9, trans-11, and cis-11 C18 isomers, Supelco, Bellefonte, PA; SRM 2377, NIST, Gaithersburg, MD) were stored at −20 °C and used without further preparation. Biodiesel fuel samples were obtained from various manufacturers throughout the United States (Minnesota Soybean Processors (soybean), Western Dubuque Biodiesel (soybean), Iowa Renewable Energy (soybean, tallow), NIST (SRM 2772, soybean from Ag Processing Inc), ADM Company (canola), TMT Biofuels (waste grease), and Texas Green Manufacturing (beef tallow)) and stored in their original shipping container at 4 °C. Prior to dilution, each biodiesel was gradually warmed to room temperature and inverted multiple times to ensure homogeneity. One milliliter of each biodiesel sample was diluted to 100 mL total volume with methylene chloride (BDH distributed by VWR, West Chester, PA). One milliliter of 0.30 M tridecanoic acid methyl ester (internal standard) was added to a 50-mL volumetric flask and diluted to volume with the 100:1 biodiesel. Tridecanoic acid methyl ester was chosen as an internal standard as it was not present in any of the biodiesel samples originally. All diluted biodiesel solutions were stored in brown bottles at 4 °C and gradually warmed to room temperature prior to analysis.
Instrumentation
Separations were performed using an Agilent 6890 gas chromatograph coupled with an Agilent 5937 mass spectrometer (Agilent Technologies, Santa Clara, CA). The GC-MS was equipped with one of four fused-silica capillary columns of dimensions 30 m × 0.25 mm × 0.25 μm (polyethylene glycol (ZB-WAXplus, Phenomenex), nitroterephthalic acid-modified polyethylene glycol (ZB-FFAP, Phenomenex), 70 % cyanopropyl-modified polydimethylsiloxane (BPX70, SGE Analytical Science), 35 % phenyl-modified polydimethylsiloxane (ZB-35, Phenomenex)). The oven temperature was optimized for each column as follows: ZB-WAXplus and ZB-FFAP −60 °C (hold 2 min) to 150 °C at 13 °C/min to 230 °C at 2 °C/min; BPX70 −60 to 150 °C at 13 °C/min to 230 °C at 1 °C/min; ZB-35 −60 to 150 °C at 13 °C/min to 190 °C at 1 °C/min to 270 °C at 5 °C/min.
High purity helium was used as a carrier gas at a nominal flow rate of 1.5 mL/min (ZB-WAXplus, ZB-FFAP, ZB-35) or 1.0 mL/min (BPX70). Each sample was injected via syringe (1 μL, Hamilton Company) with a split ratio of 50:1 (ZB-WAXplus, ZB-FFAP, ZB-35) or 100:1 (BPX70). C18:1 isomer standards were injected via syringe (0.2 μL) with a split ratio of 200:1. The inlet and transfer line temperatures were held at 250 and 280 °C, respectively. An electron-impact ionization source was utilized with a quadrupole mass analyzer operated in full-scan mode (m/z 20–300) with a sampling rate of 4.94 scans/s. The mass spectrometer source and quadrupole were held at 230 °C and 150 °C, respectively. FAME identification was performed using the mass spectra library (NIST mass spectral search program version 2.0a, Gaithersburg, MD) as well as retention time comparison to the FAME standards. Retention factors and peak resolutions were calculated in Microsoft Excel 2007. The peak area of each FAME in a sample was summed and the percentage that each FAME contributed to the total area was calculated as a peak area percentage.
