Abstract
With Yokohama City as the study area, this chapter uses input–output analysis to study impacts of the 2011 Tohoku earthquake and tsunami disaster on urban resource flows and inter-sectoral resource use efficiency. Quantifying resource flows among urban sectors, we assess resource use intensity for production. Input–output analysis of 2005 and 2011 data is used to consider self-sufficiency rates, distinguish resource sources geographically, and clarify impacts of production and consumption on local natural resource systems. Supply chain analysis is used to assess embodied energy use in the urban production layer to explore indirect impacts of sectoral resource consumption. The study found that in the production sector, the 2011 disaster had huge impacts on Yokohama’s energy and water systems, with a significant decrease in resource use efficiency in the energy sector and a substantial increase in energy consumption in water and energy sectors. Supply path analysis showed strong production linkages between energy and water sectors, as well as energy and petrochemical basic product sectors. Demand in the water sector leads to more energy consumption in the energy sector, and further increases consumption of petrochemical basic products. Conversely, consumption in the household sector tends to be economical, resulting in a decline in total water and energy consumption.
Access provided by Autonomous University of Puebla. Download chapter PDF
Similar content being viewed by others
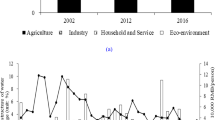
Keywords
- Food–energy–water nexus
- Great East Japan Earthquake
- Input–output analysis
- Resource use efficiency
- Yokohama
1 Introduction
The interdependencies inherent to food, water, and energy infrastructure systems make them highly susceptible to disasters, exposing infrastructure to crucial risk of failure (Dargin et al. 2020; Hameed et al. 2019), which in turn can lead to instability of physical provisioning systems. On March 11, 2011, a massive earthquake in the Pacific Ocean offshore of northeastern Japan damaged food, water, energy infrastructure systems and had a profound impact on society.
In terms of energy, infrastructure was severely damaged in the earthquake, nuclear and thermal power plant stoppages caused a significant drop in power supply capacity, resulting in power outages for nine million households in areas served by Tohoku Electric Power Company and Tokyo Electric Power Company (TEPCO). It took 3 months to fully restore power. Meanwhile, 16 companies in eight prefectures were forced to stop the supply of natural gas, and other parts of supply networks, such as oil refineries and oil supply stations were also damaged, resulting in a decline in energy supply capacity. This earthquake had a profound long-term impact on Japan’s energy system.
As shown in Fig. 1, Japan’s first commercial nuclear power plant began operating in 1966, and by 2010, national nuclear power generation capacity reached 288.2 billion kWh per year. However, due to the combined impacts of the 2011 Tohoku earthquake/tsunami and the subsequent accident at the TEPCO Fukushima Daiichi Nuclear Power Plant, the number of nuclear power plants out of service gradually increased, with the result that, and nuclear power generation plummeted to zero in 2011. As a result of the shutdown of nuclear power plants, imports of oil and natural gas expanded, Japan’s energy self-sufficiency ratio fell from around 20% during the 2000s to around 7% right after 2011 (IEA 2021). Coal generated electricity in 2014 was flat from 2013 at 282.4 billion kWh, about 16 times higher than in 1973 (BEIS 2020). Compared with nuclear power generation, thermal power generation produces more carbon emissions. Japan’s total carbon emissions in 2011 increased by 4.4% compared with the previous year, while the energy conversion sector increased by 7.7% (Ministry of the Environment 2021).
In terms of water, sudden disasters have affected water supplies in disaster-stricken areas and increased pressure on water supply in the Kanto region. During Tohoku earthquake, at least 2.3 million households experienced a water supply cut off. Meanwhile, having faced a spate of extreme weather and weather-related disasters, Japan faces concerns about safety of the food supply. Besides impacts on agricultural production in disaster-affected areas, in the case of Fukushima, radioactive materials in the atmosphere adhered to vegetables through rainfall, and food production in the area was also affected by radioactive materials.
In general, damage to infrastructure from earthquakes threatens the security of resource supplies, especially the food, energy, and water that sustain urban social and economic systems, which inevitably affects production activities of relevant sectors. On the other hand, because of the complex links between FEW infrastructure systems and other sectors in a city, attention should be paid to interactions between FEW sectors (Arthur et al. 2019; Liu et al. 2018) and track resource consumption in each sector. Several studies have analyzed the impact of earthquakes, including disruptions of supply chains, damage to infrastructure, impacts on regional economic development, etc.(Ghobarah et al. 2006; Koshimura et al. 2014; Rose et al. 1997; Son et al. 2020). However, there have been few studies on the impact of earthquakes on resource flows in urban sectors, and most of the analyses on resource flows in urban sectors only stop at quantitative assessments without further evaluating changes in resource flows and their impacts.
