Abstract
Key message
A begomovirus resistance gene Pepy-2 encoding the DFDGD-Class RNA-dependent RNA polymerase 3a was identified in pepper (C. annuum) through the forward and reverse genetic analyses.
Abstract
In several countries throughout the world, the whitefly-transmitted begomovirus causes massive yield losses in pepper (Capsicum spp.) production. Although introgression of the genetic resistance against begomovirus to commercial cultivars is strongly required, the recently discovered recessive resistance gene pepy-1, which encodes the messenger RNA surveillance factor Pelota, is the only begomovirus resistance gene identified in Capsicum so far. In this study, we fine-mapped another begomovirus resistance gene from PG1-1 (C. annuum), which is resistant to pepper yellow leaf curl Indonesia virus (PepYLCIV) and pepper yellow leaf curl Aceh virus (PepYLCAV), to further speed up the marker-assisted breeding of begomovirus resistance in peppers. A single dominant locus, Pepy-2, conferring resistance against PepYLCIV in PG1-1 was identified on chromosome 7 by screening recombinants from the F2 and F3 segregating populations derived from a cross between PG1-1 and begomovirus susceptible SCM334. In the target region spanning 722 kb, a strong candidate gene, the RNA-dependent RNA polymerase 3a (CaRDR3a), was identified. The whole-genome and transcriptome sequences of PG1-1 and SCM334 revealed a single Guanine (G) deletion in CaRDR3a first exon, causing a frameshift resulting in loss-of-function in SCM334. In addition, multiple loss-of-function alleles of CaRDR3a were identified in the reference sequences of C. annuum, C. chinense, and C. baccatum in the public database. Furthermore, virus-induced gene silencing of CaRDR3a in PG1-1 resulted in the loss of resistance against PepYLCIV. PG1-1 and the DNA marker developed in this study will be useful to breeders using Pepy-2 in their breeding programs.
Similar content being viewed by others
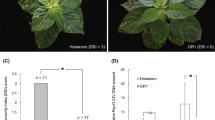
Avoid common mistakes on your manuscript.
Introduction
Pepper (Capsicum spp.) is planted on over 3.7 million ha, with global production of 42.3 million tons in 2019 (FAOSTAT 2019). There are five domesticated species in the Capsicum genus, with C. annuum being the most widely cultivated and consumed as a spice and vegetable worldwide (Bosland and Votava 2000). China, Mexico, Turkey, Indonesia, and India are the world’s top producers of peppers (total fresh and dried), accounting for ~ 70% of global production (FAOSTAT 2019). Nonetheless, several pathogens, including viruses, inhibit its production.
Pepper yellow leaf curl disease (PepYLCD) caused by whitefly (Bemisia tabaci)-transmitted begomovirus is among the major threats to the cultivation of pepper in many regions around the world, including the countries mentioned above (Devendran et al 2022). The genus Begomovirus (family Geminiviridae) contains 445 virus species, making it the most diverse group of plant-infecting viruses (ICTV 2020). In India, the outbreak of PepYLCD has resulted in severe yield loss of up to 90% of fruit production (Malathi et al. 2017). In Mexico, begomovirus infection resulted in complete crop failure due to dramatic yield loss (Morales-Aguilar et al. 2019). Due to similar restrictions, severe yield loss in pepper cultivation has been observed in Indonesia, and due to massive production losses caused by PepYLCD in 2005, pepper production in Southern Sumatra, Indonesia, was restricted due to the low possibility of more profitable crops being produced (De Barro et al. 2008; Sudiono et al. 2005). Because of their wide host range, efficient recombination ability, and ability to promote synergism in host plants, begomoviruses have become a global concern in pepper production (Devendran et al. 2022).
Many studies have screened begomovirus resistance sources from Capsicum germplasm because genetic resistance is the most effective and simplest approach to control PepYLCD (Adluri et al. 2017; Barchenger et al. 2019; García-Neria and Rivera-Bustamante 2011; Kenyon et al. 2014; Kumar et al. 2006; Mori et al. 2022; Rai et al. 2014; Retes-Manjarrez et al. 2019; Siddique et al. 2022; Singh et al. 2016; Srivastava et al. 2015, 2017; Thakur et al. 2019). However, despite the fact that these germplasms are critical for breeding begomovirus resistance in commercial pepper cultivars, no resistance genes for these peppers have yet been identified, which will help to speed up marker-assisted breeding. For the first time in peppers, we discovered pepy-1, a recessive resistance gene encoding the messenger RNA surveillance factor Pelota derived from BaPep-5 (C. annuum) (Koeda et al. 2021). Considering the strong demand from pepper producers and the crop breeding industry, the identification of multiple begomovirus resistance genes and those applications to marker-assisted breeding of new pyramiding pepper varieties possessing a robust and wide spectrum of resistance against various begomovirus species will have a significant impact as applied science.
In tomatoes (Solanum lycopersicum), which belong to the Solanaceae family with peppers, multiple resistance loci (Ty-1–Ty-6) against the monopartite tomato yellow leaf curl virus (TYLCV) have been identified in wild tomato accessions (Zamir et al. 1994; Agrama and Scott 2006; Anbinder et al. 2009; Gill et al. 2019; Ji et al. 2009a, b; Hutton and Scott 2014). Moreover, several Ty genes were cloned, and the molecular markers developed promoted resistance breeding significantly. The recessive resistance gene ty-5 identified from Solanum peruvianum encodes Pelota, which is the homolog of pepy-1 (Lapidot et al. 2015). Furthermore, the allelic Ty-1, Ty-3, and Ty-3a loci encode an RNA-dependent RNA polymerase (RDR), which was originally introgressed from Solanum chilense accessions (Zamir et al. 1994; Agrama and Scott 2006; Verlaan et al. 2013), and Ty-2 identified from Solanum habrochaites, encodes a nucleotide-binding leucine-rich repeat (NB-LRR) protein (Yamaguchi et al. 2018). Among these Ty genes, Ty-1, Ty-3, and Ty-3a are most widely used in the breeding of begomovirus resistance in F1 hybrid tomato cultivars.
In our preliminary analyses, we screened a C. annuum accession PG1-1 that was resistant to begomovirus. Capsicum is a non-model plant that has a large genome size of ~ 3.5 Gb (Kim et al. 2014; Qin et al. 2014). For efficient and accurate identification of the resistance gene, we conducted the forward-genetic analysis using restriction site-associated DNA sequencing (RAD-seq), whole-genome sequence combined with RNA-seq using an F2 and F3 population derived from a cross between PG1-1 with the begomovirus susceptible C. annuum accession SCM334. Moreover, we further conducted the reverse genetic analysis of the candidate gene using virus-induced gene silencing (VIGS) to reveal gene function in begomovirus resistance. According to our study, the dominant resistance of PG1-1 against bipartite begomoviruses pepper yellow leaf curl Indonesia virus (PepYLCIV) and pepper yellow leaf curl Aceh virus (PepYLCAV) is controlled by an RDR.
Materials and methods
Plant material
In this study, two C. annuum accessions, PG1-1 and SCM334, were used. The source of resistance in PG1-1 is derived from Capsicum pubescens, but the precise introgression process of resistance into C. annuum through several generations of back-cross followed by self-pollination has been vague. The F2 population derived from a cross between resistant PG1-1 and susceptible SCM334 was used for RAD-seq and the F3 population was used for further fine mapping. Plants were cultivated in a growth room with temperatures ranging from 23 to 30 °C and a photoperiod of 13-h light/11-h dark.
