Abstract
Analysis of the genome sequence of Vibrio parahaemolyticus reveals two IcmF family genes in putative type VI secretion system (vpT6SS) clusters in chromosomes 1 (icmF1) and 2 (icmF2). The icmF1 gene is present in majority of clinical isolates (87.5 %), but has a low fraction (25.0 %) in environmental isolates. However, icmF2 is contained in all strains of both clinical and environmental sources. Deletion of either icmF1 or hcp1 significantly reduced bacterial adhesion to Caco-2 cells or HeLa monolayers. However, the ΔicmF2 and Δhcp2 mutants showed decreased adhesion only to HeLa monolayers. Western blot analysis showed that Hcp2 was present both in the supernatant and pellet samples in the wild-type strain, but only in the pellet of the ΔicmF2 mutant, indicating that Hcp2 is a translocon of T6SS2. Although vpT6SS1 might be functional in cellular adhesion, the putative translocon Hcp1 was not detectable. Quantitative PCR revealed 10-fold and 17-fold less transcripts of hcp1 and icmF1 mRNA than those of hcp2 and icmF2 accordingly. Thus, we postulate that the putative vpT6SS systems contribute to adhesion of V. parahaemolyticus to host cells.
Similar content being viewed by others
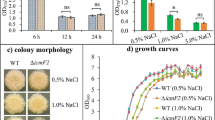
Avoid common mistakes on your manuscript.
Introduction
Vibrio parahaemolyticus, a halophilic bacterium in marine environments, is the leading cause of gastroenteritis in humans in China due to consumption of raw or under-cooked seafood. The virulence factors include thermostable direct hemolysin (TDH), TDH-related hemolysin (TRH), and type III secretion system (T3SS) (Kaper et al. 1984; Nishibuchi et al. 1986; Park et al. 2004). Most clinical isolates of V. parahaemolyticus exhibit Kanagawa phenomenon (KP) induced by TDH on Wagatsuma agar medium; however, only 1–3 % of the environmental isolates are KP positive (Shirai et al. 1990; Lozano-León et al. 2003; Robert-Pillot et al. 2004). TDH causes several cytotoxic effects, such as erythrocyte lysis, disruption of the microtubule cytoskeleton, and ion influx into cultured cells (Honda et al. 1988; Raimondi et al. 2000). Both T3SS1 and T3SS2 are identified as virulence factors. T3SS1 is present in all strains, contributes to cytotoxicity in HeLa cells, and induces autophagy (Park et al. 2004; Burdette et al. 2008). T3SS2, however, is found only in tdh- or trh-positive strains, and cytotoxic to Caco-2 cells and intestines (Park et al. 2004; Okada et al. 2009).
IcmF, a gene encoding the intracellular multiplication factor (IcmF), was first studied in Legionella pneumophila as part of the Dot/Icm cluster of genes that form type IV secretion system (T4SS) involved in host cell killing and intracellular multiplication (Purcell and Shuman 1998; Segal et al. 1998). Recently, the IcmF family proteins were identified as innermembrane proteins of VI secretion system (T6SS) (Shrivastava and Mande 2008). T6SS is a macromolecular transenvelope machine encoded within the genomes that is only found in proteobacteria (Pukatzki et al. 2006; Mougous et al. 2006; Bingle et al. 2008; Bernard et al. 2010). The system contains 13–20 proteins (IcmF-associated homologous proteins, IAHP) coded for by the gene clusters (Boyer et al. 2009). Deletion of icmF-associated proteins usually does not affect expression of the translocon Hcp (hemolysin coregulated protein) but prevents its translocation (Pukatzki et al. 2006; Suarez et al. 2008).
The IAHP gene cluster of V. cholerae was required for secretion of Hcp and VgrG proteins and for cytotoxicity toward Dictyostelium amoebae and J774 macrophages (Pukatzki et al. 2006). Mougous et al. (2006) provided evidence that the apparatus was functional during chronic lung infection of Pseudomonas aeruginosa in cystic fibrosis patients. In Edwardsiella tarda, T6SS mutant strains were attenuated in blue gourami fish (Zheng and Leung 2007). T6SS has been found to be involved in increased survival within macrophages in a number of bacterial species such as Aeromonas hydrophila (Suarez et al. 2008), and Salmonella enterica (Parsons and Heffron 2005). In Vibrio anguillarum, T6SS was involved in resistance to stress sensing, such as low pH, ethanol, and hydrogen peroxide (Weber et al. 2009). However, bacterial T6SS are not functionally limited to eliciting diseases. They may compromise virulence or play a role in interbacterial competition (Jani and Cotter 2010).