Therefore, in order to understand the impacts of the earthquake on resource flows, this chapter aims to assess the change of energy and water flows between urban sectors, as well as resource use efficiency, through input–output analysis. Also, resource flows between sectors closely related to the urban energy and water sectors are further explored through supply chain analysis. The results may provide hints on how to achieve urban sustainable development by improving sectoral resource consumption and resource use efficiency.
2 Method to Assess Disaster Impacts by Input–Output Analysis and Supply Chain Analysis
Food, energy, and water are basic resources that residents rely on for survival. Also, the network characteristics of food, water, and energy make it easier for changes in one sector to affect other sectors, and this can include spreading risks in the event of a disaster. Research shows that the power industry is a key sector that spreads risks to other sectors (Haraguchi and Kim 2016). In a disaster, long-term damage to the infrastructure system may lead to changes in urban physical provisioning systems due to the inherently complex links between components of urban infrastructure (Dargin et al. 2020) and changes in resource supplies will impose the impacts on the amounts of resources used for production. At the same time, in the context of rapid economic development and expanding resource demand, improving resource use efficiency and ensuring the security of resource supply have become important tasks for the development of sustainable urban systems (Feng et al. 2019). Therefore, it is particularly important for industrial sectors in cities to improve their resource consumption patterns and use efficiency.
Existing research has applied various methods to analyze the FEW nexus from different perspectives. While analyzing the flow of a single element, nexus analysis pays more attention to the flow and trade-off relationships between elements (Hussien et al. 2017). Material flow analysis and input–output analysis based on the perspective of production and consumption are used to track and quantify the flows of resources in urban systems. Different from other methods, combined with the inventory analysis (Wang et al. 2019), input–output analysis can track the resource flow relationships between various sectors in a city (Albrecht et al. 2018; Feng et al. 2019; Li et al. 2019), so as to clearly point out the impacts of each sector’s production and consumption on the FEW Nexus. The application of supply chain analysis can also clarify material flows between sectors involved in processes from production to consumption, so as to determine the key sectors that affect resource flows and focus on achieving energy conservation and emission reductions in key sectors (Owen et al. 2018).
Input–output analysis is an economic quantitative analysis method that analyzes “inputs” and “outputs” together. “Inputs” refer to the consumption of various production factors in production processes, such as the consumption of material products, the use of labor, and the acceptance of different types of productive services. “Outputs” refer to the distribution and use of outputs of production, that is, the direction of the physical movement of material products or the objects receiving the service (Leontief 1951). Input–output analysis can be carried out by combining input–output tables and input–output mathematical models.
Figure 2 depicts an input–output table, which shows the sources of inputs and the destinations of outputs of sectors in an economic system, reflecting in detail the interrelationships among the elements of the economic system. The column direction of the table indicates the consumption of inputs (elements) by sector, that is, the sources of the inputs. The row direction reflects the distribution and use of the product, that is, the direction of the outputs. Intermediate inputs and intermediate output constitute area A, which is the core of the input–output table, reflecting the interconnections between intermediate sectors. Intermediate input refers to the input (purchase) of raw materials, etc. (goods and services) required for the production of goods and services from other sectors, while intermediate demand refers to the production (sale) of goods and services as raw materials, etc. for the production of other goods and services. The downward extension of intermediate inputs expands the input elements of material form to other forms, such as labor and fixed assets, constituting area C. The expansion of the intermediate sector to the right reflects that in addition to the expansion of intermediate products used in production, the demand of the final sector, such as household and business consumption, investment, and export, are separated from the production process of the year, constituting area B. The demands of the final sector refers to the consumption of produced goods and services as the final stage of transactions by households, governments, exports, etc. The entire input–output table is centered on area A, and combines column-wise inputs and row-wise outputs to describe the quantitative dependencies among sectors in the economic system.
Based on the input–output table, an input–output mathematical model can be established. The intermediate input and output sectors in Fig. 2 are divided into n sectors, whereby q12 in area A represents the quantity of products of sector 1 used by sector 2, y1 in area B represents the quantity of products provided by sector 1 for the final sector, and Q1 represents the total output of sector 1. From the horizontal line, area A represents the quantity of products consumed by each intermediate sector in a certain period, and area B represents the quantity of products consumed by the final sector. The sum of these two parts is equal to the total production Q of various products in a certain period of time. Therefore, we can establish the quantitative relation model Eq. (1).
