Abstract
Vaccines used for managing Newcastle disease virus (NDV) rely heavily on cold chain, and this results in major constraints in their successful application, shipping, and storage. This study was undertaken to improve the thermotolerance properties of live attenuated NDV vaccines using vacuum foam drying (VFD) technology. The live attenuated NDV vaccine formulated in 15% trehalose, 2.5% gelatin, 0.05% pluronic, and 25 mmol/L potassium phosphate buffer (T5) and dried using VFD showed improved heat tolerance in comparison to the vaccine formulated in T5 as well, but dried using freeze-drying (FD) method. The T5-formulated VFD vaccine was stored at 37°C for 120 days, 45°C for 7 days, and 60°C for 3 days; the virus titer loss decreased by no more than 1.0 Log10. In contrast, the FD vaccine prepared in T5 could only be stored at 37°C for 7–10 days. Furthermore, the T5-formulated NDV-VFD vaccine remained infectious when heated at 100°C for 30 min. Shelf-life studies confirmed the improved thermal tolerance of the T5-formulated NDV-VFD vaccine since it could be stably stored at 2–8°C for 42 months and 25°C for 15 months. Moreover, immunization of 1-month-old specific pathogen-free (SPF) chickens with the T5-formulated NDV-VFD vaccine stored at 25 and 37°C could produce hemagglutination inhibition (HI) antibody levels comparable to those of commercial NDV-FD vaccines, which require strict adherence to the cold chain. In conclusion, not only did the VFD technology improve the thermostability and long-term shelf life of the vaccine, it also maintained its immunogenicity.
Graphical Abstract

Similar content being viewed by others
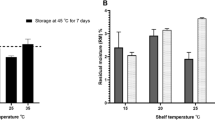
Avoid common mistakes on your manuscript.
Introduction
Newcastle Disease (ND) is a highly contagious disease in poultry caused by a paramyxovirus, with mortality rates of up to 100%, depending on the strain causing the outbreak [1, 2]. Consequently, ND is classified as a Class A animal disease by the World Organization for Animal Health (OIE) [3]. Vaccination with live attenuated vaccines based on the LaSota strain is widely used to reduce the negative impact of this viral disease [4, 5]; however, the LaSota strain live attenuated vaccine is heat-labile, thus requiring strict storage and handling of the vaccine, at temperatures between 2 and 8°C or at −15°C [6, 7].
Almost all commercial live attenuated vaccines for ND are developed using the freeze-drying (FD) technology [8], in which a mixture of antigen and heat-resistant formulation is frozen below the eutectic point in the presence of excipients such as trehalose and sucrose, followed by vacuum drying for sublimation [9, 10]. Exposure to temperatures beyond the recommended storage conditions for vaccines prepared using FD greatly reduces the efficacy of these vaccines, resulting in a major drawback in the widespread application of live attenuated vaccines. This is especially prevalent in hot climate regions, distant regions, and small-scale poultry farmers [10,11,12]. Therefore, novel ways to improve the thermotolerance properties of these vaccines will have a positive impact on the management of ND since it will overcome challenges experienced with strict cold-chain compliance required in the handling, shipping, and administering of these vaccines [13].
Vacuum foam drying (VFD) is one of the alternative methods used to improve the thermotolerance properties of heat-sensitive biotherapeutics [14, 15]. This scalable technology preserves sensitive biotherapeutics in the dry state using gradient vacuum evaporation instead of sublimation used in lyophilization [15,16,17]. The potential application of VFD has been demonstrated for a number of vaccines such as the live attenuated porcine reproductive and respiratory syndrome (PRRS) vaccine [14], the Salmonella Typhi vaccine [18], the live attenuated NDV vaccine [19, 20], and the live attenuated influenza vaccine [21]. These studies showed that VFD vaccines with a high glass transition temperature could be preserved for some time at room temperature without significant loss of efficacy compared to FD vaccines [22]. Furthermore, the VFD technology is advantageous over FD because the freezing process during drying, which may cause mechanical damage to the products due to the formation of ice crystals, is avoided [23,24,25]. Although the VFD method has been shown to improve the thermotolerance properties of the live attenuated LoSota strain vaccine, very little is known about the long-term thermal stability and heat resistance of these vaccines beyond 40°C. This acknowledged gap needs to be addressed, especially considering the significant economic and logistical impacts it has on developing countries, remote areas, and small-scale farmers associated with vaccination programs [1, 7]. Therefore, in this study, VFD was used to prepare a live attenuated vaccine with various excipients. Preparations that passed the two rounds of screening were then profiled for long-term stability, efficacy, and potency.