Begomovirus inoculation, detection, and quantification of viral DNA
In this study, the infectious clones for the bipartite begomoviruses PepYLCIV isolate BA_D1-1 (Accession number of GenBank for DNA A: LC051114, DNA B: LC314794) (Koeda et al. 2016, 2018) and PepYLCAV isolate BAPep-V2 (DNA A: LC387327, DNA B: LC387329) (Kesumawati et al. 2019) were employed (Koeda et al. 2018, 2021). Full details about the inoculation of pepper plants with begomoviruses and the detection and quantification of PepYLCIV and PepYLCAV viral DNA are provided in Koeda et al. (2017, 2018, 2021).
For graft-inoculation of PepYLCIV, the agroinfiltrated C. annuum accession No.218 plants were used as scions, and uninoculated PG1-1 and SCM334 plants were used as rootstocks. Disease symptoms were evaluated with the newly developed lateral branches of PG1-1 and SCM334 plants at 107 days after grafting for the first experiment and 57 days for the second experiment. Single inoculation experiments on PG1-1 and SCM334 plants with PepYLCAV were conducted by agroinfiltration, and disease symptoms were surveyed at 58 days post-inoculation (dpi). The F2 and F3 plants were graft-inoculated with PepYLCIV as described above, and symptom surveys were conducted at approximately 60 dpi. Young upper leaves were collected and stored at –80 °C until needed for DNA and/or RNA extraction.
DNA was extracted from pepper leaves using the Nucleon PhytoPure Kit (GE Healthcare, Little Chalfont, Buckinghamshire, UK). The DNA A components of PepYLCIV and PepYLCAV were detected by conventional PCR or quantified by qPCR. Statistical analysis was performed using the Tukey–Kramer test of Excel Toukei ver.7.0 or the Student’s t -test and a p < 0.05 was considered statistically significant. Supplementary Tables S1 and S2 list the primer sequences and PCR conditions.
Molecular mapping of the candidate gene by RAD-seq
As previously described, the RAD-seq libraries of 217 F2 individuals and their parents were constructed and sequenced with HiSeq X Ten (Illumina, Hercules, CA, USA) (Koeda et al. 2019). Data analysis was conducted as described in Koeda et al. (2021) using the whole-genome sequence of C. annuum (Zunla, ver.1) as a reference (Qin et al. 2014). The 1469 single nucleotide polymorphism (SNP) RAD tags were used to create the genetic linkage map, and linkage analyses were conducted by composite interval mapping (CIM) of R/qtl (Broman et al. 2003). Resistance-related SNPs were also identified by the Kruskal–Wallis test.
Whole-genome resequencing and fine mapping
Whole-genome resequencing of PG1-1 and SCM334 using NovaSeq 6000 (Illumina), and data analysis were conducted as described previously (Koeda et al. 2021). To narrow down the target region of the locus, SNP markers (S07_8122498 and S07_9240780) were developed for KBiosciences’ KASPar assay (LGC Genomics GmbH, Berlin, Germany), and recombinants were screened from 1146 F2 individuals according to the manufacturer’s protocol. Four recombinants, namely No.122, No.124, No.395, and No.1111, were screened and the recombination points of each F2 individual were identified using the additionally developed 11 high resolution melting (HRM) markers. The recombinants were self-pollinated to obtain F3 populations. HRM analysis was conducted as described previously (Koeda et al. 2021). Supplementary Table S3 and Table S4 list the primer sequences and PCR conditions used for fine mapping.
The co-dominant indel marker CaRDR Indel 2F/R was used to genotype the candidate gene in the F2 individuals, and the amplicons were subjected to electrophoresis using 8% polyacrylamide gels. Supplementary Table S3 and Table S2 list the primer sequences used in the analysis as well as the PCR conditions.
Analyses of the candidate gene
RNA extraction and reverse-transcription PCR (RT-PCR) were performed according to Koeda et al. (2021). RT-PCR was performed with CaRDR3a specific primer pairs using the cDNA template and KOD-plus Neo (Toyobo, Osaka, Japan) to amplify the open reading frame of the candidate gene. The PCR products were cloned into the TOPO vector (Thermo Fisher Scientific, MA, USA) and sequenced. Primer sequences used for PCR are listed in Supplementary Table S1.
The predicted amino acid sequences of RDR belonging to C. annuum, S. lycopersicum, Solanum tuberosum, Nicotiana tabacum, Arabidopsis (Arabidopsis thaliana), and rice (Oryza sativa) were aligned using the MUSCLE program (Edgar 2004). MEGA 7.0 (Kumar et al. 2016) was used to construct the phylogenetic tree by the neighbor-joining method, with 1,000 bootstrap replicates.
Expression analysis of candidate genes
RNA-seq of uninoculated and PepYLCIV-infected PG1-1 and SCM334 was conducted using NovaSeq 6000 (Illumina). Raw sequence reads were trimmed by Trimmomatic (v.0.39) (Bolger et al. 2014), mapped onto the whole-genome sequence of C. annuum (Zunla, ver.1) by STAR (v.2.7.1a) (Dobin et al. 2013), and quantified using RSEM (v.1.3.1.) (Li and Dewey 2011) to calculate gene expression as transcripts per million. De novo assembly of reads obtained by RNA-seq was conducted by Trinity (v.2.8.3.) (Grabherr et al. 2011).
According to Koeda et al. (2021), the expression of the candidate gene was analyzed by real-time quantitative reverse-transcription PCR (real-time qRT-PCR). The transcript level of the candidate gene was normalized relative to that of the CaActin (AY572427) reference gene to calculate the relative expression of the candidate gene by the 2−ΔΔCt method. Statistical analysis was performed using the Student’s t-test and a p < 0.05 was considered statistically significant. Supplementary Tables S1 and S2 list the primer sequences and PCR conditions utilized in real-time qRT-PCR.
Reverse genetic analysis by VIGS
According to Koeda et al. (2021), VIGS was conducted using the tobacco rattle virus (TRV) vectors. In brief, a partial coding sequence of CaRDR (200 bp) was amplified from PG1-1 with CaRDR VIGS F and R primers (Supplementary Table S1), and the amplicon was ligated with pTRV2. To avoid the effect of off-target genes, the SGN VIGS tool was used to design the pTRV2::CaRDR construct (Fernandez-Pozo et al. 2015).
Agrobacterium cultures containing pGreenII-p35S-PepYLCIV-DNA-A + B (Koeda et al. 2018) and pTRV1 with pTRV2::PDS, pTRV2::GFP, or pTRV2::CaRDR were mixed at a ratio of 1:1:1 for inoculation. The inoculated PG1-1 plants were maintained in a growth chamber controlled at 23 °C and 12-h light/12-h dark photoperiod. Disease symptoms were surveyed, and young upper leaves were collected at 18 dpi. The diagnosis of PepYLCIV and TRV was conducted using PepYLCIV uni 2F/R and TRV2 insert F/R primer pairs, respectively (Table S1). Statistical analysis was performed using the Student’s t-test and a p < 0.05 was considered statistically significant.
Results
PG1-1 is resistant to PepYLCIV and PepYLCAV
In the first graft-inoculation experiment, a symptomatic No.218 plant infected with PepYLCIV by agroinfiltration was used as a scion and a healthy PG1-1 plant was used as rootstock. The elongated No.218 scion showed typical yellowing symptoms in the newly developed mature leaves (Fig. 1a), whereas the elongated lateral branch from the PG1-1 rootstock showed no symptoms throughout the development until 107 days from grafting (Fig. 1a, Table 1). At 107 days after grafting, PepYLCIV was detected from No.218 scion and PG1-1 rootstock of the successfully grafted plants (n = 6) (Table 1). In the second graft-inoculation experiment, a symptomatic No.218 plant infected with PepYLCIV was used as a scion and a healthy PG1-1 or SCM334 plant was used as a rootstock. At 57 days after grafting, the elongated lateral branch from the SCM334 rootstock (n = 3) showed disease symptoms, whereas the PG1-1 plants (n = 24) showed no symptoms (Fig. 1b, Table 1). We continued the cultivation of grafted plants until the flowering and fruit-setting stage, but PG1-1 plants showed no symptoms, while SCM334 plants showed the same typical disease symptoms. At 57 days after grafting, PepYLCIV was detected in all successfully grafted SCM334 and PG1-1 plants. In the third inoculation experiment, we agroinfiltrated PepYLCAV into PG1-1 or SCM334 plants. PepYLCAV is a recombinant begomovirus of PepYLCIV that exhibit higher virulence compared to PepYLCIV (Kesumawati et al. 2019; Koeda et al. 2021). At 39 dpi and 58 dpi, PepYLCAV-infected SCM334 plants (n = 3) showed even heavier symptoms than PepYLCIV (Fig. 1c, Table 1). In contrast, PepYLCAV-infected PG1-1 plants (n = 8) showed slight symptoms with yellowing spots in the upper leaves at 39 dpi, but eventually recovered in the newly developed leaves and no symptoms were observed at 58 dpi (Fig. 1c, Table 1).
