Abstract
In this chapter, the effects of soil factors and agricultural practices on the biogeography of rhizobia are discussed. Soil properties, such as pH level, significantly affect the distribution of soybean rhizobia especially in China. Further, land use and crop management have a distinct influence on the distribution of soybean rhizobia. For the rhizobia associated with medicinal legume Astragalus spp., distribution of these nodule isolates is negatively related to the application of fertilisers. Geographic separation for a long time prevents the gene exchange and recombination of rhizobia.
Access provided by Autonomous University of Puebla. Download chapter PDF
Similar content being viewed by others
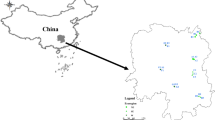
1 Soil Factors Affecting the Distribution of Rhizobia
1.1 Soil Factors Affecting the Distribution of Soybean Rhizobia
The distribution of soybean rhizobia is clearly affected by soil factors, especially the soil pH. Species in the genus Bradyrhizobium are widely distributed around the world but mainly found in acid soils, while these species belonging to Sinorhizobium (syn. Ensifer) are distributed in Asia mainly in alkaline soils (Zhang et al. 2011). No Sinorhizobium symbionts of soybean, but only bradyrhizobia, were found in the USA (Shiro et al. 2013). This is clearly related to the acidic to slightly alkaline pH (5.18–7.68) in the American soils. Both B. japonicum and B. elkanii were isolated from nine field soils in the USA. Isolates related to B. japonicum Bj123, B. japonicum Bj6 and B. elkanii Be46 were found, respectively, mainly in northern, middle and southern regions, significantly related to latitude (r2 = 0.815) (Shiro et al. 2013).
Besides the soil pH, the concentration of available Fe (AFe) was found to determine the distribution of Bradyrhizobium and Sinorhizobium in China (Yang et al. 2018). The higher concentration of AFe (54·1–155 mg kg−1), corresponding to acid soil, favoured the distribution of Bradyrhizobium species. Soils with lower AFe content (8.75–8.94 mg kg−1), corresponding to alkaline pH (7.59–7.60), were occupied by S. fredii, while in soils with intermediate AFe content (61.9 mg kg−1) and neutral soil (pH 6.98), both Sinorhizobium and Bradyrhizobium species were found.
In another study in Hebei Province, north of China by Li et al. (2011), they found that B. japonicum and B. elkanii strains were found only in neutral to slightly alkaline soils, whereas B. yuanmingense, B. liaoningense-related strains and five strains within the genus Sinorhizobium were found predominantly in alkaline-saline soils. The pH and available phosphorus in soils had the greatest influence on the soybean rhizobia distributed in Hebei Province of China. Especially, most isolates (total 91 of 215) within S. fredii and three isolates belonging to the B. liaoningense-related group were adapted to saline-alkali soils with high concentration of available phosphorus and organic material. In addition, strains belonging to Sinorhizobium spp. I and II, S. americanum-related, S. sojae and B. yuanmingense, could adapt to saline-alkali soils with a high concentration of available potassium. Only two isolates belonging to S. fredii, but most bradyrhizobia (27 isolates classified as B. japonicum, and 16 isolates clustered into B. elkanii) preferred soils with relative low pH and low concentration of available potassium and salt.
The soil pH-dependent characteristic distribution of the two genera of Bradyrhizobium and Sinorhizobium associated with soybean was supported by the comparative genomes of these rhizobia (Tian et al. 2012). Several gene clusters known to be involved in osmoprotection and adaptation to alkaline pH were found specifically in the Sinorhizobium core genome (Tian et al. 2012). The genome of Bradyrhizobium contained lipid and secondary metabolism-related genes, consistent with the biogeographic patterns and wider adaptation in acid soils but not in alkaline soils (Tian et al. 2012). The soil pH can be used as a gold marker to select applicable rhizobial species in Bradyrhizobium or Sinorhizobium when considering the inoculation of soybean in different soils of China.