The T6SS1 cluster in V. parahaemolyticus was found as divergent by microarray hybridization between pandemic strains and non-pandemic strains (Izutsu et al. 2008). About eighty percent of the V. parahaemolyticus pandemic strains harbored five genes (VP1390, VP1401, VP1405, VP1409, and VP1418) of T6SS1, and half of the nonpathogenic strains harbored some of the T6SS1 genes (Chao et al. 2010). However, functions of T6SS systems in V. parahaemolyticus remain unclear so far. We have found two icmF homologs in V. parahaemolyticus, icmF1 present in putative T6SS1 cluster on chromosome I (vpT6SS1) and icmF2 in putative T6SS2 cluster on chromosome II (vpT6SS2). In the present study, we attempted to examine if the putative vpT6SS systems are functional in cell adhesion and cytotoxicity by disruption of their putative structural proteins (IcmF1 and IcmF2) or effector proteins (Hcp1 and Hcp2).
Methods
Bacterial strains and plasmids
The bacterial strains and plasmids are described in Table 1. V. parahaemolyticus HZ is a clinical isolate from the Zhejiang Provincial Center for Disease Control and Prevention, Zhejiang, China. E. coli strains DH5α, BL21, and CC118λpir were used for general manipulation of plasmids, prokaryotic expression of proteins, and mobilization of plasmids into V. parahaemolyticus, respectively. The bacterial strains were grown at 37 °C in Luria–Bertani (LB) broth (E. coli) or LB broth supplemented with 3 % NaCl (V. parahaemolyticus). LB agar supplemented with 3 % NaCl, 10 μg/ml chloramphenicol, and 25 μg/ml polymyxin was used for screening mutant strains. The culture media were supplemented, where appropriate, with the following antibiotics: chloramphenicol (Cm, 10 μg/ml), ampicillin (Amp, 100 μg/ml), and kanamycin (Kana, 50 μg/ml).
Generation of mutant strains
In-frame gene deletion of icmF1, icmF2, hcp1, hcp2, tdh, vcrD1 (encoding the T3SS1 structural protein), and vcrD2 (encoding the T3SS2 structural protein), either alone or multiple (the triple deletion mutant DTTT void of tdh, vcrD1, and vcrD2, or quadruple mutants of DTTT plus additional deletion of icmF1, icmF2, hcp1, or hcp2) (Table 1), was generated by sacB-based allelic exchange as described previously (Park et al. 2004). Briefly, PCR amplification was performed to generate the upstream and downstream fragments of the target genes (using respective primer pairs A/B and C/D, Table S1). Overlap PCR was performed to construct a fragment with deletion of the target gene using the primer pairs A/D. The fragment was cloned into pMD18T vector (Takara) and then subcloned into an R6 K-ori suicide vector pYAK1 that contains the sacB gene conferring sensitivity to sucrose. The recombinant plasmid was introduced into E. coli CC118λpir and then mated with V. parahaemolyticus. The resulting mutant strains were screened using selective LB agar as specified above.
Gene complementation in mutant strains
For icmF1 complementation, the primer pairs picmF1-F and picmF1-R were used to amplify the entire icmF1 ORF. The PCR product digested with EcoRI and BamHI was cloned into pMMB207. The resulting plasmid picmF1 was transferred into E. coli CC118λpir and then conjugated into V. parahaemolyticus. A volume of 400-μl overnight culture of V. parahaemolyticus containing picmF1 was inoculated into 5 ml of LB and incubated for 2 h with shaking at 28 °C before adding 1 mM IPTG. Protein expression was induced at 28 °C for 12 h (Zhou et al. 2008). Complementation of icmF2, hcp1, and hcp2 was carried out in similar procedures using specific primer pairs picmF2-F/R, phcp1-F/R, and phcp2-F/R (Table S1).
Antibody production
The PCR products harboring hcp1 and hcp2 were amplified with primer pairs hcp1-F/R and hcp2-F/R and cloned into expression vector pET30a(+). Protein expression was induced in E. coli BL21 with IPTG. The bacterial pellet was washed twice with phosphate buffered saline (PBS) at pH 7.2 and sonicated intermittently for 15 min. His-tagged proteins were purified with nickel column (Bio-Rad). Polyclonal antibodies to Hcp1 and Hcp2 were raised by immunizing female New Zealand white rabbits (Park et al. 2004). Animal use was approved by Zhejiang University Committee for Experimental Animals and Animal Welfare.
