Abstract
This study presents a numerical analysis of spot-welded DP600 steel sheets to evaluate the strength of the spot-welded joint and damage model under tensile-shear loading conditions. The quality of spot-welded joint is highly dependent on process parameter, material properties and setting on the equipment. Experimental testing is commonly used method to validate the spot-weld quality, but it is costly and time consuming. The present work aims to verify the capability of FEM simulation to model the spot-welding process and generate a reliable result. The spot weld is modeled according to a standardized dimension using a non-linear FEM simulation. The main parameters used to simulate the spot-welding process are current, electrode pressure, and weld cycle. The boundary conditions were set-up in the simulation according to mechanism applied in real application and experiment. As spot weld is governed by electrical-thermal, mechanical, and metallurgical, it has been observed that the material plasticity, thermal and electrical contact conductivity are critical input in spot welding simulation. The simulation result obtained from this study is useful for future works to validate the spot-welding behavior in the FE simulation compared with experiment.
Access provided by Autonomous University of Puebla. Download conference paper PDF
Similar content being viewed by others
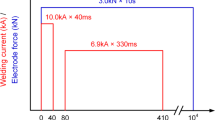
Keywords
1 Introduction
Resistance spot welding is a widely used metal joining technique for metal sheets and applied in various industries such as automotive, aerospace, rail, metal furniture, etc. Despite the advancement of technology of other welding techniques, spot welding remains relevant especially for mass production due to its advantage of time and cost efficient. This joining technique was proven to have high adaptability for automation and robotization. Approximately over 4000 individual spot welds were used in vehicle assembly [1].
Automakers research and development is aiming at producing a lightweight and fuel-efficient vehicle especially with the increasing trend of global crude oil prices. Spot weld satisfies this requirement as the joining does not require any additional materials which may increase the vehicle weight.
To reduce further the vehicle weight, vehicle manufacturer favored use of conventional and advanced high strength steels (AHSS) against traditional mild steels. Dual-phase steel (DP) is commonly used advanced high strength steels in vehicle structure to reduce the vehicle weight while retaining strength. The use of AHSS in modern automotive can be as high as 80% [2].
Spot-welded joint occurred through a formation of weld nugget which produced as a result of heat generated through a combined contact and material resistance to the flow of high localized electrical current at low voltage [3]. The spot-welded joint quality is highly dependent on the process parameter such as weld current and resistance. Other criteria that can affect spot welding performance are sheet thickness, cross-sectional area, and contact surfaces of the material and welding tips [4]. The quality of the spot-welded joint can be verified through an experimental method such as microstructure examination and destructive test such as shear test, cross tension test, peel testing and hardness measurements. However, all these experimental procedures are too costly and if done repetitively increase waste in materials.
Finite element modeling (FEM) is known as time and cost-efficient technique which helps researcher to visualize, analyze the problem with the input of relevant data [5]. However, not all available modelling software can effectively simulate the spot-welding process due to complexity of the actual process as it is governed by electrical-thermal, mechanical, and metallurgical phenomena. To solve these complex problems, a non-linear FE based software MSC Marc/Mentat was used to establish the relevant finding.
Zoltan Bezi and their respective co-authors are among researchers that actively published commendable works and extensive research on the application of similar software to study the characteristic and behavior of spot-welded metal sheets [6]. The present work aims to verify the capability of FEM simulation to model the spot-welding process and generate a reliable result to be used for further comparison with the experiment.
2 Basic Principle of Resistance Spot Welding
Resistance spot welding process is derived according to Ohm’s law, where I is current, E is the voltage drop across the electrodes and R is the resistance through the material in ohms [7]. R is the summation of the contact resistances and the resistance of the work to be welded. Therefore, for a given value of R, the magnitude of I is determined by E. Current to the primary of the transformer is controlled which, in turn, determines the current delivered to a weld of a given resistance. The resistance spot welding process can be defined by the mathematic equation as follow:
The total heat in watt-seconds generated in the work being welded and in the welding electrodes is expressed by the formula:
(T is time in seconds).
The spot-welding cycle consists of four-time segments: squeeze, heat/weld, hold, and off [8]. Figure 1a shows the example of the spot weld model and Fig. 1b the spot-welding cycle of the two key parameters, weld current and electrode force.
-
Squeeze time: the time between pressure application and weld.
-
Heat or weld time: interval of welding current flows through the circuit (60 cycles = 1 s).
-
Forge/Hold time: welding current is off, the electrode force is held
-
Off-time: interval from the end of the hold time until the beginning of the squeeze time for the next cycle. Retract the electrodes, remove or reposition the workpiece.
3 Simulation Set-up and Procedure
3.1 Materials Property and Process Parameter
The simulation of spot-welding process in this study was carried out using non-linear Finite Element Modelling (FEM) software, MSC Marc/Mentat. The component of resistance spot weld in this simulation consists of 2 pieces of DP600 metal sheets with a dimension of 100 mm length × 45 mm width × 1 mm thickness while the two electrodes are copper (cu-sw) with a truncated cone shape. Figure 2 illustrates the dimension of the metal sheets and electrodes.