Results and discussion
Comparison of column chemistry
Analysis of FAME standards
A standard solution of 37 FAMEs was used to optimize the temperature program on each column. Optimized conditions were established when the majority (if not all) of the FAMEs were baseline-resolved. The chromatograms resulting from the optimized temperature programs are shown in Fig. 1. The ZB-WAXplus and ZB-FFAP columns allowed for nearly baseline separation of 36 of the 37 FAMEs contained in the standard (Fig. 1a and b, respectively). The C18:1n9 cis/trans isomers (C18:1n9t and C18:1n9c) coelute on both phases. The BPX70 column allowed for baseline separation of all 37 FAMEs (Fig. 1c). The extent of retention on polar columns is dependent on both polarity and solubility in the phase. Short chain, nonpolar solutes are less soluble in the phase and elute quickly, while longer chain, polar solutes interact with the phase longer and are more retained. That is, saturated FAMEs elute based on carbon number. The elution order of the unsaturated FAMEs is more interesting. Mono-, di-, and some tri-unsaturated FAMEs elute prior to the next saturated FAME in the sequence, however other polyunsaturated FAMEs elute after the next saturated FAME in the sequence. For example, C20:1, C20:2, and C20:3n6 elute prior to C21, yet C20:3n3, C20:4, and C20:5 elute after C21. The elution order is comparable on the ZB-WAXplus and ZB-FFAP columns, as their column chemistries are similar (both are based on polyethylene glycol). The elution order on the cyanopropyl column (BPX70) varies from that of the polyethylene glycol phases. Most notably, the polyunsaturated C20:3 and C20:5 elute after the saturated C21 and C22, respectively. The difference in the elution order is heavily dependent on the column polarity. The cyanopropyl column is more polar than either of the polyethylene glycol phases, and thus the more polar FAMEs become more retained on the cyanopropyl phase.
Chromatograms showing separation of 37 component FAME standard (Supelco) on ZB-WAXplus (a), ZB-FFAP (b), BPX70 (c), and ZB-35 (d). The detailed elution order for the ZB-35 column is as follows: C18 region—C18:1n9c, C18:3n6 and C18:1n9t, C18 and C18:2, C18:2, C18:3n3; C20 region—C20:4, C20:1, C20:3n6, C20 and C20:2, C20:5, C20:3n3; C22 region—C22:1, C22 and C22:2, C22:6. The insets show separation of four FAME C18:1 isomers on each column (see text for more detail). Solutes: dashed line trans-9, solid line trans-11, dotted line cis-9, dash–dot line cis-11
It was strikingly more difficult to establish the optimum separation with the moderately polar column ZB-35, as compared to the ease of separation of isomers with the polar columns. For instance, several different variations of temperature programs were examined for the ZB-35 column. While some programs were ideal for separating the later eluting FAMEs (i.e., C20 and C22), others were better for the earliest eluting FAMEs (i.e., C4–C15). However, there were no conditions that were optimal for all FAMEs in the standard, and thus, the temperature program that separated the greatest number of FAMEs was utilized. This program, specified in the materials section, allowed for separation of 32 of the 37 FAMEs (Fig. 1d). The elution order on ZB-35 is different than on the previous three columns, as expected based on its polarity. While the ZB-35 column emanates both nonpolar and polar character, the extent of retention is still based on both features. Similar to the polar columns, saturated FAMEs elute based on carbon number. However, all unsaturated forms of the parent saturated FAME elute prior to the next larger saturated FAME. For instance, all C20 isomers elute prior to C21. The result is detrimental overlap of peaks that were clearly separated on the polar columns, particularly in the C18 and C20 region. In addition, interesting differences in the elution order of the isomers are present. While monounsaturated C14:1 and C15:1 elute directly after their own saturated FAME, monounsaturated C16:1 and C17:1 elute before the saturated version, and for larger FAMEs, mono- and di-unsaturated isomers elute prior to their parent FAME. Thus, retention on this phase is a combination of interactions due to polarity and solubility, with a change in the dominant effect for a given FAME throughout the separation.