Western blot analysis
Secreted proteins from the parent and mutant V. parahaemolyticus strains were prepared from supernatant samples of bacterial cultures grown for 16 h at 28 °C in LB broth. Supernatant samples were passed through a 0.22-μm pore-size syringe filter and precipitated by adding trichloroacetic acid to a final concentration of 10 % (vol/vol). The proteins were collected by centrifugation at 15,000 g for 30 min at 4 °C. The precipitates were solubilized in 40-μl 0.1 M NaOH, and 10 μl of 5 × SDS-PAGE loading buffer was added prior to SDS-PAGE with 10 % polyacrylamide. For separation of vpT6SS proteins associated with the bacterial cells, V. parahaemolyticus cultures were pelleted by centrifugation, and the pellets were resuspended in PBS (100 mg wet weight per ml). A volume of 160 μl was then mixed with 10 μl of 5 × SDS-PAGE loading buffer, and the mixtures were heat-treated for 5 min in a boiling water-bath to release proteins from the bacterial cells before SDS-PAGE.
Proteins on the gels were electro-transferred onto an Immobilon-P membrane (Millipore). The membranes were blocked with 5 % skim milk in tris-buffered saline (20 mM Tris, 137 mM NaCl, pH 7.6) containing 0.05 % Tween 20 and probed with anti-Hcp1 or anti-Hcp2 polyclonal antibodies for 1 h at room temperature. The blots were then probed with anti-rabbit IgG conjugated to horseradish peroxidase (Amersham Life Sciences), and developed by the SuperSignala West Pico Chemiluminescent Substrate (Pierce) according to the manufacturer’s instruction.
Adhesion assay
HeLa cell monolayers were grown to about 80 % confluency in Dulbecco’s modified eagle medium (DMEM) supplemented with 10 % new-born calf serum at 37 °C and 5 % CO2. Caco-2 cell monolayers were grown to about 80 % confluency in RPMI-1640 medium with 20 % NBCS at 37 °C and 5 % CO2. Overnight cultures of V. parahaemolyticus HZ or its mutants (with or without complementation) were subcultured in LB broth for further incubation at 37 °C for 3 h. The cultures were pelleted by centrifugation and re-suspended in DMEM or RPMI-1640 and adjusted to OD600 at 0.20 ± 0.02 (about 108 CFU/ml) as stock inocula. Cell monolayers were infected at multiplicity of infection (MOI) of 1:100. After incubation at 37 °C and 5 % CO2 for 1 h, the monolayers were washed twice with PBS and lysed with 0.01 % Triton-X 100. Serial 10-fold dilutions were made from the lysates and bacteria adhered to the cells were counted on LB agar plates. Percent adherence was calculated as bacterial cells adhered/bacterial cells added into the well × 100. The inoculum sizes of the strains or mutants for all experiments ranged from 2.3 to 3.9 × 107 CFU/well. Adhesion of the wild-type strain HZ was initialized as 100 % for comparison with its mutants with or without complementation. When testing the effect of anti-Hcp1 polyclonal antibody on adherence, the anti-Hcp1 serum and control serum (pooled from 3 rabbits not immunized with Hcp1) were added into the adhesion cultures at the final concentration of 1:400.
Cytotoxicity assay
HeLa or Caco-2 cells and cultures of V. parahaemolyticus or its mutants were prepared as for the adhesion assay above. The cell monolayers were inoculated with the bacteria at MOI of 1:100 in 500 μl DMEM or RPMI-1640, and incubated at 37 °C and 5 % CO2 for 2–4 h. The culture supernatants were collected for lactate dehydrogenase activity (LDH) using CytoTox 96 non-radioactive cytotoxicity assay (Promega).
Real-time PCR
To compare transcription levels of hcp1, hcp2, icmF1, and icmF2 in the wild-type strain, overnight culture of V. parahaemolyticus HZ was subcultured in LB broth at 37 °C for 6 h. Total RNA extraction kit (Shanghai Sangon, China) was used to extract mRNA for cDNA synthesis using random primers (Takara). Real-time PCR was performed in a total volume of 20 μl:10 μl of SYBR qPCR Mix (TOYOBO), 0.8 μl of each primer (QF/R-hcp1 and QF/R-hcp2, Table S1) (final concentration 400 nM), 2 μl of cDNA, and 7.2 μl of Milli-Q water. Transcription levels were quantified by linear regression of the C t values against DNA copies of recombinant pET30a vectors containing hcp1, hcp2, icmF1, and icmF2 which were calculated (http://www.uri.edu/research/gsc/resources/cndna.html) and run simultaneously with the test samples.
