Abstract
The ceramides of the stratum corneum are critical to maintaining the epidermal barrier function of the skin. A number of skin diseases and disorders are known to be related to impairments of the ceramide pattern. Therefore, obtaining mass spectrometric profiles of the nine ceramide classes known to exist aids our understanding of the underlying molecular mechanisms, which should eventually lead to new diagnostic opportunities: for example, the mass spectrometric profiles of patients suffering from serious skin diseases such as atopic dermatitis and psoriasis can be compared to those of healthy controls. Previous work on mass spectrometric analysis of ceramides relied mostly on GC/MS after hydrolysis and derivatization. The introduction of ESI–MS and LC/ESI–MS has provided new options for directly analyzing intact ceramides. However, some of the ceramide classes are not accessible to ESI–MS. However, as shown in this work, these limitations of GC/MS and ESI-MS can be overcome using a new approach based on normal phase LC interfaced with APCI–MS. Separation and online detection of the stratum corneum ceramide classes became possible in one run. Ceramide species with C26 and/or C28 fatty acid chains were the most abundant ones in Cer [NP], Cer [NH], Cer [AP], and Cer [AH]. The main component of Cer [AS] was C16. The ω-esterified ceramide classes Cer [EOS], Cer [EOP] and Cer [EOH] contained mostly species with fatty acids >C30. This was also the case for Cer [NS], suggesting an analogy to the ω-esterified ceramides. In addition, evidence for a new ceramide class Cer [NdS] was found.
Similar content being viewed by others
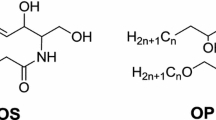
Avoid common mistakes on your manuscript.
Introduction
The stratum corneum (SC), the outermost layer of the skin, is the location of the epidermal barrier function. It consists of corneocytes, which are embedded in a complex lipid matrix consisting mainly of ceramides, cholesterol and free fatty acids [1–3].
Among the lipids in the stratum corneum, ceramides play a key role in maintaining the skin's water permeability barrier [2, 4]. A deficiency of key enzymes (such as β-glucocerebrosidase in Gaucher-type-2 disease) leads to impairments in the epidermal barrier function [5, 6]. In addition, skin diseases such as atopic dermatitis and psoriasis are linked to alterations in ceramide profiles [7–12]. Therefore, ceramides provide the focus of much important dermatological and cosmetic research.
Ceramides are sphingolipids, which consist of sphingoid bases amide-linked to fatty acids. Three types of sphingoid bases, namely sphingosine (S), phytosphingosine (P), and 6-hydroxysphingosine (H) have been found in SC ceramides. The amide-bound fatty acids can be α-hydroxylated (A) or nonhydroxylated (N). ω-Hydroxy fatty acids also occur, which are esterified with another fatty acid (EO), which is usually linolic acid. Combining the three types of the sphingoid bases with the three types of fatty acids results in nine ceramide classes: Cer [EOS], Cer [NS], Cer [EOP], Cer [NP], Cer [EOH], Cer [NH], Cer [AS], Cer [AP], and Cer [NH] (this nomenclature for the ceramide classes was proposed by Motta et al. [8] and Robson et al. [13]).
Each of the ceramide classes contains various species that result from varying the chain lengths of the sphingoid bases and the amide-linked fatty acids. The most common chain length for the sphingoid bases is C18 [2]. The chain lengths of the amide-bound fatty acids vary between C16 and C40 [2]. Figure 1 shows the structural formulae of the ceramide classes mentioned above.
Common methods of collecting skin lipids, and particularly ceramides, include biopsies, tape stripping, cyanoacrylate stripping and topical solvent extraction [14–16]. The latter has proven to be a useful tool in relation to the efficiency of extraction and the compliance of test persons, provided that suitable solvent mixtures are used.
Thin-layer chromatography (TLC) has long been the method of choice for analyzing SC ceramide classes. A number of procedures enable the separation of at least seven ceramide classes [16, 17]. Because of the similar polarities of the structural isomers Cer [AS] and Cer [NH], their separation can be achieved after acetylation [18, 19]. The ninth ceramide class (Cer [EOP]) was discovered only recently [18]. In order to overcome the disadvantages of manual TLC procedures, which are laborious and time- and solvent-consuming, new high-performance thin-layer chromatography methods with automated multiple development (AMD-HPTLC) have been developed [20–22].
Gas chromatography/mass spectrometry (GC/MS) has been previously used to analyze the molecular profiles of the ceramide classes present in the SC [23, 24]. Here, the experimental steps include separating the ceramides via TLC, sampling the bands of interest, extracting and hydrolyzing the corresponding ceramides, and then derivatizing and analyzing the resulting sphingoid bases and fatty acids separately. In addition to the tedious handling, GC/MS does not provide information on the possible combinations of both moieties.