The direct consumption coefficient εij is introduced, defined as the quantity of products provided by sector i to be consumed per unit of product production by sector j, which reflects the production technology structure and material use efficiency of the sector, as shown in Eq. (2).
Combining Eqs. (1–3) can be obtained, where I is the unit matrix, (I-ε) reflects the relationship between the input and output of the unit physical product, each column in the matrix shows what sector j produces per unit of product and needs to invest in other product quantities.
Here we introduce the resource consumption coefficient θi, defined as the quantity of a resource that needs to be used per unit of output, as shown in Eq. (4), where Vi represents the energy and water use of sector i.
By combining Eqs. (3) and (4), we can track the flow of energy and water resources across urban sectors, as shown in Eq. (5), where \( {P}_i^f \) is the vector of energy or water consumption triggered by the final sector.
In an open economic environment, the import of products and services also meets a part of the consumer demand within the city, which can be regarded as a leakage of the demand within the city. The resources consumed to meet this part of the demand come from outside the city, so by considering the self-sufficiency rate (1-M) of the sector, the consumption of local resources by local demand can be clarified, as seen in Eq. (6).
In Eq. (6), θ(I − (I − M)ε)−1 represents the resource consumption of sector i resulting from the production of one unit of final product by sector j. Therefore, the change of resource use efficiency of each sector can be observed through the change of θ(I − (I − M)ε)−1 over time, which implies the resource consumption required for the unit output of the sector.
Based on the basic input–output analysis, (I − ε)−1. is denoted as L, which can be expanded by Taylor’s series, as in Eq. (7).
Combining Eq. (6) with Eq. (7) gives us the following equation:
Equation (8) is the Taylor’s expansion, where θ[(I − M)ε]n F is a calculation of the resource use of each production layer along the supply chain.
3 Study Area
Yokohama City is an ordinance-designated city with a population of 3.7 million in Kanagawa Prefecture. This prefecture has great industrial potential since it has been designated as a national strategic special zone to “enhance the international competitiveness of industry” and “promote the formation of international economic activity.” In the region, the government can promote industrial development through targeted deregulation, enabling Yokohama to promote effective regional revitalization. Also, the city is popular for tourists thanks to its diverse tourism resources and facilities. Since Yokohama Station and Tokyo Station are only 30 min apart by rail, the city plays a role as a bedroom suburb of Tokyo as well.
Yokohama has a land area of 437.56 sq. km, accounting for 0.12% of the country’s total area, although it supports 3% of the total Japanese population. As of 2013, Yokohama’s total population was still increasing, although some districts were declining, and this could inevitably increase pressure on the supply of regional resources. Also, the population is getting older, and the number of households over 65 years of age is increasing, making them more vulnerable to earthquakes (Zhu and Sun 2017). Regarding changes in land use structure, the proportion of residential land, commercial land, and land used for public facilities in Yokohama as a whole has increased, while the proportion of industrial land, agricultural land, and wooded land has decreased due to an increase in the population. In particular, industries that require large-scale land use have been relocated in many cases in recent years, and the number of former sites becoming collective housing and large-scale shops is also increasing, which may affect local public infrastructure (Yokohama in 2015). Changes in Yokohama’s land use hint at changes in the city’s intermediate and the final sector. In the following, we take a detailed look at Yokohama’s sectoral economy and resource consumption, and how they were affected by the 2011 Great East Japan Earthquake.
3.1 Yokohama’s Industrial and Economic Development
Changes in city production value and share of Yokohama are given in Table 1. In 2015, Yokohama’s production value accounted for 40.6% of the prefecture’s share and 2.6% of the national share. With the negative growth of Kanagawa and the national production value in 2011, Yokohama’s production value increased by 2.7% over 2005. In 2015, Yokohama’s production value increased by 14.6% compared to 2011, which was much higher than the growth rate of Kanagawa and national production value.
In terms of sectoral development in Yokohama, as shown in Fig. 3, the sector that continues to grow is services and information communications, with production value increasing from 4.5 trillion yen in 1990 to 9.9 trillion yen in 2015, an increase of 2.2 times. The manufacturing sector was the city’s second-largest in production value, after the service sector. The city’s production value for construction has been declining, and the commercial sector has been declining since 2000, but showed a recovery trend in 2015. Changes in the resource consumption of a sector and the resource flows between sectors are closely related to the output value of the urban sector. The ratio of the resource input and output value of a sector reflects the resource use efficiency of a sector. Due to correlations between sectors, a decline (or increase) in the resource use efficiency of give sector may indirectly lead to an increase (or decrease) in resources used by downstream sectors.