The main parameter used in the simulation is shown in Table 1.
The material data for DP600 sheets were generated using software jmatpro which then imported to another FEA software simufact to meet the requirement of application in MSC Marc/Mentat. Table 2 represents the chemical composition of DP600 which was used to generate the material data of the metal sheets in the simulation.
3.2 Simulation Procedure
The FEA simulation of spot-welding process in MSC Marc/Mentat can be divided into 3 phases, pre-processing, solving and post-processing. Figure 3 shows the flow of input required for spot weld simulation in MSC Marc/Mentat.
Figure 4 shows tetrahedral-auto mesh which was used to develop the electrodes which have more complex in its shape.
Figure 5 shows the hexahedron-mesh which was used to develop the sheets which have a geometric shape that is relatively simplified. Since less strain and change of shape are expected, using a simplified mesh is done to save computing time and ease the modelling effort. Before drawing the geometry, analysis class of current, thermal and structural were selected. The geometry for each of the components was drawn according to the specified dimension.
Tables and coordinate systems were used to accommodate the material mechanical and thermal properties which mostly are time and temperature-dependant. Figure 6 shows one of the temperature-dependant material properties of DP600 used in the simulation.
Contact interaction is important input for spot weld simulation in MSC Marc/Mentat. Other than material resistance, contact conductivity/resistivity is an integral part of total resistance to generate sufficient heat to achieve the desired temperature in spot weld. The contact conductance/resistance consists of electrical and thermal conductivity/resistivity at the surface which is caused by a film of foreign material such as an oxide. Table 3 shows the thermal and electrical contact conductivity of DP600.
Material plasticity input is crucial in any FEA simulation including spot welding. Analysis of behaviour in the plastic region will allow a better estimation of stresses during structural analysis. For spot welding simulation in MSC Marc/Mentat the plasticity file was generated from the material flow curves which was obtained during material data generation using software jmat.pro and simufact welding. Figure 7 shows the flow curves of DP600 with grain size of 7-micron, used in the simulation.
The boundary conditions that were used to simulate the spot-welding process in MSC Marc/Mentat are structural fixed displacement, structural point load, thermal fixed temperature, current/thermal fixed voltage, and current/thermal point current. All these boundary conditions were applied along the welding, cooling and tensile process. Most of these boundary conditions are time-dependent and activated simultaneously.
Bonora damage model was used in the simulation to observe the damage condition in the material. Bonora uses continuum damage mechanics (CDM) as the basis to derive the evolution of damage in the material. The damage evolution consists of pore initiation, growth and coalescence. The Bonora damage model utilizes the current state of the stress to determine Young’s modulus changes with damage [9]. Table 4 shows the damage properties used in the simulation.
The incremental damage is given as follows:
The effective Young’s Modulus is given as follows:
To evaluate the strength of the spot-welded joint, the tensile mechanism was set-up with clamping at the top sheet and tensile force is applied at the bottom sheet. The set-up of the tensile test mechanism in the simulation is shown in Fig. 8.
A loadcase is created to ensure all the boundary conditions created in the simulation file will be activated following the right order as required. Loadcases allow seamless selection of the data input for activation without deleting other data input which may be required to be used later at different stages in the simulation. Loadcase also allows for the total time of the simulation to be set depending on the time required for each of the time-dependant parameters. Once the loadcase is defined, the simulation can be run through the job properties setting. This is the stage where the result of the simulation can be analyzed.
The result of the simulation can be read in many ways, whether from visualization of the element’s condition or each of the post simulation parameters. For spot weld, the heat is generated at the sheet in between the two electrodes. Therefore, weld temperature is expected to peak at the centre of the spot-welded joint. From the temperature distribution and visualization, the area of the fusion zone and HAZ can be determined in the simulation. For further verification, some physical tests can be done to the element to verify whether the result meets all the requirements.
4 Result and Discussion
For successful simulation of resistance spot welding process in MSC Marc/Mentat, all the input parameters must be clearly defined. Any disagreement of the value may lead to simulation error or the end-result may not as predicted. Figure 9a shows the temperature distribution along the region of the spot-welded joint. The weld nugget size was able to be determined based on temperature in the region of 1100–1386 °C. Figure 9b illustrates the condition of the sheets after being subjected to a tensile load.
Additionally, subroutine was used to define the joint which only occurs at nodes that reached the predefined temperature. The developed subroutine of glue deactivation was applied in the simulation as follow:
-
subroutine uactglue (node,iswitch,inc,time,coord,disp,temp,
-
stressnorm,stressfric,relvel,ibody,ibody2)
-
iswitch set to 1 if node should be switched to regular glue
-
user subroutine for switching nodes that have deact glue to use regular glue.