Profiling and detailed characterizations of species within most of the ceramide classes have been performed via ESI–MS, ESI–MS/MS and LC/ESI–MS, without the need for an initial hydrolysis step [25–28]. However, important ceramides with a ω-ester linkage, such as Cer [EOS], Cer [EOP] and Cer [EOH], were not accessible to ESI–MS.
Recently, a new normal phase HPLC/APCI–MS method has been developed [29]. It allows the separation and online detection of the known ceramide classes and the species in them in one run. Using this new approach, we have investigated the mass spectrometric profile of each ceramide class in healthy test persons.
Materials and methods
Lipid collection procedure
The lipid collection procedure was permitted by the Ethics Committee of the Faculty of Medicine of our university, and was carried out by an experienced dermatologist.
Lipid extracts were obtained in vivo from seven healthy subjects (five women and two men with an average age of 37±13 y). Systemic and topical applications of drugs or skin care products were not allowed eight and four weeks prior to investigations, respectively.
The lipid collection procedure was a topical extraction that followed a protocol described previously [22]. A cylindrical glass beaker 4 cm i.d. (extraction area 12.56 cm2) was filled with 10 mL n-hexane/ethanol 2:1 (v/v). To prevent lateral leakage, the open side was pressed tightly to a skin area at the inner forearm. The glass beaker was attached by a PVC holder and an adjustable belt. The extraction time was set to 5 min throughout. The extraction was carried out twice on each forearm. This resulted in four extracts per volunteer. The four extracts from each volunteer were pooled and the solvents were evaporated at 50°C under a stream of nitrogen. The dried residue was stored at −80°C. Before use, the lipid extracts were separated from hydrophilic substances and proteins as described in detail elsewhere [30]. Briefly, methanol, chloroform and distilled water were added to the extracts in the ratio 2:1:0.8 (v/v/v) leading to a homogenous solution. Upon changing the ratio to 2:2:1.8 (v/v/v), two phases were created, with the stratum corneum lipids in the lower chloroform phase; this was used for the LC/APCI–MS analysis.
Ceramide separation and profiling by HPLC/APCI–MS
All separations were performed on a LC system consisting of a Spectra System P 4000 pump, an AS 3000 auto sampler, and a SN 4000 controller (Thermo Electron, San Jose, CA, USA). The stationary phase was a silica column LichroCart (125×4 mm, 5 μm particle size) filled with Si 60 Lichrospher particles (Merck, Darmstadt, Germany). The elutions were carried out at flow rate of 1 mL/min with a gradient consisting of two components A and B, where A was chloroform and B a mixture consisting of chloroform/n-propanol/acetic acid 80:20:2 (v/v/v). Further details are given in reference [29].
An ion trap mass spectrometer (Finnigan LCQ classic, Thermo Electron, San Jose, CA, USA) equipped with an atmospheric pressure chemical ionization source (APCI) was used throughout as the detector. The APCI heater was set to 500°C and the heated capillary to 150°C. Nitrogen was used as both auxiliary and sheath gas at flow rates of 9 and 1.2 L/min, respectively.
The entire flow from the HPLC was directed to the APCI source in all experiments. The injected amount of ceramide for each sample (including all ceramide classes) was 5 μg. The spectra of the ceramides were obtained in full scan mode between m/z 450 and 1500.
All solvents used for the topical extraction and for HPLC/APCI–MS were of HPLC grade and were purchased from Baker (Deventer, The Netherlands), Merck (Darmstadt, Germany), and Roth (Karlsruhe, Germany).
Results and discussion
The aim of this work was to obtain a mass spectrometric profile of the stratum corneum ceramide species within each of the known ceramide classes. In order to achieve this, effective extraction of the ceramides from the skin is crucial. This step was performed via a topical extraction procedure that has already been described in detail [22], which takes into account the effectiveness of the extraction (collection) as well as the compliance of the persons involved in the study.
Hyphenation of LC with mass spectrometry offers a combination of an effective separation with selective and sensitive detection. Taking the huge variations in structure of the stratum corneum ceramides into account, it is obvious that a normal phase separation of the ceramide classes is very important if we wish to assign the correct structures to the isobaric compounds. In contrast, reversed phase selectivity would result in an overlap of head group polarity and chain length effects. In Fig. 2 a chromatogram representing a normal phase separation of the stratum corneum ceramide classes is shown. The ceramide classes Cer [EOS], Cer [NS] Cer [NP], Cer [EOH], Cer [AP] and Cer [AH] have been identified. Evidence for the newly found Cer [EOP] was also obtained. Due to the fact that Cer [NH] and Cer [AS] are structural isomers [19], it is not surprising that the corresponding peaks show a partial overlap. However, species belonging to Cer [AS] typically contain short fatty acids (α-hydroxy palmitic acid is the most abundant) [31]. Nevertheless, the ultimate proof of correct signal assignment can only be given by MS/MS.