3.2 Energy, Water, and Food Supply in Yokohama
Figure 4 shows the changes in the self-sufficiency rate of the energy, water, and food sectors in Yokohama, where the self-sufficiency rate of the electricity, gas, and heat supply sectors remained at 100% in 2011. However, the petroleum and coal products sector has a low self-sufficiency rate of about 27%. The self-sufficiency rate of the water and waste treatment sector increased from 93% in 2005 to 99.99% in 2011, basically achieving self-sufficiency. The food sector has a low rate of self-sufficiency, at 5% in 2011, an increase of 3% from 2005. The food and beverage industry’s self-sufficiency rate was 16% in 2011, down from 3% in 2005.
As can be seen from Yokohama’s input–output table, the production of the power, gas, and heat supply sector is heavily dependent on the mining, petroleum, and coal products sectors. The low self-sufficiency rate in the mining sector and the petroleum and coal products sectors threatens the supply security of electricity, heat, and other energy resources to cities during disasters.
3.3 Impacts of the 2011 Great East Japan Earthquake on Yokohama
The nuclear power plant accident caused by the earthquake in 2011 resulted in a significant reduction in power supply capacity. The proportion of thermal power generation increased, causing various problems such as increased greenhouse gases. Restrictions on electricity supply capacity put significant downward pressure on the supply side. Rolling power outages were used to limit the use of electricity in some areas, which had a great impact on the lives of citizens.
Also, the government asked large and small consumers and households to reduce their peak electricity consumption by approximately 15%. According to estimates, a 1% reduction in electricity supply would have a negative impact of approximately 0.9% on production across the sector. However, the impact on the manufacturing sector would be more pronounced than on the non-manufacturing sector. Within the manufacturing sector, Japan’s major export industries, such as electronics, machinery, and equipment, transport machinery and steel, would be more affected (Koike 2012). On the other hand, the Tohoku region is an important part of not only domestic but also international supply chains. Ibaraki and Chiba prefectures, neighboring the Tohoku region, are the source of a large supply of products, including communication machinery and related equipment, computers and related equipment, general machinery, and industrial electrical equipment. Impacts on those industries would have other impacts through the supply chain, including Yokohama.
Since the earthquake, Kanagawa Prefecture, where Yokohama is located, has been committed to transition from centralized to distributed power sources (Kanagawa Prefecture Industrial and Labor Bureau 2020). To this end, Yokohama has declared its intention to realize community building with uninterrupted basic services in the event of disasters through energy decentralization and independence from the perspective of global warming countermeasures (Yokohama City 2013).
As Japan’s second-largest city by population, Yokohama has a huge demand for resources. Located in the Tokyo metropolitan area, it is home to regional electricity and gas companies such as TEPCO and Tokyo Gas, as well as other large energy-related companies that serve the Kanto region.
Table 2 shows the close economic ties between Tokyo and Yokoham. Table 2 shows how much the demand in the region at the top of the table induces production in the region at the side of the table. The shaded area indicates the production inducement effect by the demand in the own region. Vertically, the demand in the Yokohama has induced production of 10925.1 billion yen in Yokohama, 2752.8 billion yen in Tokyo, and 9575.5 billion yen in other regions including the whole country. Besides, the demand in Tokyo has induced production of 1152 billion yen in Yokohama.
Using Yokohama as a study area, it is important to identify the impact of a major earthquake on sectoral resource consumption, including changes in sectoral resource use efficiency and inter-sectoral resource flows, in order to achieve resource conservation and reduce carbon emissions through improved energy supply and resource use efficiency in the city, and to improve the resilience of the city’s sectors.
4 Data Processing and Results
4.1 Data Processing
The situation in the study area, Yokohama, was introduced in the previous section. Data required to analyze the resource consumption by Yokohama’s various sectors through input–output tables includes the input–output table, energy, and water consumption data of intermediate sectors, and self-sufficiency rates of intermediate sectors. Data for 2011 and 2005 were selected in order to reflect the impacts of the 2011 Great East Japan Earthquake on Yokohama.
Input–output data for Yokohama comes from the input–output table issued by the Yokohama municipal government. Based on the input–output table, the self- sufficiency rate (I-M) of each intermediate sector can be calculated by Eq. (9).
Data for energy consumption of the intermediate sector are obtained from Agency for Natural Resources and Energy. Statistics on energy consumption are only accurate to the prefectural level, so the energy consumption of each sector in Yokohama is estimated in this study using energy consumption per million yen output by intermediate sector in Kanagawa and output by intermediate sector in Yokohama.
Data for water consumption in the intermediate sector are obtained from Yokohama City’s Waterworks Bureau.