-
if(temp(1).gt.1300.0d0)then
-
iswitch = 1
-
write(6,*)’switch the following node to regular glue’,node
-
write(6,*)’temperature is’,temp(1)
Without subroutine, the spot-welded joint will not be able to be defined. Figure 10 shows the simulation result without applying the relevant subroutine. It was observed that the two metal sheets were detached from each other once the electrode is released.
In RSW simulation, electrical/thermal contact resistance/conductance is highly significant to achieve the desired results. In most cases, inaccurate value of these parameter for a specified material will lead to insufficient amount of total resistance which affect the welding temperature. Figure 11 shows the resistances involved during spot welding process and the effect to the welding temperature. R1, R3, R5 and R7 represents the bulk resistance of the electrodes and sheets while R2, R4 and R6 indicates the contact resistance which occurs between the interface that connecting electrode to sheet and sheet to sheet.
Resistances during resistance spot welding process [10]
5 Conclusion
From the finding obtained through this study, several key understanding can be established. In conclusion, physical optimized process parameter is crucial for successful FE simulation of spot-welding process. Main Parameter value, material property and boundary conditions are known as key contributing factor to the success of FE simulation of spot-welding process. Most of the data used are not constant. They are either time or temperature dependent. Based on the simulation result, it was found that weld current and electrode pressure are significant. Tensile stress characterization shows that the weld current and tensile strength is directly proportional to each other, satisfies earlier theoretical assumption. The weld nugget will be produced only when the weld temperature achieved the material melting temperature. To achieve this, appropriate value of total resistance which consist of material and contact resistance for both thermal and electrical must be applied in the simulation.
Extensive works on the same subject are required to establish deeper understanding, especially on the thermal changes during the RSW process as well as other characteristics that can lead to further improvement of the mechanical and thermal properties at the weld nugget and HAZ which will affect the tensile strength of the spot-welded joint. This includes experimental investigation of spot welding of the same material to be further compared with the simulation. Application of scanning electron microscope (SEM) may be used to obtain a fuller apprehension of the phases of the welded zone and development of the weld nugget. Furthermore, damage model and result obtained will be compared with experiment to investigate the damage behavior under the same tensile-loading condition.
References
Pirondi A, Bonora N (2003) Modeling ductile damage under fully reversed cycling. Comput Mater Sci 26:129–141
Baskoro AS, Trianda MR (2014) Effects of welding time and welding current to weld nugget and shear load on electrical resistance spot welding of cold rolled sheet for body construction. In: 2014 IEEE international conference on electrical engineering and computer science. Bali, Indonesia: IEEE
Andersson J, Deleskog J (2014) Fatigue life and stiffness of the spider spot weld model. Master’s Thesis, Publication Library, Chalmers University of Technology, Sweden
Prém L, Bézi S (2017) Development of complex spot welding technologies for automotive DP steels with fem support. Springer International Publishing AG 2017, Miskolc, Hungary
Pouranvari M, Marashi SP (2013) Critical review of automotive steels spot welding: process, structure and properties. ResearchGate, Tehran, Iran
Hamedi M, Atashparva M (2016) A review of electrical contact resistance modeling in resistance spot welding. In: IIW2016, Springer
Raut M, Achwal V (2014) Optimization of spot welding process parameters for maximum tensile strength. Int J Mech Eng Robot Res 3(4):506–517
Szávai S, Bézi Z (2014) Experimental and numerical analysis of resistance spot welded joints on DP600 sheets. ResearchGate, Miskolc, Hungary
Nasir Z, Khan DM (2016) Resistance spot welding and optimization techniques used to optimize its process parameters. Int Res J Eng Technol (IRJET) 03(05):887–893
Bézi Z, Baptiszta B (2014) Simulation and validation of welded joints of high strength steel sheets. In: 6th International conference from “scientific computing to computational engineering”, vol II, pp. 452–459, Athens: ResearchGate. ISBN:978-614-80527-4-1
Author information
Authors and Affiliations
Corresponding author
Editor information
Editors and Affiliations
Rights and permissions
Copyright information
© 2021 The Author(s), under exclusive license to Springer Nature Singapore Pte Ltd.
About this paper
Cite this paper
Yusuf, M.N., Rahaman, W.E.W.A., Manurung, Y.H.P., Rafie, M.A., Reduan, M.S., Fadhil, M.Z.H. (2021). Numerical Analysis and Modelling of Resistance Spot Welded DP600 Steel Sheets. In: Osman Zahid, M.N., Abdul Sani, A.S., Mohamad Yasin, M.R., Ismail, Z., Che Lah, N.A., Mohd Turan, F. (eds) Recent Trends in Manufacturing and Materials Towards Industry 4.0. Lecture Notes in Mechanical Engineering. Springer, Singapore. https://doi.org/10.1007/978-981-15-9505-9_63
Download citation
DOI: https://doi.org/10.1007/978-981-15-9505-9_63
Published:
Publisher Name: Springer, Singapore
Print ISBN: 978-981-15-9504-2
Online ISBN: 978-981-15-9505-9
eBook Packages: EngineeringEngineering (R0)