It should be mentioned that in this type of investigation only the ceramides were directed to the mass spectrometer, whereas other coextracted lipids were directed to waste using a divert valve.
The measurements were carried out in full scan mode between m/z 450 and 1500, which allows the extraction of every m/z that occurs afterwards. It is important to note that the high full scan sensitivity is an advantage of iontrap analyzers over quadrupole systems. Since the ionization conditions of APCI are somewhat harsher than those for ESI, there is a higher tendency for fragment formation. The operational parameters were adjusted in a way that fragmentation was limited. Consequently, ceramide molecules containing sphingosine showed a loss of one water molecule each, which could lead to a stabilized conjugated double bond system. In contrast, species with phytosphingosine did not show any fragmentation under the conditions used.
In order to assign the chain lengths to the fatty acids, a chain length of C18 was assumed for the sphingoid base, which is by far the most abundant [2, 24].
Tables 1 and 2 give the detected m/z values for the ceramide species. For ceramides with sphingosine as the sphingoid base, the masses were corrected for the loss of a water molecule. All measurements were performed in positive mode. In contrast to ESI, the negative mode did not work. As demonstrated in Table 1, species with chain lengths between C30 and C36 were detected for the ω-esterified ceramides. No fatty acid other than linolic acid was found at the ω-position in any of these species. Figure 3 shows the mass spectrometric profile of Cer [EOH] as an example of a ω-esterified ceramide class analyzed by LC/APCI–MS.
The detected species and the corresponding m/z values of the ceramide classes Cer [NS], Cer [NP], Cer [NH], Cer [AP] and Cer [AH] are listed in Table 2. Here, the chain lengths of the amide-linked fatty acids lie between C24 and C34. These results are consistent with data published by Vietzke et al. [28]. On the other hand, species with chain lengths C15–C18 have been detected for Cer [AS]. This conflicts with the results published by Vietzke et al., who found chain lengths between C22–C36. On the other hand, our findings correlate well with those of Wertz et al. [31]. The reason for this discrepancy is not known and should be clarified in additional experiments, preferably with the help of MS/MS.
The mass spectrum of Cer [NS] is shown in Figure 4. In this case, two homologous series are observed. Whereas one of the series is clearly related to Cer [NS], the m/z values of the second series can be assigned to the previously described [23, 24] but not yet established ceramide class Cer [NdS] that has dihydrosphingosine as its sphingoid base.
Mass spectrometric profile of Cer [NS]. Three-pronged symbols indicate Cer [NS] species ([M–H2O+H]+). Asterisks indicate Cer [NdS] species ([M+H]+). Note: mass differences between Cer [NS] and Cer [NdS] species with the same sphingoid base and amide-liked fatty acid chain lengths are not equal to 2 amu, because species of Cer [NS] lose a water molecule while those of Cer [NdS] do not
Tables 3 and 4 present the mass spectrometric profiles of all ceramide classes based on the relative abundances in all test persons. As shown in Table 3, the species with the C34 fatty acid in Cer [EOS] and Cer [EOP] have the highest relative abundances. On the other hand, the C32 species is most abundant in Cer [EOH]. Wertz et al. and Ponec et al. reported that species with chain lengths of C30 are the main components of the ω-esterified ceramides [18, 23, 24]. Interestingly, Vielhaber recently presented MALDI–TOF spectra of covalently bound Cer [EOS] and Cer [EOH] in which the highest relative intensities were recorded for species with chain lengths >C30 [32].
A number of previous publications have pointed out that the species C24 and C26 are the most abundant ones in the ceramide classes Cer [NS], Cer [NP], Cer [NH], Cer [AP] and Cer [AH], while C16 is the main representative of Cer [AS] [18, 23, 24]. According to Table 4, most of the ceramide classes mentioned above show the highest relative abundance for the C26 and/or C28 species. For Cer [NS], species with chain lengths >C28 are the most abundant, indicating a possible analogy to ω-esterified ceramides. α-Hydroxy palmitic acid (C16) was the main component of Cer [AS]. When discussing these different findings, we should bear in mind that both the location of the extraction area and the extraction procedure can influence the outcome of the study. In addition, the chain lengths of the sphingoid bases should be verified using MS/MS experiments.
Conclusion
Using a new approach with normal phase HPLC/APCI–MS, it was possible to create the mass spectrometric profile of each of the known ceramide classes in the stratum corneum. Initial hydrolysis into sphingoid bases and fatty acids, a step necessary for GC/MS, was not needed for this technique. In addition, ceramide classes such as Cer [EOS], Cer [EOH] and Cer [EOP], which are difficult to study using ESI–MS, were easily analyzed by means of normal phase LC/APCI–MS. This is the first time that MS profiles of these esterified ceramides have been reported. In addition, evidence for the so far not established Cer [NdS] was obtained. However, further characterization of all profiles using MS/MS experiments is needed. Comparison with mass spectrometric profiles could help us to understand the mechanisms associated with diseases such as atopic dermatitis and psoriasis that are known to impair the barrier function and alter lipid profiles. Application of this novel technique for diagnostic purposes is possible in the future.