Materials and Methods
Materials
The live attenuated NDV (LaSota strain) was obtained from the National Institute of Veterinary Drug Control (Beijing, China). The specific pathogen-free (SPF) chicken eggs were purchased from Beijing Boehringer Ingelheim Viton Biotechnology Co., Ltd. The NDV solution used in this study was amplified by inoculation into the allantoic cavity of 9–11-day-old embryonated SPF eggs. Trehalose dihydrate (Tre), sucrose (Suc), gelatin (Gel), pluronic F127, and potassium phosphate (KPO4) were purchased from Sigma-Aldrich (St. Louis, MO, USA).
Formulation Design and NDV Formulation Preparation
The NDV solution was formulated with an equal volume ratio of heat resistance protective agents such as trehalose dihydrate and pluronic F127 that consisted of low, medium, and high sugar concentrations. These additives were selected based on preliminary studies (data not shown). The thirteen studied formulations and their compositions are shown in Table I. The prepared vaccine solutions were packed into 10 mL vials with 1.0 mL per vial and sealed with rubber stoppers half-opened and then either vacuum foam-dried or freeze-dried. For vacuum drying, the prepared mixture of virus and formulation was placed into a lyophilizer (SP Scientific, VirTis AdVantage Pro, Laboratory Benchtop Freeze Dryer, Warminster, PA, USA) and kept for 30 min after the shelf temperature reached 25–30°C. The pressure of the dryer chamber was decreased in a stepwise manner from 60,000 to 300 Pa over 1 h. Then, the vacuum pressure was decreased to 10 Pa over 12 h. Finally, the chamber’s pressure was decreased to 1.0 Pa for 18–24 h. The whole drying process was performed over 30–42 h.
For shelf freeze-drying, formulations to be lyophilized were frozen to −45°C at a rate of 1°C/min. The primary drying was performed at −10°C at a rate of 0.5°C/min and then held for 12–18 h, and the secondary drying was performed at 25–28°C at a rate of 1°C/min and then held for 6–8 h. The whole drying process was performed over 26–36 h. The vacuum pressure was maintained at < 10 Pa.
Screening of Heat-resistant NDV Formulations
The heat resistance profiles of the VFD NDV formulations were evaluated using the heat challenge experiment [6, 25]. Lyophilized vaccines were qualified when the residual moisture content was <3%, and the virus titer loss was < 1.0 Log10 after storage at 37°C for 10 days [7]. Therefore, this criterion was used to initially eliminate formulations that resulted in residual water of > 3% and virus titer loss of > 1.0 Log10 after VFD after storage at 37°C for 10 days. We introduced a second round of screening for VFD NDV formulations that displayed promising heat resistance profiles. Formulations with no more than 1.0 Log10 titer loss at 37°C for 20 days and 45°C for 7 days were selected as the optimum formulations. The virus titer was detected by the egg infectious dose 50 (EID50) method as described by Reed and Muench [26].
The residual moisture content of the VFD-prepared vaccine formulations was determined by thermogravimetric analysis [7, 9, 27]. Briefly, eight vials of each VFD vaccine formulation were weighed before and after drying at 80°C for 2 h without a stopper. The moisture percentage was calculated using the following equation: RM%= [(initial weight − final constant weight)/initial weight]×100. Based on the stated criteria, the vaccine prepared using the T5 formulation was selected for further characterization.
Thermal Stability of T5-Formulated VFD and FD Vaccine
Considering that the ambient temperature of prepared vaccines may rise to 40–60°C due to the break in cold-chain during storage and transportation, it became imperative to investigate the thermal stability of T5-formulated VFD and FD vaccines. Therefore, the selected VFD vaccine with T5 formulations underwent an accelerated stability test by placing them at 37°C, 45°C, 60°C, and 100°C for different periods to determine their thermotolerance properties. Meanwhile, the FD vaccine prepared with T5 formulation was used as a control. The samples were taken at different time points to measure the EID50 [7] until the titer loss decreased by 1.0 Log10.
Physical Characterization of T5-Formulated NDV Vaccine
The micro-appearance of VFD and FD-dried T5 vaccine formulation was observed using a scanning electron microscope (SEM) (s-3400n II, Japan Hitachi) to evaluate their morphological characteristics. The glass transition temperature (Tg) of the dried T5 vaccine formulation was detected by a differential calorimeter scanner (DSC) (Pyris1 DSC, Perkin-Elmer Life Sciences, Waltham, MA, USA). Samples in the range of 100–200 mg were analyzed in crimped, vented aluminum pans under a dry nitrogen purge with an automated liquid nitrogen-cooling accessory. The samples were heated from −50 to 150°C with a scanning rate of 10°C/min.