4.2 Results
4.2.1 Water and Energy Use Triggered by Intermediate Sector Production
Figure 5 shows changes in water and energy use per unit of yen arising from output in intermediate sectors in 2011 compared to 2005. Changes in water intensity arising from production of the intermediate sectors are greater for energy intensity. The water (Wa) and other manufacturing (Om) sectors experienced significant increases in production-induced water intensity, followed by the transportation (Tr) and mining (Mi) sectors, while there was a decline in water intensity caused by production in the service (Se) and food manufacturing (Fm) sectors. In terms of energy consumption, the water (Wa) and energy (El) sectors experienced significant increases in production-induced energy intensity, followed by the transportation (Tr) and mining (Mi) sectors, while there was a decline in water intensity caused by production in the service (Se) and food manufacturing (Fm) sectors. Production in the business and service sectors resulted in a decrease in the resource intensity of their own production, while production in the other manufacturing (Om), water (Wa), electricity, gas and heating (El) resulted in an increase in the resource intensity of their own production.
Changes in intermediate sector production-induced water intensity (cu m per thousand yen) (left) and energy intensity (GJ per million yen) (right). Agriculture (Ag), Commerce (Com), Construction (Co), Electricity, gas and heating (El), Finance (Fi), Food manufacturing (Fm), Mining (Mi), Other manufacturing (Om), Real estate (Es), Service (Se), Telecommunication (Te), Transportation (Tr), Unknown classification (Un), Water and waste management (Wa), intermediate supply (Inter_S), intermediate demand (Inter_D)
Overall, in terms of water consumption in each sector, compared with 2005, the production activities of various urban sectors in 2011 led to the consumption of more water in the “other manufacturing” sector, followed by the water, telecommunication, and commercial sectors. As supplier of commodities, water consumption per thousand yen of output in the “other manufacturing” sector increased significantly, followed by the mining and transportation sectors. Conversely, water consumption associated with the service and food manufacturing sectors dropped significantly. In terms of energy consumption, production activities in various sectors of the city led to more energy consumption in the water and energy sectors. Also, the water and energy sectors significantly increased in water consumption per thousand yen of output, while energy consumption related to the service and agricultural sectors dropped significantly.
4.2.2 Energy and Water Use Triggered by the Final Sector
Commodity demand for the final sector, which is dominated by the household and export sectors, triggers the production and service activities of the intermediate sectors, which in turn leads to the consumption of resources. Through input–output analysis, it is possible to track the water and energy consumption of the intermediate sector triggered by the demand of the final sector.
The amount of energy consumption triggered by the final sectoral demand is given in Table 3. In 2011, the per capita daily energy consumption in the household sector was 4.02 kWh, a 15% decrease from 2005. From the table we can see that the energy consumption of the commercial sector triggered by the demand of the household sector was the highest, followed by the energy sector and the service sector. Compared with 2005, the energy consumption of the manufacturing sector caused by the demand of the household sector dropped significantly, while the energy demand of the energy supply and the water sector increased significantly. In terms of the energy consumption triggered by the export sector, in 2011, the annual energy consumption per million yen in the export sector was 27.38, a decrease from 2005. The energy consumption of the manufacturing sector triggered by the demand of the export sector was the highest, followed by the service sector. Compared with 2005, the energy consumption of the service sector triggered by the export sector’s demand dropped significantly, while the energy demand of the water sector increased significantly.
The amount of water consumption triggered by the final sectoral demand is given in Table 4. In 2011, the per capita water consumption in the household sector was 44.17 liters per day, a 14% decrease from 2005. The manufacturing sector consumed the most water resources triggered by the demand from the household sector, followed by the service sector and the food and beverage sector. Compared with 2005, the consumption of water resources in the service industry triggered by the household sector’s demand dropped significantly, while the demand for water resources in the transportation sector increased significantly. In terms of water consumption caused by the export sector, in 2011, water consumption per million yen was 1,12.80 L, down 15% from 2005. The manufacturing sector consumed the most water resources triggered by export demand, followed by the commercial and service sectors. Compared with 2005, the consumption of water resources in the manufacturing industry triggered by the export sector dropped significantly, while the demand for water resources in the water, commercial, and service sectors increased.
4.2.3 Energy Use Embodied in Production Layers
Table 5 shows the amounts and changes of energy use triggered by water and energy sectors in different production layers in 2011. Production in both the Wa and El sectors triggered energy consumption in the El sector, Pe sector. Compared with 2005, final sectoral consumption triggered more energy consumption, and production demand in the energy and water sectors triggered a substantial increase in energy consumption in the energy, petroleum, and coal sectors. The final sector’s demand for products from the water sector caused a large amount of energy consumption in the water sector and commercial service sectors, and compared with 2005, this part of the energy consumption increased significantly; the production of the energy sector triggered a large amount of energy use in the construction sector and the transportation sector.