PG1-1 and SCM334 plants with single inoculations of pepper yellow leaf curl Indonesia virus (PepYLCIV) and pepper yellow leaf curl Aceh virus (PepYLCAV). a PG1-1 plant (rootstock) was grafted with PepYLCIV-infected No.218 (Scion). b SCM334 and PG1-1 plants (rootstock) infected with PepYLCIV through grafting with No.218 (Scion). c PepYLCAV-infected SCM334 and PG1-1 plants by agroinfiltration. Pictures were taken at a 107 days post-grafting, b 57 days post-grafting, and c 58 post-agroinfiltration (dpi)
Plants infected with begomovirus by graft-inoculation and agroinfiltration were randomly selected for PepYLCIV or PepYLCAV DNA quantification. The accumulation of begomoviral DNA in PG1-1 plants was significantly lower than that in No.218 or SCM334 plants (Fig. 2). These results suggest that PG1-1 is resistant to PepYLCIV and PepYLCAV by restricting the accumulation of viral DNA.
Quantification of PepYLCIV and PepYLCAV DNA in infected PG1-1, SCM334, and No.218 plants. Accumulation of viral DNA was quantified a in PepYLCIV-infected plants at 107 days post-grafting, b in PepYLCIV-infected plants at 57 days post-grafting, and c in PepYLCAV-infected plants at 58 dpi from agroinfiltration. Biological replicates are indicated on each bar. Data represent mean ± standard deviation (SD). Asterisk indicates significant differences among means (Student’s t-test, p < 0.05)
Genetic mapping of begomovirus resistance gene
Because graft-inoculation is a reliable method to deliver begomovirus without any inoculation escapes (Table 1) (Anaya-López et al. 2003; Vanderschuren et al. 2012; Koeda et al. 2018, 2021; Mori et al. 2022), we graft-inoculated PepYLCIV to further analyze F1, F2, and F3 populations derived from a cross between PG1-1 and SCM334. Unless mentioned, all plants used for phenotyping were positive for PepYLCIV infection by PCR-based diagnosis. Because F1 plants (n = 5) were also resistant to PepYLCIV, the resistance of PG1-1 seems to be a dominant trait. When we graft-inoculated PepYLCIV to parental SCM334 and PG1-1 plants, susceptibility and resistance were distinguishable at 57 days from grafting and the same phenotype was observed until the flowering and fruiting stage (Table 1). Thus, we evaluated the resistance of each PepYLCIV-infected F2 individual at approximately 60 days from grafting. The F2 individuals (n = 217) showed the following phenotypic segregation with severe distortion: n = 216, resistant; n = 1, susceptible. The resistant individuals showed no symptoms same as PG1- and the single susceptible F2 individual showed typical leaf yellowing symptoms identical to SCM334.
The 1469 SNPs obtained from RAD-seq were used for linkage analysis of the F2 population (n = 217). The linkage map consisted of 12 linkage groups, which was equivalent to the chromosome number of pepper (C. annuum), and the average distance between DNA markers was 1.9 cM (Supplementary Fig. S1a). The linkage analysis performed by CIM detected a single peak on chromosome 7 with the highest logarithm of the odds (LOD) score above 1500, although the peak was below the threshold because of the severe segregating distortion (Supplementary Fig. S1b). Analysis of resistance-linked marker by the Kruskal–Wallis test detected S07_8329905 (p-value 1.25E-47), which perfectly co-segregated with the phenotype in all 217 F2 individuals. Because the phenotype did not match with genotype for a single individual of the S07_8020814 and S07_9542047 markers, the candidate region was delimited to a 1.52 Mb region (Fig. 3). This single locus was named Pepper yellow leaf curl disease virus resistance 2 (Pepy-2), considering its dominant nature. Because only a single susceptible individual was homozygous for the SCM334 alleles among 217 F2 individuals, it seemed that recombination was severely suppressed in this genomic region.
Physical mapping of the candidate gene. The candidate gene was mapped between S07_8122498 and HRM 8,844,414 markers, within a physical distance of 722 kb on chromosome 7. Homozygous dominant (PG1-1 allele), heterozygous, and homozygous recessive (SCM334 allele) genotypes are represented by red, gray, and blue bars, respectively. Names of DNA markers are indicated above the horizontal line, and the numbers above the marker names indicate the numbers of recombinant plants. The F3 populations derived from the F2 recombinants No.122 and No.124 were genotyped using the S07_9240780 marker. PepYLCIV resistance phenotypes are shown for each segregating genotype of the F3 population: RR; homozygous dominant, RS; heterozygous, SS; homozygous recessive. R indicates resistance and N.C. indicates phenotyping was not conducted
We prepared an additional F2 population (n = 1,146) to assess the suppression of recombination in the genomic region containing the Pepy-2 locus and to further narrow down the candidate region. Since only two individuals were homozygous for the SCM334-genotype among all 1146 F2 individuals tested, it was reconfirmed that the susceptible genotype for the candidate region seemed to be rarely obtained because of the recombination suppression. Randomly selected individuals for PG1-1 genotypes (n = 4) and heterozygotes (n = 8) were resistant to PepYLCIV by graft-inoculation at approximately 60 days from grafting, whereas individuals for SCM334 genotypes (n = 2) were susceptible; the genotype perfectly co-segregated with the phenotype. To narrow down the candidate region, two additional KASP markers, S07_8122498 and S07_9240780 were developed, and four recombinants (No.122, No.124, No.395, and No.1111) were screened from the newly prepared F2 population (n = 1,146) (Fig. 3). To detect recombination points, 11 HRM SNP markers were developed, based on the comparison of whole-genome sequences of PG1-1 and SCM334. F2 individual No.395 had recombination between HRM 8,910,283 and HRM 9,039,615 markers, No.1111 had recombination between HRM 8,844,414 and HRM 8,910,283 markers, No.122 had recombination between HRM 8,810,113 and HRM 8,844,414 markers, and No.124 had recombination between S07_8122498 and HRM 8,128,285 markers. Graft-inoculated F2 recombinants No.122, No.124, No.395, and No.1111 were resistant to PepYLCIV. Because the annotated gene information inferred that No.122 and No.124 were the most informative recombinants, these recombinants were self-pollinated to obtain F3 populations.
Genotyping using a DNA marker S07_9240780 revealed that the F3 population (n = 237) derived from the recombinant No.124 segregated into PG1-1 genotypes (n = 80) and heterozygotes (n = 157) without any SCM334 genotypes. This result also showed that recombination is severely suppressed in this genomic region, which was consistent with the results observed in the F2 populations. Thus, we could not examine the relationship between genotype and phenotype for the segregating region of the F3 population derived from recombinant No.124. Genotyping using the DNA marker S07_9240780 revealed that the F3 population (n = 1,530) derived from the recombinant No.122 segregated into PG1-1 genotypes (n = 260), SCM334 genotypes (n = 512), and heterozygotes (n = 758). At approximately 60 days from grafting, randomly selected individuals for PG1-1 genotypes (n = 15), SCM334 genotypes (n = 26), and heterozygotes (n = 22) were all resistant to PepYLCIV (Fig. 3). These results indicated that the candidate gene was located within the genomic region of 8,122–8,844 k on chromosome 7 which was between S07_8122498 and HRM 8,844,414.