References
Madison KC (2003) J Invest Dermatol 121:231–241
Coderch L, Lopez O, de la Maza A, Parra JL (2003) Am J Clin Dermatol 4:107–129
Elias PM, Friend DS (1975) J Cell Biol 65:180–191
Holleran WM, Feingold KR, Man MQ, Gao WN, Lee JM, Elias PM (1991) J Lipid Res 32:1151–1158
Sidransky E, Sherer DM, Ginns EI (1992) Pediatr Res 32:494–498
Holleran WM, Ginns EI, Menon GK, Grundmann JU, Fartasch M, McKinney CE, Elias PM, Sidransky E (1994) J Clin Invest 93:1756–1764
Melnik B, Hollmann J, Plewig G (1988) Br J Dermatol 119:547–549
Motta S, Monti M, Sesana S, Caputo R, Carelli S, Ghidoni R (1993) Biochim Biophys Acta 1182:147–151
Motta S, Monti M, Sesana S, Mellesi L, Ghidoni R, Caputo R (1994) Arch Dermatol 130:452–456
Di Nardo A, Wertz P, Giannetti A, Seidenari S (1998) Acta Derm Venereol 78:27–30
Bleck O, Abeck D, Ring J, Hoppe U, Vietzke JP, Wolber R, Brandt O, Schreiner V (1999) J Invest Dermatol 113:894–900
Imokawa G, Abe A, Jin K, Higaki Y, Kawashima M, Hidano A (1991) J Invest Dermatol 96:523–526
Robson KJ, Stewart ME, Michelsen S, Lazo ND, Downing DT (1994) J Lipid Res 35:2060–2068
De Paepe K, Weerheim A, Houben E, Roseeuw D, Ponec M, Rogiers V (2004) Skin Pharmacol Physiol 17:23–30
Raith K, Farwanah H, Wartewig S, Neubert R (2004) Eur J Lipid Sci Technol 106:561–571
Weerheim A, Ponec M (2001) Arch Dermatol Res 293:191–199
Ponec M, Boelsma E, Weerheim A (2000) Acta Derm Venereol 80:89–93
Ponec M, Weerheim A, Lankhorst P, Wertz P (2003) J Invest Dermatol 120:581–588
Stewart ME, Downing DT (1999) J Lipid Res 40:1434–1439
Bonté F, Pinguet P, Chevalier JM, Meybeck A (1995) J Chromatogr B 664:311–316
Zellmer S, Lasch J (1997) J Chromatogr B 691:321–329
Farwanah H, Neubert R, Zellmer S, Raith K (2002) J Chromatogr B 780:443–450
Wertz PW, Miethke MC, Long SA, Strauss JS, Downing DT (1985) J Invest Dermatol 84:410–412
Wertz PW, Swartzendruber DC, Madison KC, Downing DT (1987) J Invest Dermatol 89:419–425
Raith K, Zellmer S, Lasch J, Neubert R (2000) Anal Chim Acta 418:167–173
Raith K, Neubert R (2000) Anal Chim Acta 403:295–303
Vietzke JP, Strassner M, Hintze U (1999) Chromatographia 50:15–20
Vietzke JP, Brandt O, Abeck D, Rapp C, Strassner M, Schreiner V, Hintze U (2001) Lipids 36:299–304
Farwanah H, Nuhn P, Neubert R, Raith K (2003) Anal Chim Acta 492:233–239
Signorelli P, Hannun YA (2002) Methods Enzymol 345:275–294
Wertz PW (2000) Acta Derm Venereol Suppl (Stockh) 208:7–11
Vielhaber G, Pfeiffer S, Brade L, Lindner B, Goldmann T, Vollmer E, Hintze U, Wittern KP, Wepf R (2001) J Invest Dermatol 117:1126–1136
Acknowledgements
The authors thank M. Woigk for technical assistance and C. Schmelzer for fruitful discussions and constant support.
Author information
Authors and Affiliations
Corresponding author
Rights and permissions
About this article
Cite this article
Farwanah, H., Wohlrab, J., Neubert, R.H.H. et al. Profiling of human stratum corneum ceramides by means of normal phase LC/APCI–MS. Anal Bioanal Chem 383, 632–637 (2005). https://doi.org/10.1007/s00216-005-0044-3
Received:
Revised:
Accepted:
Published:
Issue Date:
DOI: https://doi.org/10.1007/s00216-005-0044-3