Statistical analysis
The experiments for adhesion assays were repeated three times and all experiments were performed in triplicate wells. Data were expressed as mean ± SD. Student’s t test was used to extract statistical differences.
Results
icmF1 has higher frequency in clinical V. parahaemolyticus strains than non-clinical ones
Bioinformatic analysis reveals two IcmF family genes in V. parahaemolyticus, icmF1 on chromosome 1 and icmF2 on chromosome 2. PCR typing showed that icmF1 has different distributions, 90.9 % (30/33) positive in clinical strains, but 25 % (127/507) in non-clinical strains (Table 2), suggesting that icmF-related T6SS1 is possibly functional in the pathogenesis of V. parahaemoyticus. However, icmF2 exists in all V. parahaemolyticus strains. Gene typing showed that virulence genes tdh, trh, and vcrD2 (representing T3SS2, Park et al. 2004) are more prevalent in clinical strains than in non-clinical ones (Table 2). The icmF1 gene is present in all T3SS2-positive strains.
The putative vpT6SS1 and vpT6SS2 are involved in adhesion to cultured cells
The ΔicmF1 and ΔicmF2 mutant strains showed similar growth patterns to their parent strain in vitro (data not shown). In Caco-2 cell monolayers, the icmF1 mutant had about 53 % lower adhesion than the parent strain HZ (Fig. 1a). In HeLa cells, both ΔicmF1 and ΔicmF2 mutants had significantly lower levels of adhesion than strain HZ (Fig. 1b). Since several different V. parahaemolyticus cytotoxic factors, including TDH, T3SS1, and T3SS2, can disrupt mammalian cell structures (Nishibuchi and Kaper 1995; Park et al. 2004; Okada et al. 2009), we further examined the effect on adhesion contributed by individual icmF1, icmF2, Hcp1, or Hcp2 in the absence of cytotoxic components by making quadruple mutants from the triple deletion mutant void of tdh, vcrD1, and vcrD2 (named DTTT, Table 1) with additional deletion of icmF1, icmF, hcp1, or hcp2 (Table 1). In Caco-2 cell monolayers, deletion of either icmF1 or hcp1 had about 64–68 % lower adhesion than DTTT (Fig. 2a). In HeLa cell monolayers, ΔicmF1, ΔicmF2, Δhcp1, and Δhcp2 mutants had significantly lower levels of adhesion than their parent strain DTTT (ranging from 51 to 63 %) (Fig. 2b). Such impaired adhesion was restorable by complementation of the relative genes (Fig. 2). These results suggest that both vpT6SS1 and vpT6SS2 are involved in adhesion to HeLa cells, but only T6SS1 is involved in adhesion to Caco-2 cells.
Adhesion to Caco-2 and HeLa cell monolayers of V. parahaemolyicus strain HZ and its mutant strains ΔicmF1 and ΔicmF2. Adhesion of the wild-type strain HZ (added at 2.2–2.8 × 107 CFU to the cell monolayers with post-washing recovery at 0.95–1.75 × 107 CFU for different experiments) was initialized to 100 %. Deletion of icmF1 reduced bacterial adhesion to both Caco-2 and HeLa cells, while deletion of icmF2 affected adhesion only to HeLa cells
Adhesion to Caco-2 and HeLa cell monolayers of the triple deletion mutant (DTTT, void of tdh as well as T3SS1 and T3SS2 structural protein genes vcrD1 and vcrD2) and its isogenic mutants with additional deletion of icmF1, icmF2, hcp1, or hcp2. Reduced adhesion to the cell monolayers due to deletion of T6SS1- or T6SS2-related icmF or hcp genes was recoverable by genetic complementation. Adhesion of the triple DTTT mutant strain (added at 2.3–3.9 × 107 CFU to the cell monolayers with post-washing recovery at 1.3–2.13 × 107 CFU for different experiments) was initialized to 100 %
IcmF2 constitutes the structure of functional vpT6SS2, and Hcp2 is likely the adhesion molecule upon translocation
A canonical T6SS consists of several structural proteins, the innermembrane protein IcmF, two translocon proteins (usually Hcp family protein and VgrG family protein), and some secretion proteins (Cascales 2008). Deletion of structural genes usually prevents protein translocation and secretion (Pukatzki et al. 2006). Genome analysis of V. parahaemolyticus shows that icmF1 and icmF2 are embedded in two putative T6SS clusters. Therefore, we examined the putative translocon proteins Hcp1 and Hcp2 in respective clusters in pellets and supernatants of ΔicmF1 and ΔicmF2 mutant strains using Hcp1 and Hcp2 polyclonal antibodies. Figure 3 shows that Hcp2 could be detected both in pellet and supernatant from the wild-type strain HZ, but only in the pellet in the ΔicmF2 mutant (Fig. 3a). However, the supernatant Hcp2 was detectable upon complementation of icmF2 in the ΔicmF2 mutant. These results indicate that IcmF2 is the component of the functional T6SS2 for efficient translocation of Hcp2.