Analysis of the annotation data of the reference genome of Zunla (Qin et al. 2014) revealed that the candidate region of 722 kb contained 17 candidate genes (Table 2). Comparison of re-sequenced data clarified that there are nonsynonymous substitutions for all the candidate genes in PG1-1 compared to SCM334. RNA-seq analysis of uninoculated and PepYLCIV-infected PG1-1 and SCM334 plants showed that there were no significant differences in gene expression for Capana07g000158, Capana07g000160, Capana07g000163, Capana07g000164, Capana07g000166, Capana07g000167, Capana07g000169, Capana07g000170, Capana07g000171, and Capana07g000176. Although significant differences were detected between PG1-1 and SCM334 for Capana07g000165, Capana07g000173, and Capana07g000175, no correlation with PepYLCIV resistance was observed. Whereas significant differences correlating with PepYLCIV resistance were detected for Capana07g000161 encoding pentatricopeptide repeat-containing protein, Capana07g000162 encoding F-box protein SKIP1-like, Capana07g000168 encoding probable RNA-dependent RNA polymerase (RDR), and Capana07g000174 encoding uncharacterized protein. Because Ty-1/Ty-3 resistance gene to TYLCV in tomato encodes DFDGD-Class RDRγ (Verlaan et al. 2013), Capana07g000168 seemed to be the strong candidate for conferring resistance to PepYLCIV in PG1-1.
RNA-dependent RNA polymerase is a strong candidate for the resistance gene
De novo assembly of RNA-seq obtained reads from PG1-1 and SCM334 inferred that Capana07g000167 and Capana07g000168 are single genes instead of two different genes. Genome-wide analysis of RDRs in pepper (C. annuum) identified six hypothetical CaRDRs (CaRDR1, CaRDR2, CaRDR3a, CaRDR3b, CaRDR5, and CaRDR6) (Qin et al. 2018). Isolation of the full-length sequences for the transcript from PG1-1 and SCM334 clarified that CaRDR3a, located on the target region of chromosome 7, consist of 19 exons (Fig. 4a). Single deletion of Guanine (G) was detected in the first exon, which caused a frameshift and led to a truncated protein of 105 amino acids in SCM334 (Fig. 4b). Moreover, additional insertion of 2 bp causing a frameshift and a nonsense mutation were detected in the 8th exon (Fig. 4a). In contrast, a protein of 1016 amino acids was predicted for PG1-1, which showed high similarity with the RDR (Ty-1/Ty-3) of tomato. Phylogenetic analysis of RDRs from different plant species showed that α-clade RDRs and γ-clade RDRs clustered independently (Fig. 5) In the phylogenetic tree, CaRDR3a of PG1-1 showed high sequence similarity with the amino acid sequences of RDR from tomato (SlRDR3), potato (StRDR3), and tobacco (NtRDR3). Whereas RDRs of rice and Arabidopsis constituted independent clades from CaRDR3a.
Molecular genetic identification of CaRDR3a as the candidate gene responsible for the PepYLCIV resistant phenotype of PG1-1. a Schematic diagram of CaRDR3a in SCM334 and PG1-1. Exons (closed black boxes) were predicted from the corresponding cDNA sequences. Single deletion of Guanine (G) was detected in the first exon, which caused a frameshift in SCM334. b The amino acid sequence of CaRDR3a of PG1-1 (C. annuum) and SCM334 (C. annuum) was aligned with those of its homologs in Solanum lycopersicum (TYLCV-resistant alleles of LA1969 [Ty-1], LA2779 [Ty-3], and susceptible allele of MM) using Clustal Omega. Black underbars represent the DFDGD motif
Phylogenetic analysis of CaRDR3a with RDRs of other plant species. A phylogenetic tree was constructed using the neighbor-joining method. Bootstrap values are indicated at the nodes (based on 1000 replicates). The branch lengths are proportional to the number of nucleotide changes, as indicated by the scale bar (0.05 substitutions per site)
A comparison of CaRDR3a sequences obtained from whole-genome sequences in the public database revealed that all the C. annuum, including ECW, Chiltepin, Zunla, and SF, also had a deletion of G in the 1st exon (Fig. S2). C. annuum CM334 and UCD10X not only had a deletion of G in the 1st exon but also a 34 bp deletion which resulted in missing the start codon (ATG). Interestingly, a Capsicum chinense accession PI159236 had a single base substitution from Thymine (T) to G in the 2nd exon, which raised a premature termination codon. Moreover, a Capsicum baccatum accession PBC81 had a single base substitution from T to G in the 8th exon, which raised a premature termination codon. All the analyzed genomic regions of CaRDR3a for the above-mentioned capsicums had more than three putative loss-of-function mutations. These results inferred that only PG1-1 had putative functional CaRDR3a.
From comparing the genomic sequence of CaRDR3a for PG1-1, SCM334, and other capsicums in the public database, only PG1- had 27 bp deletion in the thirteenth intron (Fig. 6a). We developed a co-dominant marker system based on PCR using the Indel marker (Fig. S3). Electrophoresis of the PCR amplicons detected a single fragment of 117 bp in PG1-1, a single fragment of 144 bp in SCM334, and both fragments in F1 individual (Fig. 6b). Genotyping all 235 F2 individuals with this Indel marker (CaRDR Indel 2F/R) revealed that the genotype perfectly co-segregated with PepYLCIV resistance in the F2 population (Table 3).
DNA marker analysis. a Schematic diagram of CaRDR3a in SCM334 and PG1-1. Exons (closed white boxes), and the location of the 27 bp deletion at the 13th intron of PG1-1 are shown. The annealing positions of CaRDR Indel 2F and R primers are indicated. b Polyacrylamide gel showing the results of CaRDR3a genotyping in PG1-1, SCM334, F1, and F2 individuals. M represents the 100 bp DNA ladder
Expression analysis and VIGS of CaRDR3a
Because it was revealed that CaRDR3a was a single gene instead of two different genes Capana07g000167 and Capana07g000168, we conducted gene expression analysis of RNA-seq using a modified gff3 file updated with the collect annotation data (Supplementary file 1). The expression of CaRDR3a was significantly higher in PG1-1 than SCM334 for uninoculated and PepYLCIV-infected plants (Fig. 7a).
Expression analysis and virus-induced gene silencing (VIGS) of CaRDR3a. a RNA-seq analysis of CaRDR3a in the leaves of uninoculated or PepYLCIV-infected SCM334 and PG1-1 plants. Effect of VIGS on b PepYLCIV symptoms in PG1-1, c CaRDR3a expression levels, and d PepYLCIV DNA levels at 18 dpi. Biological replicates are indicated in the figures. Data represent mean ± SD. Different letters indicate significant differences between means (Tukey–Kramer test, p < 0.05). Asterisk indicates significant differences among means (Student’s t-test, p < 0.05)
Linkage analysis, sequencing of the genome and transcript sequences, and gene expression analysis all supported that CaRDR3a is conferring resistance to PepYLCIV in PG1-1. We conducted VIGS of CaRDR3a in PG1-1 to analyze the function of CaRDR3a in PepYLCIV resistance. VIGS is a powerful tool for reverse genetics in pepper, which is recalcitrant to transformation (Chung et al. 2004). To design specific VIGS construct for CaRDR3a without any off-target results, the SGN VIGS tool was used, and Blast analysis was conducted. Because PepYLCIV and TRV need to be mixed infected and gene silencing is more effective in small young plants, we agroinfiltrated two viruses to cotyledons of PG1-1.