Resolution values for key pairs of FAMEs on each column are provided in Table 1. For all pairs, the resolution is greatest on the BPX70 column. For all pairs that are not baseline-resolved on ZB-WAXplus and ZB-FFAP (save the monounsaturated C18 isomers), the resolution is still adequate for qualitative analysis (greater than 1.0). If the same pairs are examined with respect to the ZB-35 column, it appears that the resolution is adequate. Yet, there are many instances in which baseline resolution is not achieved. In fact, there are several cases where there is complete overlap (e.g., C18:3–C18:1 and C22–C22:2). To summarize the resolutions obtained on each column, mean resolution values for several sets of pairs are included in Table 1. The average resolution for the key pairs of FAMEs (identified in Table 1) indicate adequate resolution for all columns, yet higher values (and thus better resolution) for the most polar columns. When the pairs for the five lowest resolution values on each column are averaged, it is apparent that the ZB-35 column provides inadequate separation for a number of FAME pairs, and that the ZB-Waxplus and ZB-FFAP columns contain pairs with resolution values below 1.5 (indicating pairs that are not baseline-resolved). In fact, the number of pairs with resolution below 1.5 decreases as the column polarity increases (Table 1). In addition, the average resolution of the C18 isomers in the standard mixture increases as the polarity of the column is increased, again showing the versatility of the BPX column for the separation of a greater number of FAMEs.
Analysis of biodiesel samples
A set of biodiesels was analyzed utilizing the optimum temperature programs developed for the FAME standard. The soybean biodiesel is the most simple chemically, as there are six prevalent FAMEs ranging from C16 to C18:3. All peaks are baseline-resolved on each of the polar columns. The peak areas for the FAMEs present in the soybean biodiesel are similar from one polar column to the next, with low relative standard deviations across columns, showing no bias in terms of column selection if a polar column is utilized (Table 2). Similar results were obtained for other simple samples (e.g., waste grease). The canola and tallow biodiesels are more chemically complex, as they contain a larger number of FAMEs and a higher concentration of the C18 and C18:1 isomers. Due to this complexity, some of the peaks in the C18 region were not well resolved using ZB-WAXplus and ZB-FFAP, yet were baseline-resolved on BPX70. In addition, many biodiesel samples contain C18, C18:1, and C18:3. Due to the overlap in this region on the ZB-35 column, complete separation is not achieved even with the simple chemical nature of soybean biodiesel.
To further understand the effect of the column polarity on the separation of unsaturated isomers, four C18:1 isomer standards were injected individually to determine elution order. These C18:1 isomers are commonly found in biodiesel samples and their identity may help provide a useful fingerprint for each biodiesel feedstock. The chromatographic region where the C18:1 isomers elute on each column are shown in the insets of Fig. 1. On both the ZB-WAXplus and the ZB-FFAP columns, the C18:1n9 isomers elute prior to the C18:1n11 isomers (Fig. 1a and b, respectively). There is slight separation between the trans and cis C18:1n9, however it would likely be difficult to perform this separation in a biodiesel sample. On ZB-35, the cis versions of each isomer elute before the trans versions, however, there is overlap between the C18:1n11c and C18:1n9t (Fig. 1d). There is surprisingly adequate separation of these isomers, however, as mentioned previously many of the C18 compounds elute in this region, and coelution is major problem on this column. Thus, if the goal of the separation is to determine isomeric content of FAMEs in a sample, these three columns may not be ideal. However, on the BPX70 column, there is clear separation between the four isomers with the trans versions of each isomer eluting before the cis versions and in each set the C18:1n9 elutes before the C18:1n11 (Fig. 1c). The increased polarity of the cyanopropyl column allows for further discrimination of geometric and structural isomers. Thus, for clear discrimination of the FAME isomers present in a sample, the BPX 70 column would be the ideal choice. Thus, for all further analyses, only the BPX70 column will be discussed.
Comparison of biodiesel samples
Representative chromatograms for soybean, tallow, canola, and waste grease biodiesels separated on the BPX70 column are shown in Fig. 2, with identification of each FAME along with peak area percent displayed in Table 3. The FAME composition in these four biodiesel samples varies considerably from feedstock to feedstock. For example, while the C18:2 is the most prevalent FAME in the soybean biodiesel, it is one of the lowest contributors to the tallow biodiesel. In fact, the tallow has many FAMEs (the most of all feedstocks tested), yet the most prevalent are the C16, C18, and C18:1n9. There are several FAMEs present in low concentration in the tallow, canola, and waste grease biodiesels, contributing less than 2 % each to the overall FAME profile. Yet the FAMEs in low concentration are not consistent from feedstock to feedstock. The fingerprints generated from the BPX70 column nicely display the inherent differences in FAME composition of the biodiesel fuels.