Hcp1 has low transcription and expression
Because Hcp1 was not detectable using the enhanced chemiluminescence imaging, we suspected that hcp1 might have low expression in V. parahaemolyticus. We introduced the recombinant expression plasmid phcp1 into the wild-type strain HZ or its ΔicmF1 mutant to see if extrachromosomal expressed Hcp1 could be detectable. Hcp1 was detected only in the pellet samples, but not in supernatants, of the strain HZ and ΔicmF1 mutant carrying phcp1(Fig. 4b). No target bands were developed either in the pellet or supernatant samples from the corresponding strains containing the control vector pMMB207 (Fig. 4). We further supposed that low expression of Hcp1 might be due to low transcription of hcp1. Real-time PCR showed that the transcriptional level of icmF1 was about 10-fold lower than icmF2 (4.90 × 103 vs. 4.84 × 104 cDNA copies). The hcp1 gene had 2.41 × 106 copies, about 17-fold lower than that of hcp2 (4.22 × 107 copies) (Fig. 4c, d). These results strongly suggest that the putative T6SS1 genes had lower expression and transcription than their counterparts of T6SS2 in V. parahaemolyticus. However, Hcp1 did contribute to adhesion to cultured cells, as shown in Fig. 2 as well as by adhesion inhibition assay using anti-Hcp1 antibody, about 50 % lower recovery of the mutant strain DTTT in cultured cells treated with anti-Hcp1 antibody than that using control serum (Fig. 4e), suggesting that Hcp1 might be activated once V. parahaemolyticus attached to cultured cells by an unknown mechanism.
The putative vpT6SS1 and vpT6SS2 do not mediate cytotoxicity
To examine if putative vpT6SSs are involved in cytotoxic effect on eukaryotic cells, deletion mutants of icmF1, icmF2, hcp1, or hcp2 were constructed from the parent strain HZ or DTTT. Single deletion of icmF1, icmF2, hcp1, or hcp2 did not cause significant changes of LDH release from both Caco-2 and HeLa cell monolayers, as compared to their parent strain HZ (Table 3). Deletion of vcrD1 and vcrD2 almost abolished the cytotoxic effect of V. parahaemolyticus on Caco-2 cells, and also on HeLa cells, but to a lesser extent. Further deletion of T6SS-related genes from the DTTT triple deletion mutant did not have apparent effect on cytotoxicity.
Discussion
Early studies linked gastroenteritis to the presence of tdh, trh, T3SS1, and/or T3SS2, which caused general cytotoxicity or enterotoxicity (Alam et al. 2002; Yeung and Boor 2004; Park et al. 2004; Okada et al. 2009). Here, we provide evidence that the putative vpT6SS systems contribute to adhesion of V. parahaemolyticus to host cells most probably by the translocon proteins Hcp1 and Hcp2, or other unknown factors which were translocated likely by the T6SS structural IcmF family proteins.
The icmF family protein, though first found as a component of T4SS in L. pneumophila (Sexton and Vogel 2002), has been found as the component of T6SS in several bacterial species such as V. cholerae, P. aeruginosa, A. hydrophilia (Pukatzki et al. 2006; Mougous et al. 2006; Suarez et al. 2008) and participates in bacterial pathogenicity: adhesion to epithelial cells, cytotoxicity, resistance to phagocytosis, and replication inside the host cell (Zheng and Leung 2007; Suarez et al. 2008; Mougous et al. 2006; Bingle et al. 2008; Jani and Cotter 2010; Chow and Mazmanian 2010). By domain blast in EMBL-EBL (http://www.ebi.ac.uk/Tools/pfa/iprscan/), VP1408 and VPA1039 in V. parahaemolyticus were found as the T6SS IcmF family proteins and have an icmF-related domain at position 489–755 aa of IcmF1 (VP1408) and position 502–805 aa of IcmF2 (VP1039). VP1393 (Hcp1) and VPA1027 (Hcp2) belong to the hcp1-like superfamily and have a DUF796 domain (position 6–156aa of Hcp1, 6–134aa of Hcp2) defined as virulence factors and secretion apparatus (Bingle et al. 2008; Boyer et al. 2009).