At 18 dpi, photobleaching caused by the silencing of the phytoene desaturase (PDS) gene was observed in PG1-1 plants infected with PepYLCIV and TRV2::PDS (Fig. 7b). PepYLCIV and TRV mixed infected plants were selected according to PCR-based diagnosis and used for further analysis. Quantification of CaRDR3a expression and the accumulating PepYLCIV DNA were conducted in PG1-1 plants co-infected with PepYLCIV and TRV harboring partial sequences of the green fluorescent protein (GFP) gene or CaRDR3a. The expression of CaRDR3a was significantly lower and the accumulation of PepYLCIV DNA was significantly higher in the young upper leaves of plants inoculated with TRV2::CaRDR (n = 11) compared to that in plants inoculated with TRV2::GFP (n = 4) (Fig. 7c, d). Moreover, TRV2::CaRDR-inoculated plants showed typical PepYLCIV symptoms (Fig. 7b). From these results, we concluded that the begomovirus resistance gene Pepy-2 in PG1–1 is most likely CaRDR3a.
Discussion
Begomovirus caused PepYLCD has been a major limitation to pepper production in many countries around the world (Devendran et al. 2022). Therefore, marker-assisted breeding of begomovirus resistance is strongly required, but pepy-1 derived from BaPep-5 (C. annuum) is the only begomovirus resistance gene cloned to date in peppers (Koeda et al. 2021). In this study, we mainly utilized graft-inoculation of begomovirus to pepper plants, which is a reliable method to deliver begomovirus without any inoculation escapes (Anaya-López et al. 2003; Vanderschuren et al. 2012; Koeda et al. 2018, 2021; Mori et al. 2022). It should be noted that all plants used for analyses were verified for the infection of inoculated begomovirus by PCR. Moreover, we carefully evaluated the resistance and susceptible phenotypes in the laboratory conditions under the constant climate and without any unexpected infection of other viruses or pathogens, which is difficult to control in the field of Southeast Asia, where PepYLCIV and PepYLCAV originate. Although the DNA polymorphisms, even with a non-model plant species with a relatively large genome size such as Capsicum, can now be easily obtained by high-throughput sequencing technology, accurate phenotyping of the traits needs deliberate and laborious work. Uniform inoculation and cultivation methods are significantly important to avoid miss-phenotyping of resistance and susceptible traits, which will critically affect the successful genetic mapping of the resistance gene via linkage analysis. Our linkage analysis using the F2 and F3 segregating populations derived from PG1-1 narrowed down the candidate region on chromosome 7 to 722 kb which contained 17 candidate genes (Fig. 3; Table 2). Although we could not completely rule out the possibility that other candidate genes from RDR are also contributing to the begomovirus resistance in PG1-1, sequence analysis of CaRDR3a derived from multiple capsicums within and among the species, reverse genetic analysis by VIGS, and previous research regarding TYLCV resistance in tomato conferred by Ty-1/Ty-3 encoding RDR (Verlaan et al. 2013), in combination provides a solid ground for the conclusion that CaRDR3a is involved in begomovirus resistance in PG1-1.
Several studies have conducted genetic mapping of begomovirus resistance genes in peppers. Thakur et al. (2020) reported that the chili leaf curl virus disease resistance gene of a C. annuum accession S-343 is linked to a DNA marker located on chromosome 6. In our previous study, we mapped pepy-1 from BaPep-5 (C. annuum) on chromosome 5 (Koeda et al. 2021). More recently, we also mapped C. chinense-derived quantitative trait loci (QTLs) related to PepYLCIV resistance on chromosomes 3, 4, and 11 (Mori et al. 2022). Meanwhile, Siddique et al. (2022) mapped the QTLs for pepper yellow leaf curl virus resistance in LP97 (C. annuum) on chromosomes 1, 7, and 12. Because our candidate genes located within the genomic region of 8122–8844 k (722 kb) on chromosome 7 in this study did not overlap with the candidate genes for the major QTL peplcv-7 reported by Siddique et al. (2022) (Table 2; Fig. 3), peplcv-7 might be different resistance gene from Pepy-2. Further genetic mapping and identification of additional begomovirus resistance genes will be significantly important for the marker-assisted breeding of begomovirus resistance in peppers.
In the present study, severe segregating distortions in the F2 and F3 populations derived from crossing between PG1-1 and SCM334 were observed; the appearance of susceptible genotype (pepy-2/pepy-2) was restricted by the recombination suppression (Table 3; Fig. 3). Recombination suppression is a common phenomenon in the genomic regions introgressed from wild tomato species into cultivated tomatoes. Suppression of recombination was reported in the F2 populations derived from interspecific crosses between S. lycopersicum and S. peruvianum, which were used for cloning the Mi-1 gene conferring resistance to root-knot nematodes (Kaloshianet al. 1998). Also, the chromosomal rearrangements in the S. chilense introgression caused the recombination suppression which hampered to precisely locating Ty-1 (Verlaan et al. 2011). Moreover, similar recombination suppression was observed in the genomic region where Ty-2 was introgressed from S. habrochaites, and no progeny with homozygous of the S. lycopersicum alleles were identified in the genomic region in the interval of markers TG36 and C2_At3g52090 (Yang et al. 2014). The source of begomovirus resistance in PG1-1 derives from C. pubescens. There are five domesticated species in the genus Capsicum, and C. pubescens is genetically furthest away from C. annuum cultivated most widely around the world (Carrizo García et al. 2016). The introgressed DNA fragment harboring the Pepy-2 derived from C. pubescens might be causing the segregating distortion, but the underlying mechanism is unknown in this study. As Yang et al. (2014) discussed, there are at least two reasons to reduce the size of introgressed chromosome segment when considering the use in practical breeding. First is the possible linkage drag. Secondly, introgression of the large chromosome segment derived from wild species can hamper combining important genes in cis. So, far, we have not observed any obvious linkage drag related to the introgression of the DNA fragment harboring the Pepy-2, but to reduce the introgressed segment, further screening of recombinants from a larger population using the DNA marker developed on the resistance gene CaRDR3a and the multiple DNA markers developed at the nearby genomic region is needed.
In plants, siRNAs are typically derived from long double-stranded RNA molecules synthesized by RDR, which is considered a fundamental element in RNA silencing pathways and participates in antiviral defense mechanisms. RDRs are defined by the presence of a conserved RNA-dependent RNA polymerase catalytic domain, and there are three major clades of eukaryotic RDRs (RDRα, RDRβ, and RDRγ) (Willmann et al. 2011). The RDRβ is conserved only among fungi and a few animals (Zhang et al. 2014). The model plant A. thaliana possesses six identifiable RDRs, which are classified into RDRα and RDRγ (Wassenegger and Krczal 2006). Arabidopsis RDRα (RDR1, RDR2, and RDR6) share the C-terminal canonical catalytic DLDGD motif of eukaryotic RDRs and have orthologs in many plant species (Wassenegger and Krczal 2006). However, it was demonstrated that viral siRNAs in geminivirus-infected Arabidopsis do not require the functional RDR1, RDR2, or RDR6 (Aregger et al. 2012). Arabidopsis also has three RDRγ (RDR3, RDR4, and RDR5; also called RDR3a–RDR3c), which share an atypical DFDGD amino acid motif in the catalytic domain, but the function of these genes has not yet been described in this plant species. The function of RDRγ especially in begomovirus resistance, is well understood in tomatoes. The Ty-1, Ty-3, and Ty-3a loci are allelic originating from different Solanum chilense accessions (Zamir et al. 1994; Agrama and Scott 2006), and these allelic loci encode a DFDGD-Class RDRγ (Verlaan et al. 2013). Our present study showed that the PepYLCIV and PepYLCAV resistance gene of PG1-1 encodes CaRDR3a, which also has a DFDGD motif and showed high sequence similarity with the amino acid sequences of RDRγ from tomato (Ty-1 and Ty-3), potato, and tobacco (Figs. 4b and 5). In tomatoes, whether amino acid sequence differences between Ty-1 (resistance) and ty-1 (susceptible) protein or just those in transcriptional expression levels or a combination of both, are the cause of resistance remains to be investigated (Verlaan et al. 2013). A comparison of CaRDR3a sequences among PG1-1, SCM334, and many other accessions from different Capsicum species in our study inferred that difference in the amino acid sequence is the cause of resistance in Capsicum (Fig. 4b; Supplementary Fig. S2). Since it is reported that Ty-1 confers resistance to TYLCV and to a bipartite begomovirus tomato severe rugose virus by increasing cytosine methylation of viral genomes (Butterbach et al. 2014), it was suggested that PepYLCIV and PepYLCAV resistance in PG1-1 is the result of restricted replication of begomoviral DNA by transcriptional gene silencing pathway by the role of CaRDR3a.