5 Discussion
In this section, we first examine Yokohama’s role in securing energy for the Tokyo metropolitan area, then analyze the results of input–output analysis based on the carbon emissions of each sector in Yokohama, and how the 2011 earthquake effected resource use efficiency in each sector. Finally, we discuss the advantages and limitations of input–output analysis.
5.1 The Role of Yokohama City in Securing Energy for the Tokyo Metropolitan Area
As shown in Fig. 6, the wide-area power system in the Tokyo metropolitan area consists of multiple 500 kV outer ring lines and transmission lines connected to the outer ring lines. A large amount of power is transmitted within the metropolitan area. There are several high-capacity power strips in the metropolitan area, the largest of which is located the Tokyo Bay area. In that area, TEPCO has capacity of 29.1 million kilowatts of thermal power stations, accounting for 70% of the total thermal power generation, while the thermal power stations located in Yokohama have capacity of 4.62 million kilowatts, accounting for 16% of the total. Yokohama also has a diverse range of power generation options inland, providing security of electricity supply to the Tokyo metropolitan area. Table 6 shows the energy supply (electricity, gas, heat) in each region of the Kanto area. The table shows that the self-sufficiency rate of Yokohama’s energy sector is about 100%, and the outflow of the energy sector accounts for 65.4% of the entire Kanagawa, which fully demonstrates Yokohama’s important role in ensuring the regional energy supply.
5.2 Impact of Earthquakes on CO2 Emissions and Resource Use Efficiency
Energy consumption is the main source of carbon emissions. According to the Japanese Ministry of the Environment, Japan’s carbon dioxide emissions in 2020 were 1044 million tons, of which energy-related carbon dioxide emissions were 967 million tons (84.2%), and non-energy-related carbon dioxide emissions were 78.9 million tons (6.7%). After the 2011 earthquake, Japan’s energy structure changed significantly, with the replacement of nuclear energy by natural gas, oil, and coal, which led to a significant increase in total carbon emissions and energy consumption of the energy conversion sector. The carbon emission factor of electricity also increased, thereby increasing the carbon emissions of other sectors from the consumption of electricity.
Analysis of survey of carbon emissions and energy consumption of each sector in Yokohama showed that after the 2011 earthquake, carbon emissions in all sectors increased significantly (Yokohama, 2022). Although the energy consumption of all types of sectors (except the energy conversion sector) decreased due to planned blackouts conducted by the local government, total carbon emissions increased significantly, due to a significant increase in the carbon emissions coefficient of electricity consumption in 2011 in the business, household, and industrial sectors. Figure 7 shows the trend of changes in carbon emissions per unit of electricity consumption in each sector in Yokohama before and after the earthquake. As for the energy conversion sector, after the earthquake, the carbon emissions and energy consumption of this sector increased significantly, resulting in a slight change in carbon dioxide emissions per unit of energy consumption.
Through input–output analysis, we could identify the substantial increase in energy use in the energy and water sectors that occurred after the 2011 earthquake, which may indirectly increase resource consumption in other sectors. For example, from the results of changes in resource consumption triggered by the final sector, it could be seen that a decline in resource use efficiency in the energy sector increased energy consumption caused by electricity demand in the household sector. Also, by analyzing energy use embodied in production layers, we could find that the increase in energy demand in the energy sector directly led to more energy consumption in the coal and petroleum sector, leading to more carbon emissions of the energy conversion sector. On the other hand, due to damage to the power and transportation infrastructure, production of the industrial sector, including the manufacturing sector, was also greatly affected. The efficiency of water and energy use in the industrial sector declined, leading to more carbon emissions. In terms of water supply, the earthquake caused damage to the water infrastructure in the Kanto region, including both the displacement of and damage to water pipes, which also affected energy and water use efficiency of the water sector.
It is clear that the impact of changes in resource use efficiency of sectors will expand through inter-sectoral correlations and eventually affect the total resource consumption of each sector. Therefore, the resource use efficiency of the resource supply sector should be improved first, which will indirectly reduce resource consumption in other sectors. Secondly, resource consumption sectors should actively make use of natural resource systems such as solar power generation and rainwater harvesting, which can help reduce pressure on urban physical provisioning systems and enhance the ability of various sectors to deal with risks.