Chromatograms showing separation of FAMEs in soybean-based biodiesel (a), tallow-based biodiesel (b), canola-based biodiesel (c), and waste grease-based biodiesel (d) on BPX70 column. Peak identification is provided in Table 3. *Internal standard—C13 FAME
The largest benefit of using the BPX70 column is the resolution of the monounsaturated C18 isomers. This clear separation allowed for an unidentified C18:1 isomer to be recognized in one of the tallow samples (Fig. 3a). This isomer is quite difficult to distinguish on the other columns (shown in Fig. 3b and c) as the resolution of the C18:1 isomers is inadequate. This isomer is not present in the same concentration in the other tallow samples in the study including a sample from the same company taken from a different year (present but in a lower abundance, not shown) as well as a tallow from a different manufacturer (not present in sample, Fig. 3d), yielding evidence towards the beneficial use of the cyanopropyl stationary phase to distinguish biodiesel samples.
While it has been known that the feedstock type can greatly influence the FAME profile, there is little information regarding how variable the FAME content can be from region to region or even from year to year. The peak area percents for all soybean biodiesels used in this study are tabulated in Table 4. Samples taken from the same manufacturer 1 year apart (Minnesota soy) are consistent in chemical composition. In addition, samples taken from manufacturers in different states (Minnesota and Iowa) or within the same state (Iowa soy, IRE soy, Soy SRM) have consistent chemical composition. The relative standard deviation of each FAME ranges from 0.04 to 0.2 indicating consistency across samples taken from different regions over different time frames. These data validate the idea that biodiesel composition is mostly consistent within same feedstock type if a plant-based feedstock is used.
Summary
In this study, GC column chemistry and polarity were compared to determine optimal conditions for isolating FAMEs present in biodiesel fuels. To develop the method on each column, a complex FAME standard was utilized. Elution order and resolution of the FAMEs was highly dependent on the column chemistry. Yet, baseline resolution of all 37 components in the FAME standard was achieved using a cyanopropyl column. Quantitative metrics describing the resolution of important FAME pairs show the versatility of the polar columns for the separation of FAMEs, and the importance of the cyanopropyl column for the separation of isomeric FAME pairs. A strong emphasis was placed on the separation of four C18 monounsaturated isomers; only the cyanopropyl column allowed for complete separation of these isomers. Thus, adequate qualitative analysis could be performed with any of the polar columns used in this study, however quantitative analysis, specifically of the monounsaturated C18 isomers, could only be performed using the cyanopropyl column. Finally, using these standard separation conditions, a unique fingerprint for each biodiesel was generated. Using these fingerprints, the chemical composition of a variety of biodiesel fuels was determined. The composition of FAMEs in each biodiesel was found to depend greatly on the feedstock type yet less so on the origin of the feedstock or the year of processing. Additional work with chemometric methods of analysis is ongoing in the lab to further investigate the importance of column choice and feedstock type.