Long-Term Thermal Stability of T5-Formulated VFD and FD NDV Vaccines
The T5 VFD NDV vaccine formulations were stored at 4°C and 25°C to investigate their long-term thermal stability. The vaccine potency was assayed by determining the EID50 every 2 months for samples stored at 25°C and every 6 months for samples stored at 4°C.
The potency of the vaccine formulations was detected using SPF chicken eggs, as described by Osman et al. [7]. Briefly, the vaccine formulations were reconstituted to 1 mL using a normal saline solution. Ten-fold serial dilutions were prepared using normal saline solution, and 0.1 mL of each dilution was inoculated in the allantoic sacs of five 10-day-old embryonated SPF chicken eggs. The chicken embryo allantoic fluid’s hemagglutinin activity (HA) was tested after 5 days of culturing. Eggs that were HA-positive but negative for bacteria were considered infected. The EID50 was calculated according to the method described by Reed and Muench [26]. The values were normalized to the vaccine dose within vaccine vials.
Immunogenicity Study
Fifty 1-month-old healthy SPF chickens were divided into five groups (groups A, B, C, and D and the control group). Groups A, B, and C were immunized with the VFD vaccines, which were stored at 2–8°C (for 15 months), 25°C (for 15 months), and 37°C (for 4 months), respectively. Group D was immunized with the commercial FD vaccine stored at 2–8°C for 15 months, and the control group was not immunized. The hemagglutination inhibition (HI) antibody was detected just before vaccination and at 7, 14, and 21 days after immunization to confirm vaccine immunity.
Statistical Analysis
The data were presented as mean ± standard deviation or n (%). Graphs were generated using Prism 6.0 (GraphPad Software Inc., San Diego, CA, USA).
Results
Screening of the Heat-Tolerance and Residual Water Content of the Formulations
Thirteen formulations of live attenuated NDV vaccines (Table I) were prepared and dried using VFD. These VFD samples were screened for improved thermal stability to determine optimal formulation conditions. All 13 formulations were initially screened in an accelerated stability analysis at 37°C for 10 days, and the residual moisture content of each formulation was analyzed. Formulations that contained a residual water content exceeding 3% and had a potency loss of greater than or close to 1.0 Log10 at 37°C for 10 days were discontinued. Therefore, based on the results shown in Table II, formulations T4, T5, and T6, which contained 15% trehalose, were selected for the second round of screening since they passed the initial residual water content and heat resistance screening tests.
In the second screening round, the titer loss of the ND virus was evaluated after storage at 37°C for 20 days and 45°C for 7 days. Table III shows that extended storage at 37°C for up to 20 days for all three formulations did not significantly affect vaccine potency since all three formulations had a titer loss of 1.0 Log10 or less. However, an increase in storage temperature from 37 to 45°C resulted in significant titer loss for T4 and T6 formulations. Both these formulations resulted in titer losses of greater than 1.0 Log10. In contrast, the T5 formulation displayed better thermal properties, withstanding an increased temperature of up to 45°C. T5-formulated NDV vaccine stored for up to 7 days at 45°C resulted in a potency titer loss of less than 1.0 Log10.
Physical Characterization of T5 Formulation Dried Using VFD and FD
Following the successful determination of a suitable formulation for the NDV vaccine, we then sought to determine its physical characteristics. T5-formulated NDV was subjected to VFD or FD and then analyzed using SEM and DSC. The VFD process required the vacuum pressure to be decreased gradually and for the sample to be dried by bubbling and evaporation at low pressure (Fig. 1a). As a result, the sample became viscous, then bubbled gradually, forming a dry foam as seen in Fig. 1a. On the other hand, drying the T5 formulation using FD adopted the classical three-step method: pre-freezing, primary drying, and secondary drying, resulting in a dry cake-like pellet (Fig. 1b). Both the VFD and FD T5 NDV samples were further analyzed by SEM. Figure 2 shows that the T5-formulated VFD vaccine presented a regular, glass-like lamellar structure while the FD vaccine showed a regular porous structure. This observation was consistent with the principle of evaporation in the former (VFD) and sublimation in the latter (FD) method [18, 28].
Schematic representation of the drying technology and the appearance of vacuum foam-dried (VFD) and freeze-dried (FD) vaccines. Shown in a is the VFD process where the sample to be dried is converted into a dried foam structure in a single step. The overall VFD process involves boiling or foaming the sample under gradient pressure prior to rapid evaporation, resulting in the sample forming a foam-like structure. In contrast, the sample in FD (b) is frozen, as the vacuum descends rapidly and continues to heat in successive stages. The water sublimates, eventually forming a dried cake
The Tg is one of the properties used to characterize the thermal properties of substances. Studies have shown that there is a direct relationship between Tg of the dried product and its storage temperature [29]. Formulations with higher Tg have been found to inhibit recrystallization, subsequently leading to reduced water content during the drying process, thus improving stability [30]. The Tg of the T5-formulated NDV vaccine was determined to be 56.87±1.68°C and 31.03±1.74°C for VFD and FD-dried vaccines, respectively (Table IV). The Tg of the VFD vaccine was 25°C higher than that of the FD vaccine, suggesting better thermal stability of the sugar-glass vaccine, and less dependency on strict cold chain conditions recommended for live attenuated vaccines.