The geographic distributions of the two genera, Bradyrhizobium and Sinorhizobium, were not significantly affected by the different soybean varieties (Yang et al. 2018).
1.2 Soil Factors Affecting the Distribution of Astragalus Rhizobia
The geographic distribution of Astragalus rhizobia has been discussed in detail in Chap. 8 of this book. Here we only focus on the factors in soil affecting the distribution of rhizobia associated with medicinal legumes, Astragalus mongholicus, Astragalus membranaceus and their closer relative Hedysarum polybotrys, based on the work of Yan et al. (2016).
The fields used for growing the above medicinal legumes are of two kinds: with fertiliser applied and without fertiliser. Clearly, the components of these two kinds of soils are different, and therefore they influence the bacterial communities and populations inhabiting the nodules of these medicinal legumes. In our survey of the soil properties of the fields used for the growth of the medicinal legumes, we found the fields at some sites (N7, N4, N6, G5, G4, G2, N5, G3, S2) were supplied by farmers with higher concentration of nitrogen, phosphate and potassium fertilisers (AN, TN, AP, AK), and the distribution of nodule isolates there was negatively related to these fertilisers (Fig. 9.1) (Yan et al. 2016). The species M. temperatum was distributed more widely than other species, suggesting its environmental adaptation and higher competitive abilities than other species. The species M. ciceri was only found in nodules of Site S1, whose soil was very barren, with no fertilisers used there. The M. ciceri identified here should be reclassified as M. cantuariense based on recA sequence similarity (99%) (De Meyer et al. 2015). Site S1 was also occupied by the species M. septentrionale, even more predominant than M. ciceri (M. cantuariense). The higher content of organic carbon (OC) in Sites N2 and N3 favoured the wide distribution of M. temperatum. The species M. tianshanense was distributed mainly at Site N5, where the OC content was relatively lower than at other sampling sites (Fig. 9.1).
Correspondence analysis among the soil factors, sampling sites and distribution of Astragalus-rhizobia-associated genospecies (Yan et al. 2016). Abbreviations for soil factors are as follow: TN total nitrogen, OC organic carbon, AN available nitrogen, AP available phosphorus, AK available potassium, and EC electrical conductivity. Nodule bacteria were isolated from in Ningxia (N1–N7), Gansu (G1–G5) and Shanxi (S1 and S2) provinces, respectively (Yan et al. 2016)
In contrast, R. yanglingense and P. giardinii were also found in nodules of Astragalus species, but they tended to exist in soils with low OC and alkaline pH values. The pH was positively correlated with the distribution of M. temperatum, M. muleiense, R. alamii and Rhizobium sp. I in nodules collected from sampling site N2 but was negatively correlated with the distribution of other genospecies. Electrical conductivity (EC) and available phosphorus (AP) were negatively correlated with the distribution of M. muleiense but positively correlated with the distribution of A. tumefaciens and M. tianshanense (Yan et al. 2016).
It is suggested that, since these medicinal legumes have N2-fixing ability by associating with rhizobia, the usage of fertilisers, especially nitrogen fertiliser, should be restricted or limited to the lowest amount, so that symbiotic nitrogen fixation can be made functional by inoculating effective rhizobial inoculants. In considering the selection of effective rhizobia, species in the genus Mesorhizobium, especially the two species M. temperatum and M. septentrionale, should be considered first. Even though M. temperatum was predominant in nodules where these medicinal legumes were grown in nitrogen-rich fields (Yan et al. 2016), the nitrogen-fixing activity may be inhibited because of the higher nitrogen content in the soils.
2 Effects of Agricultural Practices on Distribution of Rhizobia
2.1 Effect of Land Use and Crop Management on the Distribution of Soybean Rhizobia
The long-term effects of land use and crop management on the distribution of soybean rhizobia was extensively studied by Yan et al. (2014). In their studies, seven different treatments were set up, including grassland, bare land and different rotations of soybean, maize and wheat with different fertilization and tillage (Yan et al. 2014). Because of the acid pH of the soils (5.68–6.30), all the soybean rhizobia in the experimental fields (Hailun) in Heilongjiang Province, northeast China, were Bradyrhizobium but included different species (Yan et al. 2014).