The Hcp family proteins are considered as effectors of T6SS in other bacterial species (Boyer et al. 2009; Cascales 2008; Jani and Cotter 2010). Here, we show that T6SS2 is an active secretion system and Hcp2 is the translocon of T6SS2. Intriguing to us is that we were not able to detect the presence of Hcp1 in the supernatant or pellet samples of the wild-type strain HZ, although T6SS1 was functional. Real-time PCR and Western blot showed that genes of T6SS1 were in a low transcriptional and expressional level (probably below 3 ng/ml, a level detectable with our enhanced chemiluminescence imaging). Low expression of hcp1 was also seen in other bacterial species. V. cholerae strain N16961 has the VAS cluster (T6SS cluster) genes almost identical to V. cholerae strain V52. However, Hcp was not detected in its supernatant or pellets, as contrast to that in the strain V52 (Pukatzki et al. 2006). Recently, LuxO and TsrA were found to regulate T6SS in V. cholerae and their deletion led to increased Hcp expression as well as induction of T6SS-dependent fecal diarrhea and intestinal inflammation in infant rabbits (Zheng et al. 2010). In P. aeruginosa, HSI-1(T6SS) was believed to be involved in the course of cystic fibrosis. However, Hcp1 could only be detectable in the mutant strain with deletion of retS, a global regulator, but not in the wild-type PAO1 in vitro (Mougous et al. 2006). Therefore, it is possible that vpT6SS1 could be negatively regulated by an unknown factor and might be expressed at higher level in vivo.
However, T6SS systems are not the only factors contributing to adhesion of V. parahaemolyticus to host cells. A recent study shows that multivalent adhesion molecule 7 (MAM7) is involved in adhesion of V. parahaemolyticus to host cells by forming complex with fibronectin as the host cell ligand (Krachler and Orth 2011).
Adherence is a prerequisite event for bacterial infection. Attachment to epithelial cells is dependent on surface characteristics of both the bacteria and the host cells (Sakarya et al. 2010). We have found apparent differences between vpT6SS1 and vpT6SS2 in adhesion to the cell lines used, with the former being involved in adhesion to both Caco-2 and HeLa cells and the latter to HeLa cells only. This phenomenon might be due to differences between Caco-2 and HeLa cells in their surface characteristics such as receptors interacting with vpT6SS. Since Caco-2 is an intestinal epithelial cell line, T6SS1 may play an important role in enteric infection of V. parahaemolyticus.
In conclusion, we reveal that vpT6SS systems, though not involved in cytotoxicity as is the case with other bacterial T6SS (Pukatzki et al. 2006; Suarez et al. 2008), are functional for adhesion to host cells most probably via their Hcp2 and/or Hcp1. Because cytotoxic T3SS2 co-exists with vpT6SS1, both targeting on enterocytes (Park et al. 2004), we postulate that V. parahaemolyticus has developed an effective strategy of having both secretion systems functionally cooperative, T6SS1 playing its role in adhesion, the first step of infection, and the effectors exported by T3SS2 inducing enterocytotoxicity.