A recent study using transgenic plants showed that RDRγ is also related to the development and yield-related traits. Jha et al. (2021) showed that OsRDR3-overexpressing rice (O. sativa) and tobacco (N. tabacum) plants grew vigorously, whereas the growth of OsRDR3 and OsRDR4 knockdown lines were stunted and did not survive beyond vegetative growth. Analysis of whole-genome sequences of C. annuum, C. chinense, and C. baccatum accessions in the public database revealed that those accessions possessed the putative loss-of-function alleles of CaRDR3a (Fig. S2). Interestingly, those multiple loss-of-function alleles of C. annuum, C. chinense, and C. baccatum probably arose through independent mutational events during the domestication. Because it is difficult to imagine that those C. annuum, C. chinense, and C. baccatum accessions used for the whole-genome sequencing projects show visible growth or developmental defects like the OsRDR3 and OsRDR4 knockdown lines of rice, loss-of-function mutations in CaRDR3a seems to have no negative effects to the growth and development of pepper plants. The disease resistance genes are frequently lost in crops during domestication from their wild relatives (Mammadov et al. 2018). Although we did not observe any visible penalty caused by pleiotropic effects of the putative functional CaRDR3a (Pepy-2) in PG1-1 and its progenies, further precise analysis using the backcrossed generations will be needed for the conclusion.
PG1-1 harboring Pepy-2 showed no symptoms to PepYLCIV. In contrast, PepYLCAV-infected plants showed slight symptoms with yellowing spots in the upper leaves at 39 dpi, but eventually recovered in the newly developed leaves and no symptoms were observed at 58 dpi (Fig. 1c, Table 1). The slight or mild symptoms are sometimes observed in the interaction between some begomovirus with pepper and tomato plants possessing resistance genes, and in some cases, the recovering phenomenon was observed. The pepy-1 derived from BaPep-5 (C. annuum) is a homolog of ty-5 and is a recessive resistance gene encoding the messenger RNA surveillance factor Pelota (Koeda et al. 2021). BaPep-5 plants were highly resistant to PepYLCIV without showing any disease symptoms, whereas they showed mild symptoms to a more virulent PepYLCAV in the early infection stage, but gradually recovered in the newly developed leaves and symptoms almost disappeared in the late growing stage (Koeda et al. 2021). In commercial tomato cultivars, the Ty-1/Ty-3/Ty-3a resistance loci encoding RDRγ is most widely introgressed. The Ty-1/Ty-3/Ty-3a conferred resistance is effective against Israel and Mild strains of TYLCV that have distribution in many regions around the world (Verlaan et al. 2013; Mabvakure et al. 2016). However, Ty-1/Ty-3/Ty-3a conferred resistance is only partially effective against some of the begomovirus species with higher virulence, and tomato plants show slight to mild symptoms when infected with these begomoviruses (Prasanna et al. 2015; Torre et al. 2018; Koeda et al. 2020). Because begomovirus contains more than 400 species and recombination is frequently observed between begomoviruses, one of the driving forces for the emergence of the new virus with higher virulence or broader host range, control of begomovirus caused disease by the introgression of a single resistance gene seems to be sometimes difficult.
Several studies have shown that pyramiding multiple Ty genes in a single tomato plant results in robust resistance to begomoviruses (Mejía et al. 2010; Gil et al. 2019; Kenyon et al. 2019; Yan et al. 2021). In many of the pepper-producing countries suffering from the damage caused by PepYLCD, multiple begomovirus species are isolated and mixed infection is frequently observed. For example, at least seven begomovirus species accompanied by five different betasatellite were isolated from pepper plants in India, pepper huasteco yellow vein virus and pepper golden mosaic virus are reported as predominant begomovirus species with frequent mixed infection in Mexico, and PepYLCIV and PepYLCAV as predominant pepper-infecting begomoviruses in Indonesia (Rentería-Canett et al. 2011; Kumar et al. 2015; Kesumawati et al. 2019; Koeda et al. 2016, 2021). In these areas, using a single resistance gene for the complete control of PepYLCD will be difficult, because of significantly high begomovirus infection pressure. Currently, we are setting up and proceeding with several experiments to further evaluate the begomovirus resistance in PG1-1. The first is to evaluate the resistance with whitefly transmission of begomoviruses under the field condition in different geographic areas where PepYLCD is causing the problem in pepper productions. The second is to evaluate the gene pyramiding effect of pepy-1 and Pepy-2 resistance genes in a single pepper plant against single and mixed infections of begomoviruses. Further studies will provide a better approach to controlling PepYLCD in pepper production.
Availability of data and material
Accession numbers for each of the gene sequences referred to in this work are as follows: mRNA sequences of PG1-1 (LC642629) and SCM334 (LC642630).
Code availability
Not applicable.