Four species, B. japonicum and three potential novel Bradyrhizobium spp. (I, II and III) were found in the experimental fields (Yan et al. 2014). Bradyrhizobium sp. III was later identified as B. ottawaense (Yan et al. 2017; Yu et al. 2014). Grassland maintained a higher diversity of soybean bradyrhizobia than the other cultivation systems. All the four species could be trapped using soybean from the grassland, whereas from the other treatments only two or three species were found. Of the four species, B. sp. I and III were predominant in the experimental fields, accounting for 90.7% of the total isolates, followed by B. japonicum (7.9%) and B. sp. II (1.4%).
The predominant species in grassland was B. sp. I (42.5%), followed by B. sp. III (35.0%), B. japonicum (12.5%) and B. sp. II (10.0%). The significantly increased organic carbon, available phosphorus contents and the pH level because of long-term natural restoration in grassland may contribute to the high diversity of bradyrhizobia compared to other treatments. In particular, B. sp. II was the only species that was isolated from the grassland but could not be found in other treatments. Though the abundance of B. sp. II was lower, it would be worth studying the reasons for the emergence of this species, its competitiveness with other species and symbiotic nitrogen-fixing efficiency.
In the bare land treatment, where the grasses were manually eliminated periodically, Bradyrhizobium sp. III (77.5%) was the dominant species, followed by B. sp. I (15.0%) and B. japonicum (7.5%). The composition of the soybean rhizobia was similar to that in the cropland treatment (rotation among maize/soybean/wheat and no fertiliser supplied since 1985) and similar to the treatment with rotation of the above crops but with chemical fertiliser since 1990. However the usage of chemical fertiliser in the rotation of the three above crops changed the ratio of the three rhizobial species, with B. sp. I as the dominant species (65.0%), followed by B. japonicum (25.0%) and B. sp. III (10%).
Curiously, only two rhizobial species, B. sp. I and III, were found in all the three monocropped treatments of soybean, maize or wheat. In the monocropped soybean treatment, B. sp. I was the dominant species, while in the monocropped maize/wheat treatment, B. sp. II was the main rhizobial species. Clearly, the legume and cereals changed the long-term ratio of the rhizobial species in soil. The symbiotic nitrogen-fixing efficiency and adaptation of these two species should be evaluated in the future.
The relationships between soil environmental factors and soybean rhizobial genospecies were also analysed. The organic carbon (OC) content and pH had a strong positive correlation with the existence of B. sp. I, B. japonicum and B. sp. II and strong negative correlation with the distribution of B. sp. III. This was due to the increase of grass in the soil in the grassland treatment. The available potassium (AK) and phosphorus (AP) contents had slight effects on the distribution of soybean rhizobia, especially in fields with no chemical fertiliser supply, such as cropland and grassland treatments. The soil bulk density was positively correlated with the distribution of B. sp. III but negatively correlated with B. spp. I, II, B. japonicum and the population size (abundance) of soybean rhizobia in the soil.
In conclusion, the population, communities and abundance of rhizobia were determined not only by the host legume cultivation history but also by fertiliser application and crop management.
2.2 Distribution of Bradyrhizobia Associated with Kummerowia spp. Grown in Urban and Rural Areas
Kummerowia stipulacea (Korean clover) and Kummerowia striata (Japanese clover) are small legumes widely distributed in rural and urban areas (Ji et al. 2018; Lin et al. 2007; Park et al. 1999). Most rhizobia associated with Kummerowia spp. were classified into the slow-growing genus Bradyrhizobium (Lin et al. 2007), though some fast growers were isolated from the nodules of these plants (Liu et al. 2012; Wei et al. 2002; Yao et al. 2012).