Reference
Alam MJ, Tomochika KI, Miyoshi SI, Shinoda S (2002) Environmental investigation of potentially pathogenic Vibrio parahaemolyticus in the Seto-Inland Sea, Japan. FEMS Microbiol Lett 208:83–87
Bernard CS, Brunet YR, Gueguen E, Cascales E (2010) Nooks and crannies in type VI secretion regulation. J Bacteriol 192:3850–3860
Bingle LE, Bailey CM, Pallen MJ (2008) Type VI secretion: a beginner’s guide. Curr Opin Microbiol 11:3–8
Boyer F, Fichant G, Berthod J, Vandenbrouck Y, Attree I (2009) Dissecting the bacterial type VI secretion system by a genome wide in silico analysis: what can be learned from available microbial genomic resources? BMC Genomics 10:104
Burdette DL, Yarbrough ML, Orvedahl A, Gilpin CJ, Orth K (2008) Vibrio parahaemolyticus orchestrates a multifaceted host cell infection by induction of autophagy, cell rounding, and then cell lysis. Proc Natl Acad Sci USA 105:12497–12502
Cascales E (2008) The type VI secretion tool kit. EMBO Rep 9:735–741
Chao G, Jiao X, Zhou X, Wang F, Yang Z, Huang J, Pan Z, Zhou L, Qian X (2010) Distribution of genes encoding four pathogenicity islands (VPaIs), T6SS, biofilm, and type I pilus in food and clinical strains of Vibrio parahaemolyticus in China. Foodborne Pathog Dis 7:649–658
Chow J, Mazmanian SK (2010) A pathobiont of the microbiota balances host colonization and intestinal inflammation. Cell Host Microbe 7:265–276
Honda T, Ni Y, Miwatani T (1988) Purification and characterization of a hemolysin produced by a clinical isolate of Kanagawa phenomenon-negative Vibrio parahaemolyticus and related to the thermostable direct hemolysin. Infect Immun 56:961–965
Izutsu K, Kurokawa K, Tashiro K, Kuhara S, Hayashi T, Honda T, Iida T (2008) Comparative genomic analysis using microarray demonstrates a strong correlation between the presence of the 80-kilobase pathogenicity island and pathogenicity in Kanagawa phenomenon-positive Vibrio parahaemolyticus strains. Infect Immun 76:1016–1023
Jani AJ, Cotter PA (2010) Type VI secretion: not just for pathogenesis anymore. Cell Host Microbe 8:2–6
Kaper JB, Campen RK, Seidler RJ, Baldini MM, Falkow S (1984) Cloning of the thermostable direct or Kanagawa phenomenon associated hemolysin of Vibrio parahaemolyticus. Infect Immun 45:290–292
Krachler AM, Orth K (2011) Functional characterization of the interaction between bacterial adhesin multivalent adhesion molecule 7 (MAM7) protein and its host cell ligands. J Biol Chem 286:38939–38947
Lozano-León A, Torres J, Osorio CR, Martínez-Urtaza J (2003) Identification of tdh positive Vibrio parahaemolyticus from an outbreak associated with raw oyster consumption in Spain. FEMS Microbiol Lett 226:281–284
Mougous JD, Cuff ME, Raunser S, Shen A, Zhou M, Gifford CA, Goodman AL, Joachimiak G, Ordoñez CL, Lory S, Walz T, Joachimiak A, Mekalanos JJ (2006) A virulence locus of Pseudomonas aeruginosa encodes a protein secretion apparatus. Science 312:1526–1530
Nishibuchi M, Kaper BJ (1995) Thermostable direct hemolysin gene of Vibrio parahaemolyticus: a virulence gene acquired by a marine bacterium. Infect Immun 63:2093–2099
Nishibuchi M, Hill WE, Zon G, Payne WL, Kaper JB (1986) Synthetic oligodeoxyribonucleotide probes to detect Kanagawa phenomenon-positive Vibrio parahaemolyticus. J Clin Microbiol 23:1091–1095
Okada N, Iida T, Park KS, Goto N, Yasunaga T, Hiyoshi H, Matsuda S, Kodama T, Honda T (2009) Identification and characterization of a novel type III secretion system in trh-positive Vibrio parahaemolyticus strain TH3996 reveal genetic lineage and diversity of pathogenic machinery beyond the species level. Infect Immun 77:904–913
Park KS, Ono T, Rokuda M, Jang MH, Okada K, Iida T, Honda T (2004) Functional characterization of two type III secretion systems of Vibrio parahaemolyticus. Infect Immun 72:6659–6665
Parsons DA, Heffron F (2005) sciS, an icmF homolog in Salmonella enterica serovar Typhimurium, limits intracellular replication and decreases virulence. Infect Immun 73:4338–4345
Pukatzki S, Ma AT, Sturtevant D, Krastins B, Sarracino D, Nelson WC, Heidelberg JF, Mekalanos JJ (2006) Identification of a conserved bacterial protein secretion system in Vibrio cholerae using the Dictyostelium host model system. Proc Natl Acad Sci USA 103:1528–1533