5.3 Advantages and Limitations of Input–Output Analysis
Through input–output analysis, the flow of material resources between sectors can be estimated based on economic linkages between urban sectors, which cannot be achieved by other methods. By linking environmental indicators with input–output tables, we can evaluate impacts on the environment in the context of a city’s economic development. Meanwhile, since input–output tables have clear geographical boundaries, the connections between regions can also be clarified, so that resource transfers between regions and related impacts associated with economic activities can be further analyzed. However, as mentioned, the compilation of input–output tables is very cumbersome. Thus, not every region has an input–output table, which limits the application of regional input–output analysis. Data required for input–output analysis is difficult to obtain, and results of analysis will deviate from the actual situation depending on the accuracy and scope of data. Therefore, for input–output analysis it is necessary to clarify the specific economic activities that are to be included from the inputs and outputs of each sector into the input–output table, as well as the resource consumption caused thereby, so as to more accurately evaluate resource flows between sectors and changes in resource use efficiency.
6 Conclusion
This chapter assessed resource consumption and flows between sectors in order to analyze the impacts of the 2011 Tohoku earthquake and tsunami disaster on urban resource supply and resource use efficiency, with Yokohama as the study area. Our research found that the energy and water sectors responsible for resource generation and distribution were significantly affected by the disaster, which indirectly contributed to increased energy and water consumption triggered by some sectors due to cross-sectoral interdependencies. Also, the energy and water sectors are highly correlated, so changes on one side directly and indirectly affect the resource consumption and use efficiency on the other. Therefore, attention should be paid to how to achieve the stability of urban physical provisioning by promoting the capacity of energy and water infrastructure systems to respond to risks. Meanwhile, for sectors where resource use has declined, the resource use pattern of sectors should be improved through technological advancement or greater awareness of conservation.
References
Agency for Natural Resources and Energy; Annual Energy Report of Japan in Fiscal Year 2023, p77; https://www.enecho.meti.go.jp/about/whitepaper/2023/pdf/
Albrecht TR, Crootof A, Scott CA (2018) The water-energy-food nexus: a systematic review of methods for nexus assessment. Environ Res Lett 13(4):043002. https://doi.org/10.1088/1748-9326/aaa9c6
Arthur M, Liu G, Hao Y, Zhang L, Liang S, Asamoah EF, Lombardi GV (2019) Urban food-energy-water nexus indicators: a review. Resour Conserv Recycl 151:104481. https://doi.org/10.1016/j.resconrec.2019.104481
BEIS (2020) Energy White Paper. Energy Department 44:903–905; http://www.ret.gov.au/energy/facts/white_paper/Pages/energy_white_paper.aspx
City of Yokohama; Yokohama Economic and Trade Bureau, 2015 Yokohama City Input-Output Table; https://www.city.yokohama.lg.jp/business/kigyoshien/tokei-chosa/rennkannhyou.html
City of Yokohama, Greenhouse gas emissions in the city in FY2020; https://www.city.yokohama.lg.jp/cityinfo/koho-kocho/press/ondan/2022/0422haishutsuryou.files/0001_20220420.pdf
Dargin J, Berk A, Mostafavi A (2020) Assessment of household-level food-energy-water nexus vulnerability during disasters. Sustain Cities Soc 62:102366. https://doi.org/10.1016/j.scs.2020.102366
Feng C, Qu S, Jin Y, Tang X, Liang S, Chiu ACF, Xu M (2019) Uncovering urban food-energy-water nexus based on physical input-output analysis: the case of the Detroit Metropolitan Area. Appl Energy 252:113422. https://doi.org/10.1016/j.apenergy.2019.113422
Ghobarah A, Saatcioglu M, Nistor I (2006) The impact of the 26 December 2004 earthquake and tsunami on structures and infrastructure. Eng Struct 28(2):312–326. https://doi.org/10.1016/j.engstruct.2005.09.028
Hameed M, Moradkhani H, Ahmadalipour A, Moftakhari H, Abbaszadeh P, Alipour A (2019) A review of the 21st century challenges in the food-energy-water security in the middle east. Water (Switzerland) 11(4):682. https://doi.org/10.3390/w11040682