Kummerowia-associating bradyrhizobia isolated from rural (130 isolates) and urban (153 isolates) areas, respectively, were compared and analysed to explore the distribution and gene exchange among these strains (Ji et al. 2018).
Based on the analysis of housekeeping genes, all the strains isolated from urban areas were clustered into one large branch, containing three genospecies, while the other strains from rural areas were very diverse and they separated clearly from the urban strains (Ji et al. 2018). Similarly, the analyses of symbiosis genes (nodC and nifH) were consistent with results of housekeeping genes (Ji et al. 2018). The phylogenetic consistency between the housekeeping genes and the symbiosis genes suggests the coevolution of these two kinds of gene. In addition, it also reveals that geographic segregation has occurred between the strains in urban and rural areas.
The rate of nonsynonymous substitutions of the strains from rural areas was higher than in those from urban areas for the housekeeping genes, suggesting gene flow and genetic exchange among these strains. In addition, nonsynonymous substitution rates were higher among strains from southern China than those from northern China. However the opposite was true for the symbiosis genes, where synonymous substitution rates were higher.
In conclusion, the results confirm that the diversity of Kummerowia-associating rhizobia has been drastically reduced in urban environments. Gene exchange and recombination were frequent within the rural or urban areas separately but seldom occurred between the two ecoregions. Furthermore, a single evolutionary lineage formed gradually for the Bradyrhizobium species of cultivated Kummerowia because of urban segregation (Ji et al. 2018).
References
De Meyer SE, Tan HW, Heenan PB, Andrews M, Willems A. Mesorhizobium waimense sp. nov. isolated from Sophora longicarinata root nodules and Mesorhizobium cantuariense sp. nov. isolated from Sophora microphylla root nodules. Int J Syst Evol Microbiol. 2015;65(10):3419–26. https://doi.org/10.1099/ijsem.0.000430.
Ji ZJ, Liu TY, Zhang JX, Yan H, Wang ET, Cui QG, Chen WX, Chen WF. Genetic divergence among Bradyrhizobium strains nodulating wild and cultivated Kummerowia spp. in China. Syst Appl Microbiol. 2018. https://doi.org/10.1016/j.syapm.2018.10.003.
Li QQ, Wang ET, Zhang YZ, Zhang YM, Tian CF, Sui XH, Chen WF, Chen WX. Diversity and biogeography of rhizobia isolated from root nodules of Glycine max grown in Hebei Province, China. Microb Ecol. 2011;61(4):917–31. https://doi.org/10.1007/s00248-011-9820-0.
Lin DX, Man CX, Wang ET, Chen WX. Diverse rhizobia that nodulate two species of Kummerowia in China. Arch Microbiol. 2007;188(5):495–507. https://doi.org/10.1007/s00203-007-0271-4.
Liu TY, Li Y, Liu XX, Sui XH, Zhang XX, Wang ET, Chen WX, Chen WF, Puławska J. Rhizobium cauense sp. nov., isolated from root nodules of the herbaceous legume Kummerowia stipulacea grown in campus lawn soil. Syst Appl Microbiol. 2012;35(7):415–20. https://doi.org/10.1016/j.syapm.2012.08.006.
Park KY, Lee S-H, Min B-K, Lee KS, Choi J-S, Chung SR, Min KR, Kim Y. Inhibitory effect of luteolin 4′-o-glucoside from Kummerowia striata and other flavonoids on interleukin-5 bioactivity. Planta Med. 1999;65(05):457–9. https://doi.org/10.1055/s-2006-960812.
Shiro S, Matsuura S, Saiki R, Sigua GC, Yamamoto A, Umehara Y, Hayashi M, Saeki Y. Genetic diversity and geographical distribution of indigenous soybean-nodulating bradyrhizobia in the United States. Appl Environ Microbiol. 2013;79(12):3610–8. https://doi.org/10.1128/AEM.00236-13.