References
Knothe G, Van Gerpen J, Krahl J (eds) (2005) The biodiesel handbook. AOCS, Champaign, IL
Knothe G (2008) Energ Fuel 22:1358–1364
Moser BR (2009) In Vitro Cell Dev Biol Plant 45:229–266
Knothe G (2006) JAOCS 83:823–833
Schober S, Seidl I, Mittelbach M (2006) Eur J Lipid Sci Technol 108:309–314
Stauffer E, Byron D (2007) J Forensic Sci 52:371–379
Freedman B, Kwolek WF, Pryde EH (1986) JAOCS 63:1370–1375
Fan H, Wang B, Yun Z (2012) Energy Sources Part A 34:2084–2091
Ruiz-Méndez MV, Marmesat S, Liotta A, Dobarganes MC (2008) Grasas Y Aceites 59:45–50
Moser BR, Vaughn SF (2010) Bioresour Technol 101:646–653
Pardo VL, Fagundes CAM, Caldas SS, Kurz MH, Clementin RM, D’Oca MGM, Primel EG (2012) JAOCS 89:631–637
Koria L, Nithya G (2012) Journal of Phytology 4:6–9
Jham GN, Moser BR, Shah SN, Holser RA, Dhingra OD, Vaughn SF, Berhow MA, Winkler-Moser JK, Isbell TA, Holloway RK, Walter EL, Natalino R, Anderson JC, Stelly DM (2009) JAOCS 86:917–926
Moser BM, Knothe G, Vaughn SF, Isbell TA (2009) Energ Fuel 23:4149–4155
Poerschmann J, Parsi Z, Górecki T, Augustin J (2005) J Chromatogr A 1071:99–109
Pauls RE (2011) J Chromatograph Sci 49:370–374
Zhang W-B (2012) Renewable & Sustainable Energy Reviews 16:6048–6058
Foglia TA, Jones KC, Phillips JG (2005) Chromatographia 62:115–119
NicolaG D, Pacetti M, Polonara F, Santori G, Stryjek R (2008) J Chromatogr A 1190:120–126
Cole J, Lefler J, Chen R (2008) LC-GC Europe 44–46
Seeley JV, Seeley SK, Libby EK, McCurry JD (2007) J Chromatogr Sci 45:650–656
Adam F, Bertoncini F, Coupard V, Charon N, Thiébaut D, Espinat D, Hennion M-C (2008) J Chromatogr A 1186:236–244
Tiyapongpattana W, Wilairat P, Marriott PJ (2008) J Sep Sci 31:2640–2649
Hejazi L, Ebrahimi D, Guilhaus M, Hibbert DB (2009) Anal Chem 81:1450–1458
Seeley JV, Bates CT, McCurry JD, Seeley SK (2012) J Chromatogr A 1226:103–109
Pauls RE (2011) J Chromatograph Sci 49:384–396
Anderson JL, Armstrong DW (2005) Anal Chem 77:6453–6462
Ragonese C, Tranchida PQ, Dugo P, Dugo G, Sidisky LM, Robillard MV, Mondello L (2009) Anal Chem 81:5561–5568
Ragonese C, Tranchida PQ, Sciarrone D, Mondello L (2009) J Chromatogr A 1216:8992–8997
Yamamoto K, Kinoshita A, Shibahara A (2008) J Chromatogr A 1182:132–135
Mondello L, Casilli A, Tranchida PQ, Costa R, Chiofalo B, Dugo P, Dugo G (2004) J Chromatogr A 1035:237–247
Ratnayake WMN, Hansen SL, Kennedy MP (2006) JAOCS 83:475–488
Harynuk J, Wynne PM, Marriott PJ (2006) Chromatographia 63:S61–S66
Delmonte P, Rader JI (2007) Anal Bioanal Chem 389:77–85
Kiridena W, Qian J, Koziol WW, Poole CF (2007) J Sep Sci 30:740–745
Vickers AK (2007) Am Lab 39:18–20
Cruz-Hernandez C, Destaillats F (2009) J of Liq Chromatogr Rel Technol 32:1672–1688
Acknowledgments
The authors are greatly appreciative of financial support provided by the O’Brien Family (JCG and DYR) and Jacqueline and George Paletta, Jr. (SJB) Summer Research Fellowships, the University Syringe Program Grant from Hamilton Company, the Robert L. Ardizzone Fund for Junior Faculty Excellence (AMH), and the College of the Holy Cross. In addition, the authors thank the National Institute of Standards and Technology (NIST, Gaithersburg, MD) for supplying the FAME standards and Minnesota Soybean Processors, Western Dubuque Biodiesel, ADM Company, TMT Biofuels, Texas Green Manufacturing, and Iowa Renewable Energy for donating biodiesel samples.
Author information
Authors and Affiliations
Corresponding author
Rights and permissions
About this article
Cite this article
Goding, J.C., Ragon, D.Y., O’Connor, J.B. et al. Comparison of GC stationary phases for the separation of fatty acid methyl esters in biodiesel fuels. Anal Bioanal Chem 405, 6087–6094 (2013). https://doi.org/10.1007/s00216-013-7042-7
Received:
Revised:
Accepted:
Published:
Issue Date:
DOI: https://doi.org/10.1007/s00216-013-7042-7