References
Adluri PK, Baldoldiya GM, Nath PD (2017) Screening of Bhut Jolokia (Capsicum chinense Jacq.) germplasm of North East India against chili leaf curl virus. Int J Pure Appl Biosci 5:1189–1196
Agrama HA, Scott JW (2006) Quantitative trait loci for Tomato yellow leaf curl virus and Tomato mottle virus resistance in tomato. J Am Soc Hortic Sci 131:267–272
Anaya-López JL, Torres-Pacheco I, González-Chavira M et al (2003) Resistance to geminivirus mixed infections in Mexican wild peppers. HortScience 38:251–255
Anbinder I, Reuveni M, Azari R et al (2009) Molecular dissection of Tomato leaf curl virus resistance in tomato line TY172 derived from Solanum peruvianum. Theor Appl Genet 119:519–530
Aregger M, Borah BK, Seguin J et al (2012) Primary and secondary siRNAs in geminivirus-induced gene silencing. PLoS Pathog 8:e1002941
Barchenger DW, Yule S, Jeeatid N, Lin S, Wang Y, Lin T, Chan Y, Kenyon L (2019) A novel source of resistance to Pepper yellow leaf curl Thailand virus (PepYLCThV) (Begomovirus) in chile pepper. HortScience 54:2146–2149
Bolger AM, Lohse M, Usadel B (2014) Trimmomatic: a flexible trimmer for Illumina sequence data. Bioinformatics 30:2114–2120
Bosland PW, Votava EJ (2000) Peppers: vegetable and spice capsicums. CABI Publishing, New York
Broman KW, Wu H, Sen Ś, Churchill GA (2003) R/qtl: QTL mapping in experimental crosses. Bioinformatics 19:889–890
Butterbach P, Verlaan MG, Dullemans A et al (2014) Tomato yellow leaf curl virus resistance by Ty-1 involves increased cytosine methylation of viral genomes and is compromised by cucumber mosaic virus infection. Proc Natl Acad Sci U S A 111:12942–12947
Carrizo García C, Barfuss MH, Sehr EM et al (2016) Phylogenetic relationships, diversification and expansion of chili peppers (Capsicum, Solanaceae). Ann Bot 118:35–51
Chung E, Seong E, Kim YC et al (2004) A method of high frequency virus-induced gene silencing in chili pepper (Capsicum annuum L. cv. Bukang). Mol Cells 17:377–380
De Barro PJ, Hidayat SH, Frohlich D, Subandiyah S, Ueda S (2008) A virus and its vector, pepper yellow leaf curl virus and Bemisia tabaci, two new invaders of Indonesia. Biol Invasions 10:411–433
Devendran R, Kumar M, Ghosh D et al (2022) Capsicum-infecting begomovirus as global pathogens: host-virus interplay, pathogenesis, and management. Trends Microbiol 30:170–184. https://doi.org/10.1016/j.tim.2021.05.007
Dobin A, Davis CA, Schlesinger F et al (2013) STAR: ultrafast universal RNA-seq aligner. Bioinformatics 29:15–21
Edgar RC (2004) MUSCLE: multiple sequence alignment with high accuracy and high throughput. Nucleic Acids Res 32:1792–1797
FAOSTAT (2019). http://www.fao.org/faostat/en/#data/. Accessed 25 Feb 2022
Fernandez-Pozo N, Rosli HG, Martin GB, Mueller LA (2015) The SGN VIGS tool: user-friendly software to design virus-induced gene silencing (VIGS) constructs for functional genomics. Mol Plant 8:486–488
García-Neria MA, Rivera-Bustamante RF (2011) Characterization of geminivirus resistance in an accession of Capsicum chinense Jacq. Mol Plant Microbe Interact 24:172–182
Gill U, Scott JW, Shekasteband R et al (2019) Ty-6, a major begomovirus resistance gene on chromosome 10, is effective against Tomato yellow leaf curl virus and Tomato mottle virus. Theor Appl Genet 132:1543–1554
Grabherr M, Haas B, Yassour M et al (2011) Full-length transcriptome assembly from RNA-Seq data without a reference genome. Nat Biotechnol 29:644–652
Hutton SF, Scott JW (2014) Ty-6, a major begomovirus resistance gene located on chromosome 10. Rep Tomato Genet Coop 64:14–18
International Committee on Taxonomy of Viruses (2020) https://talk.ictvonline.org/taxonomy/. Accessed 16 Sep 2021
Jha V, Narjala A, Basu D et al (2021) Essential role of γ-clade RNA-dependent RNA polymerases in rice development and yield-related traits is linked to their atypical polymerase activities regulating specific genomic regions. New Phytol 232:1674–1691
Ji Y, Scott JW, Schuster DJ (2009a) Toward fine mapping of the Tomato yellow leaf curl virus resistance gene Ty-2 on chromosome 11 of tomato. HortScience 44:614–618
Ji Y, Scott JW, Schuster DJ, Maxwell DP (2009b) Molecular mapping of Ty-4, a new tomato yellow leaf curl virus resistance locus on chromosome 3 of tomato. J Am Soc Hortic Sci 134:281–288
Kaloshian I, Yaghoobi J, Liharska T et al (1998) Genetic and physical localization of the root-knot nematode resistance locus mi in tomato. Mol Gen Genet 257:376–385
Kenyon L, Kumar S, Tsai WS, Hughes Jd’A, (2014) Virus diseases of peppers (Capsicum spp.) and their control. In: Loebenstein G, Katis N (eds) Advances in virus research, vol 90. Academic Press, Cambridge, pp 297–254
Kenyon L, Hanson PM, Nguyen TLH et al (2019) The benefit of combining different Ty-genes for resistance to tomato leaf curl begomoviruses. Acta Hortic 1257:15–22
Kesumawati E, Okabe S, Homma K, Fujiwara I, Zakaria S, Kanzaki S, Koeda S (2019) Pepper yellow leaf curl Aceh virus: a novel bipartite begomovirus isolated from chili pepper, tomato, and tobacco plants in Indonesia. Arch Virol 164:2379–2383
Kim S, Park M, Yeom SI et al (2014) Genome sequence of the hot pepper provides insights into the evolution of pungency in Capsicum species. Nature Genet 46:270–278
Koeda S, Kesumawati E, Tanaka Y, Hosokawa M, Doi M, Kitajima A (2016) Mixed infection of begomoviruses on pepper plants at Northern Sumatra, Indonesia. Trop Agric Dev 60:59–64
Koeda S, Homma K, Tanaka Y, Kesumawati E, Zakaria S, Kanzaki S (2017) Highly efficient agroinoculation method for tomato plants with Tomato yellow leaf curl Kanchanaburi virus. Hort J 86:479–486
Koeda S, Homma K, Tanaka Y, Onizaki D, Kesumawati E, Zakaria S, Kanzaki S (2018) Inoculation of capsicums with Pepper yellow leaf curl Indonesia virus by combining agroinoculation and grafting. Hort J 87:364–371
Koeda S, Sato K, Saito H, Nagano AJ, Yasugi M, Kudoh H, Tanaka Y (2019) Mutation in the putative ketoacyl-ACP reductase CaKR1 induces loss of pungency in Capsicum. Theor Appl Genet 132:65–80
Koeda S, Fujiwara I, Oka Y, Kesumawati E, Zakaria S, Kanzaki S (2020) Ty-2 and Ty-3a conferred resistance are insufficient against tomato yellow leaf curl Kanchanaburi virus from Southeast Asia in single or mixed infections of tomato. Plant Dis 104:3221–3229
Koeda S, Onouchi M, Mori N, Pohan NS, Nagano AJ, Kesumawati E (2021) A recessive gene pepy-1 encoding Pelota confers resistance to begomovirus isolates of PepYLCIV and PepYLCAV in Capsicum annuum. Theor Appl Genet 134:2947–2964
Kumar S, Kumar S, Singh M, Singh AK, Rai M (2006) Identification of host plant resistance to pepper leaf curl virus in chilli (Capsicum species). Sci Hort 110:359–361
Kumar RV, Singh AK, Singh AK et al (2015) Complexity of begomovirus and betasatellite populations associated with chilli leaf curl disease in India. J Gen Virol 96:3143–3158
Kumar S, Stecher G, Tamura K (2016) MEGA7: molecular evolutionary genetics analysis version 7.0 for bigger datasets. Mol Biol Evol 33:1870–1874
Lapidot M, Karniel U, Gelbart D et al (2015) A novel route controlling begomovirus resistance by the messenger RNA surveillance factor Pelota. PLoS Genet 11:e1005538
Li B, Dewey CN (2011) RSEM: accurate transcript quantification from RNA-Seq data with or without a reference genome. BMC Bioinformatics 12:323
Mabvakure B, Martin DP, Kraberger S et al (2016) Ongoing geographical spread of Tomato yellow leaf curl virus. Virology 498:257–264