Tian CF, Zhou YJ, Zhang YM, Li QQ, Zhang YZ, Li DF, Wang S, Wang J, Gilbert LB, Li YR, Chen WX. Comparative genomics of rhizobia nodulating soybean suggests extensive recruitment of lineage-specific genes in adaptations. Proc Natl Acad Sci U S A. 2012;109(22):8629–34. https://doi.org/10.1073/pnas.1120436109.
Wei GH, Wang ET, Tan ZY, Zhu ME, Chen WX. Rhizobium indigoferae sp. nov. and Sinorhizobium kummerowiae sp. nov., respectively isolated from Indigofera spp. and Kummerowia stipulacea. Int J Syst Evol Microbiol. 2002;52(6):2231–9. https://doi.org/10.1099/00207713-52-6-2231.
Yan J, Han XZ, Ji ZJ, Li Y, Wang ET, Xie ZH, Chen WF. Abundance and diversity of soybean-nodulating rhizobia in black soil are impacted by land use and crop management. Appl Environ Microbiol. 2014;80(17):5394–402. https://doi.org/10.1128/aem.01135-14.
Yan H, Ji ZJ, Jiao YS, Wang ET, Chen WF, Guo BL, Chen WX. Genetic diversity and distribution of rhizobia associated with the medicinal legumes Astragalus spp. and Hedysarum polybotrys in agricultural soils. Syst Appl Microbiol. 2016;39(2):141–9. https://doi.org/10.1016/j.syapm.2016.01.004.
Yan J, Chen WF, Han XZ, Wang ET, Zou WX, Zhang Z. Genetic diversity of indigenous soybean-nodulating rhizobia in response to locally-based long term fertilization in a Mollisol of Northeast China. World J Microbiol Biotechnol. 2017;33(1):6. https://doi.org/10.1007/s11274-016-2170-9.
Yang SH, Chen WH, Wang ET, Chen WF, Yan J, Han XZ, Tian CF, Sui XH, Singh RP, Jiang GM, Chen WX. Rhizobial biogeography and inoculation application to soybean in four regions across China. J Appl Microbiol. 2018;125(3):853–66. https://doi.org/10.1111/jam.13897.
Yao LJ, Shen YY, Zhan JP, Xu W, Cui GL, Wei GH. Rhizobium taibaishanense sp. nov., isolated from a root nodule of Kummerowia striata. Int J Syst Evol Microbiol. 2012;62(2):335–41. https://doi.org/10.1099/ijs.0.029108-0.
Yu XM, Cloutier S, Tambong JT, Bromfield ESP. Bradyrhizobium ottawaense sp. nov., a symbiotic nitrogen fixing bacterium from root nodules of soybeans in Canada. Int J Syst Evol Microbiol. 2014;64(Pt 9):3202–7. https://doi.org/10.1099/ijs.0.065540-0.
Zhang YM, Li Y Jr, Chen WF, Wang ET, Tian CF, Li QQ, Zhang YZ, Sui XH, Chen WX. Biodiversity and biogeography of rhizobia associated with soybean plants grown in the North China Plain. Appl Environ Microbiol. 2011;77(18):6331–42. https://doi.org/10.1128/aem.00542-11.
Author information
Authors and Affiliations
Corresponding author
Rights and permissions
Copyright information
© 2019 Springer Nature Singapore Pte Ltd.
About this chapter
Cite this chapter
Chen, W.F. (2019). Environmental Determinants of Biogeography of Rhizobia. In: Ecology and Evolution of Rhizobia. Springer, Singapore. https://doi.org/10.1007/978-981-32-9555-1_9
Download citation
DOI: https://doi.org/10.1007/978-981-32-9555-1_9
Published:
Publisher Name: Springer, Singapore
Print ISBN: 978-981-32-9554-4
Online ISBN: 978-981-32-9555-1
eBook Packages: Biomedical and Life SciencesBiomedical and Life Sciences (R0)