Purcell M, Shuman HA (1998) The Legionella pneumophila icmGCDJBF genes are required for killing of human macrophages. Infect Immun 66:2245–2255
Raimondi F, Kao JPY, Fiorentini C, Fabbri A, Donelli G, Gasparini N, Rubino A, Fasano A (2000) Enterotoxicity and cytotoxicity of Vibrio parahaemolyticus thermostable direct hemolysin in in vitro systems. Infect Immun 68:3180–3185
Robert-Pillot A, Guenole A, Lesne J, Delesmont R, Fournier JM, Quilici ML (2004) Occurrence of the tdh and trh genes in Vibrio parahaemolyticus isolates from waters and raw shellfish collected in two France coastal areas and from seafood imported into France. Int J Food Microbiol 91:319–325
Sakarya S, Göktürk C, Öztürk T, Ertugrul MB (2010) Sialic acid is required for nonspecific adherence of Salmonella enterica ssp. enteriaca serovar Typhi on Caco-2 cell. FEMS Immunol Med Microbiol 58:330–335
Segal G, Purcell M, Shuman HA (1998) Host cell killing and bacterial conjugation require overlapping sets of genes within a 22-kb region of the Legionella pneumophila genome. Proc Natl Acad Sci USA 95:1669–1674
Sexton JA, Vogel JP (2002) Type IVB secretion by intracellular pathogens. Traffic 3:178–185
Shirai H, Ito H, Hirayama T, Nakamoto Y, Nakabayashi N, Kumagai K, Takeda Y, Nishibuchi M (1990) Molecular epidemiologic evidence for association of thermostable direct hemolysin (TDH) and TDH-related hemolysin of Vibrio parahaemolyticus with gastroenteritis. Infect Immun 58:3568–3573
Shrivastava S, Mande SS (2008) Identification and functional characterization of gene components of Type VI secretion system in bacterial genomes. PLoS ONE 3:e2955
Suarez G, Sierra JC, Sha J, Wang S, Erova TE, Fadl AA, Foltz SM, Horneman AJ, Chopra AK (2008) Molecular characterization of a functional type VI secretion system from a clinical isolate of Aeromonas hydrophila. Microb Pathog 44:344–361
Vongxay K, He X, Cheng S, Zhou X, Shen B, Zhang G, Zhang Y, Fang W (2006) Prevalence of Vibrio parahaemolyticus in seafood and their processing environments as detected by duplex PCR. J Sci Food Agric 86:1871–1877
Vongxay K, Wang S, Zhang X, Wu B, Hu H, Pan Z, Chen S, Fang W (2008) Pathogenic characterization of Vibrio parahaemolyticus isolates from clinical and seafood sources. Int J Food Microbiol 126:71–75
Wang Q, Liu Q, Ma Y, Rui H, Zhang Y (2007) LuxO controls extracellular protease, haemolytic activities and siderophore production in fish pathogen Vibrio alginolyticus. J Appl Microbiol 103:1525–1534
Weber B, Hasic M, Chen C, Wai SN, Milton DL (2009) Type VI secretion modulates quorum sensing and stress response in Vibrio anguillarum. Environ Microbiol 11:3018–3028
Yeung PS, Boor KJ (2004) Epidemiology, pathogenesis, and prevention of foodborne Vibrio parahaemolyticus infections. Foodborne Pathog Dis 1:74–89
Zheng J, Leung KY (2007) Dissection of a type VI secretion system in Edwardsiella tarda. Mol Microbiol 66:1192–1206
Zheng J, Shin OS, Cameron DE, Mekalanos JJ (2010) Quorum sensing and a lobal regulator TsrA control expression of type VI secretion and virulence in Vibrio cholerae. Proc Natl Acad Sci USA 107:21128–21133
Zhou X, Shah DH, Konkel ME, Call DR (2008) Type III secretion system 1 genes in Vibrio parahaemolyticus are positively regulated by ExsA and negatively regulated by ExsD. Mol Microbiol 69:747–764
Acknowledgments
This research is funded in part by Natural Science Foundation of China (30571436 to WHF and 30700605 to BBW) and the Key Project of National Science and Technology Pillar Program (2009BADB9B01). We thank Dr T. Iida at Osaka University for providing the suicide vector pYAK1.
Author information
Authors and Affiliations
Corresponding author
Additional information
Communicated by Jorge Membrillo-Hernandez.
Electronic supplementary material
Below is the link to the electronic supplementary material.
Rights and permissions
About this article
Cite this article
Yu, Y., Yang, H., Li, J. et al. Putative type VI secretion systems of Vibrio parahaemolyticus contribute to adhesion to cultured cell monolayers. Arch Microbiol 194, 827–835 (2012). https://doi.org/10.1007/s00203-012-0816-z
Received:
Revised:
Accepted:
Published:
Issue Date:
DOI: https://doi.org/10.1007/s00203-012-0816-z