Malathi VG, Renukadevi P, Chakraborty S et al (2017) Begomoviruses and their satellites occurring in India: distribution, diversity and pathogenesis. In: Mandal B, Rao GP, Baranwal VK, Jain RK (eds) a century of plant virology in India. Springer, Singapore
Mammadov J, Buyyarapu R, Guttikonda SK et al (2018) Wild relatives of maize, rice, cotton, and soybean: treasure troves for tolerance to biotic and abiotic stresses. Front Plant Sci 9:886
Mejía L, Teni RE, Garcia BE, Fulladolsa AC, Mendez L (2010) Preliminary observations on the effectiveness of five introgressions for resistance to begomoviruses in tomatoes. Rept Tomato Genet Coop 60:41–53
Morales-Aguilar JJ, Rodríguez-Negrete EA, Camacho-Beltrán E et al (2019) Identification of Tomato yellow leaf curl virus, Pepper huasteco yellow vein virus and Pepper golden mosaic virus associated with pepper diseases in northern Mexico. Can J Plant Pathol 41:544–550
Mori N, Hasegawa S, Takimoto R, Horiuchi R, Watanabe C, Onizaki D, Shiragane H, Nagano AJ, Kesumawati E, Koeda S (2022) Identification of QTLs conferring resistance to begomovirus isolate of PepYLCIV in Capsicum chinense. Euphytica 218:20. https://doi.org/10.1007/s10681-022-02970-9
Prasanna HC, Sinha DP, Rai GK et al (2015) Pyramiding Ty-2 and Ty-3 genes for resistance to monopartite and bipartite tomato leaf curl viruses of India. Plant Pathol 64:256–264
Qin C, Yu CS, Shen YO et al (2014) Whole-genome sequencing of cultivated and wild peppers provides insights into Capsicum domestication and specialization. Proc Natl Acad Sci USA 111:5135–5140
Qin L, Mo N, Muhammad T, Liang Y (2018) Genome-wide analysis of DCL, AGO, and RDR gene families in pepper (Capsicum annuum L.). Int J Mol Sci 19:1038
Rai VP, Kumar R, Singh SP, Kumar S, Kumar S, Singh M, Rai M (2014) Monogenic recessive resistance to Pepper leaf curl virus in an interspecific cross of Capsicum. Sci Hort 172:34–38
Rentería-Canett I, Xoconostle-Cázares B, Ruiz-Medrano R et al (2011) Geminivirus mixed infection on pepper plants: synergistic interaction between PHYVV and PepGMV. Virol J 8:104
Retes-Manjarrez J, Hernández-Verdugo S, López-Orona C, Medina-López R, Garzón-Tiznado J, Retes-Cázarez J (2019) Inheritance of resistance to Pepper huasteco yellow vein virus in Capsicum annuum L. HortScience 54:783–786
Siddique MI, Lee JH, Ahn JH et al (2022) Genotyping-by-sequencing-based QTL mapping reveals novel loci for Pepper yellow leaf curl virus (PepYLCV) resistance in Capsicum annuum. PLoS One 17:e0264026
Singh AK, Kushwaha N, Chakraborty S (2016) Synergistic interaction among begomoviruses leads to suppression of host defense-related gene expression and breakdown of resistance in chilli. Appl Microbiol Biotechnol 100:4035–4049
Srivastava A, Mangal M, Saritha RK, Jat SL, Gosavy GU, Kalia P (2015) Natural epiphytotic screening of chilli germplasm lines against leaf curl virus complex. Int J Trop Agric 33:3581–3586
Srivastava A, Mangal M, Saritha RK, Kalia P (2017) Screening of chilli pepper (Capscium spp.) lines for resistance to the begomovirus causing chilli leaf curl disease in India. Crop Prot 100:177–185
Sudiono YN, Hidayat SH, Hidayat P (2005) The distribution and molecular detection of geminivirus pathogen of chili yellowing disease in Sumatera Island. J HPT Tropika 5:113–121
Thakur H, Jindal SK, Sharma A, Dhaliwal MS (2019) A monogenic dominant resistance for leaf curl virus disease in chilli pepper (Capsicum annuum L.). Crop Prot 116:115–120
Thakur H, Jindal SK, Sharma A, Dhaliwal MS (2020) Molecular mapping of dominant gene responsible for leaf curl virus resistance in chilli pepper (Capsicum annuum L.). Biotech 10:182
Torre C, Donaire L, Gómez-Aix C et al (2018) Characterization of begomoviruses sampled during severe epidemics in tomato cultivars carrying the Ty-1 gene. Int J Mol Sci 19:2614
Vanderschuren H, Moreno I, Anjanappa RB, Zainuddin IM, Gruissem W (2012) Exploiting the combination of natural and genetically engineered resistance to cassava mosaic and cassava brown streak viruses impacting cassava production in Africa. PLoS One 7:e45277
Verlaan MG, Szinay D, Hutton SF et al (2011) Chromosomal rearrangements between tomato and Solanum chilense hamper mapping and breeding of the TYLCV resistance gene Ty-1. Plant J 68:1093–1103
Verlaan MG, Hutton SF, Ibrahem RM et al (2013) The Tomato yellow leaf curl virus resistance genes Ty-1 and Ty-3 are allelic and code for DFDGD-class RNA-dependent RNA polymerases. PLoS Genet 9:e1003399
Wassenegger M, Krczal G (2006) Nomenclature and functions of RNA-directed RNA polymerases. Trends Plant Sci 11:142–151
Willmann MR, Endres MW, Cook RT, Gregory BD (2011) The functions of RNA-dependent RNA polymerases in Arabidopsis. Arabidopsis Book 9:e0146
Yamaguchi H, Ohnishi J, Saito A et al (2018) An NB-LRR gene, TYNBS1, is responsible for resistance mediated by the Ty-2 Begomovirus resistance locus of tomato. Theor Appl Genet 131:1345–1362
Yan Z, Wolters AA, Navas-Castillo J, Bai Y (2021) The global dimension of tomato yellow leaf curl disease: current status and breeding perspectives. Microorganisms 9:740
Yang X, Caro M, Hutton SF et al (2014) Fine mapping of the tomato yellow leaf curl virus resistance gene Ty-2 on chromosome 11 of tomato. Mol Breed 34:749–760
Zamir D, Eksteinmichelson I, Zakay Y et al (1994) Mapping and introgression of a tomato yellow leaf curl virus tolerance Gene, Ty-1. Theor Appl Genet 88:141–146
Zhang DX, Spiering MJ, Nuss DL (2014) Characterizing the roles of Cryphonectria parasitica RNA-dependent RNA polymerase-like genes in antiviral defense, viral recombination and transposon transcript accumulation. PLoS One 9:e108653
Acknowledgements
We thank Satoko Kondo (Ryukoku University, Japan) for supporting RAD-seq library preparation. We thank Shinya Kanzaki (Kindai University, Japan), Elly Kesumawati (Syiah Kuala University, Indonesia), Ryohei Arimoto (Takii seeds, Japan), and Akihito Kano (Takii seeds, Japan) for useful discussion. The authors would like to thank Enago (www.enago.jp) for the English language review.
Funding
This study was supported by the Japan Society for the Promotion of Science (JSPS) KAKENHI Grant Number 19H02950 and 21KK0109 to S. Koeda.
Author information
Authors and Affiliations
Contributions
SK designed the experiments; performed genetic mapping; analyzed the data; interpreted the results and wrote the manuscript. NM performed virus inoculation, resistance evaluation, genetic mapping, gene expression analysis, and VIGS. RH and CW performed virus inoculation, resistance evaluation. HS prepared the material. AJN, performed RAD-seq. All authors read and approved the final manuscript.
Corresponding author
Ethics declarations
Conflict of interest
The authors declare no conflict/competing of interest.
Additional information
Communicated by Richard G.F. Visser.
Publisher's Note
Springer Nature remains neutral with regard to jurisdictional claims in published maps and institutional affiliations.
Supplementary Information
Below is the link to the electronic supplementary material.
Rights and permissions
About this article
Cite this article
Koeda, S., Mori, N., Horiuchi, R. et al. PepYLCIV and PepYLCAV resistance gene Pepy-2 encodes DFDGD-Class RNA-dependent RNA polymerase in Capsicum. Theor Appl Genet 135, 2437–2452 (2022). https://doi.org/10.1007/s00122-022-04125-9
Received:
Accepted:
Published:
Issue Date:
DOI: https://doi.org/10.1007/s00122-022-04125-9