Haraguchi M, Kim S (2016) Critical infrastructure interdependence in New York City during Hurricane Sandy. Int J Disaster Resil Built Environ 7(2):133–143. https://doi.org/10.1108/IJDRBE-03-2015-0015
Hussien WA, Memon FA, Savic DA (2017) An integrated model to evaluate water-energy-food nexus at a household scale. Environ Model Softw 93:366–380. https://doi.org/10.1016/j.envsoft.2017.03.034
Ijo T, Oshima K (2019) Creation and analysis of an inter-industry relationship table among the seven regions of Japan, with Minato Mirai 21 and Yokohama city center as the segments. Yokohama International Social Science Research 23(3):283–295
International Energy Agency (IEA) (2021) Japan 2021—Energy Policy Review. International Energy Agency; https://www.iea.org/reports/japan-2021
Institute for Global Environmental Strategies; Yokohama in 2030.(2021). https://www.iges.or.jp/en/publication_documents/pub/policyreport/en/11892/IGES_Yokohama+Scenario_Web.pdf
Kanagawa Prefectural Industrial Labor Bureau (2020); 32 March 2018
Koike J, Ukon T (2012) Economic Impact Analysis of Electricity Supply Shortages. Collected Papers of the Civil Engineering Society d3 (Civil Engineering Design) 68(5):I_111-I_119. https://doi.org/10.2208/jscejipm.68.I_111
Koshimura S, Hayashi S, Gokon H (2014) The impact of the 2011 Tohoku earthquake tsunami disaster and implications to the reconstruction. Soils Found 54(4):560–572. https://doi.org/10.1016/j.sandf.2014.06.002
Leontief WW (1951) Input-Output Economics. 185(4):15–21
Li X, Yang L, Zheng H, Shan Y, Zhang Z, Song M, Cai B, Guan D (2019) City-level water-energy nexus in Beijing-Tianjin-Hebei region. Appl Energy 235:827–834. https://doi.org/10.1016/J.APENERGY.2018.10.097
Liu J, Hull V, Godfray HCJ, Tilman D, Gleick P, Hoff H, Pahl-Wostl C, Xu Z, Chung MG, Sun J, Li S (2018) Nexus approaches to global sustainable development. Nature Sustainability 1(9):466–476. https://doi.org/10.1038/s41893-018-0135-8
Ministry of the Environment of Japan; Greenhouse Gas Inventory Office of Japan of NIES (2021) National Greenhouse Gas Inventory Report of Japan in Fiscal Year 2021, p 794; https://warp.da.ndl.go.jp/info:ndljp/pid/11434599/www.cger.nies.go.jp/publications/report/i144/i144.pdf
Owen A, Scott K, Barrett J (2018) Identifying critical supply chains and final products: an input-output approach to exploring the energy-water-food nexus. Appl Energy 210:632–642. https://doi.org/10.1016/j.apenergy.2017.09.069
Rose A, Benavides J, Chang SE, Szczesniak P, Lim D (1997) The regional economic impact of an earthquake: direct and indirect effects of electricity lifeline disruptions. J Reg Sci 37(3):437–458. https://doi.org/10.1111/0022-4146.00063
Son BG, Chae S, Kocabasoglu-Hillmer C (2020) Catastrophic supply chain disruptions and supply network changes: a study of the 2011 Japanese earthquake. Int J Oper Prod Manag 41(6):781–804. https://doi.org/10.1108/IJOPM-09-2020-0614
Wang S, Fath B, Chen B (2019) Energy–water nexus under energy mix scenarios using input–output and ecological network analyses. Appl Energy 233:827–839. https://doi.org/10.1016/J.APENERGY.2018.10.056
Yokohama City Basic Policy on Power Saving and Energy Saving Measures (2013)
Zhu X, Sun B (2017) Study on earthquake risk reduction from the perspectives of the elderly. Saf Sci 91:326–334. https://doi.org/10.1016/j.ssci.2016.08.028
Acknowledgments
This article is part of the M-NEX project (m-nex.net), a grant of the Sustainable Urbanization Global Initiative (SUGI) —Food–Water–Energy Nexus by the Belmont Forum and Japan Science and Technology Agency (JST). The authors are grateful for financial support from the Taikichiro Mori Memorial Research Fund of Keio University.
Author information
Authors and Affiliations
Editor information
Editors and Affiliations
Rights and permissions
Copyright information
© 2024 The Author(s), under exclusive license to Springer Nature Singapore Pte Ltd.
About this chapter
Cite this chapter
Hanyu, L., Yan, W. (2024). Impacts of the 2011 Disaster on Food–Energy–Water Material Flows and Resource Use Efficiency in Yokohama City. In: Yan, W., Galloway, W., Shaw, R. (eds) Resilient and Adaptive Tokyo. Springer, Singapore. https://doi.org/10.1007/978-981-99-3834-6_11
Download citation
DOI: https://doi.org/10.1007/978-981-99-3834-6_11
Published:
Publisher Name: Springer, Singapore
Print ISBN: 978-981-99-3833-9
Online ISBN: 978-981-99-3834-6
eBook Packages: Earth and Environmental ScienceEarth and Environmental Science (R0)