Thermal Stability of T5-Formulated VFD Vaccine
Given the temperature sensitivity of the live attenuated vaccines, we evaluated the heat resistance of T5-formulated VFD vaccine by incubating the vaccine preparations at 37°C, 45°C, 60°C, and 100°C for various intervals. Subsequently, the vaccine potency was determined at different time intervals by measuring the EID50. The T5-formulated NDV vaccine dried using VFD showed improved thermal stability compared to the FD-dried vaccine, as shown in Fig. 3. The VFD-prepared vaccine titer loss remained less than 1.0 Log10 for up to 120 days at 37°C, while the virus titer loss of the FD vaccine was 1.05±0.19 Log10 at 10 days when incubated at the same temperature (Fig. 3A). A similar trend was observed at 45°C where the FD-prepared vaccine titer loss was more than 1.0 Log10 at 3 days, whereas the VFD-prepared vaccine titer loss was less than 1.0 Log10 for the same duration and only reached 1.1±0.29 Log10 after 9 days (Fig. 3B). As anticipated, the VFD-prepared vaccine’s titer loss was much more rapid at higher temperatures (60°C and 100°C) compared to 37°C. VFD-prepared vaccines were more stable at 37°C, with titer losses of less than 1.0 Log10 for up to 120 days of incubation, whereas at 60°C virus titer loss was close to 1.0 Log10 (0.89±0.39 Log10) in 3 days (Fig. 3C). Similarly, at 100°C, the potency of the VFD-prepared vaccine was reduced by 1.5±0.19 Log10 in 10 min, which was much shorter than the potency reduction after storage for 120 days at 37°C. It was interesting to note that, although virus titer loss of the VFD-prepared vaccine decreased significantly at 100°C compared to other samples investigated, it remained infectious for up to 30 min of exposure at this high temperature. The FD-prepared vaccine, on the other hand, was completely inactivated after 15 min when heated at 100°C (Fig. 3D).
Long-Term Shelf Life Studies
Three batches of T5-formulated VFD vaccines (NDV-VFD-1, NDV-VFD-2, and NDV-VFD-3) were prepared and preserved at 2–8°C and 25°C for long-term stability studies (Fig. 4). As shown in Fig. 4a, when the vaccines were preserved at 25°C for 15 months, the virus infectivity titer of the three batches of T5-formulated vaccine decreased by 1.06±0.09 Log10, 1.06±0.09 Log10, and 1.0±0.11 Log10, respectively. Furthermore, the virus infectivity titer of the three batches of T5-formulated vaccine decreased by 0.89±0.09 Log10, 0.89±0.09 Log10, and 0.79±0.12 Log10, respectively, after 42 months at 2-8°C (Fig. 4b).
Immunogenicity Study
The immunogenicity and protective capacity of the T5-formulated VFD vaccine were evaluated in an animal trial and the results are presented in Fig. 5. One-month-old SPF chickens were inoculated intranasally with VFD vaccine preparations stored at different temperatures. The commercial FD vaccine (used as a positive control) was stored at 2–8°C. All the vaccine preparations had the same initial potency at the commencement of the study. The antibodies against NDV were detected at 7, 14, and 21 days after immunization to confirm the immunogenicity of the vaccine (Fig. 5). Seven days after vaccination, the HI levels of each group were between 2.5 and 4.0. At 14 days post-immunization, all the immunized groups exhibited an HI of greater than 4, which thereafter increased to greater than 6 at 21 days. The HI in group C was lower than that of group D 7 days following immunization, but reached the level of group D rapidly after 14 and 21 days. Therefore, the data obtained in this study demonstrates the enhanced thermal stability of the T5-formulated VFD NDV vaccine, which showed no dependence on cold chain conditions. This was supported by the observation that, after being stored at 25°C and 37°C for 15 months and 4 months, respectively, the T5-formulated VFD NDV vaccine could still produce HI antibody levels comparable to those of commercial FD vaccines, which are dependent on cold chain conditions.
HI antibody titers of vacuum foam drying (VFD) and freeze-drying (FD) vaccines after intranasal inoculation. Groups A, B, and C were immunized with the VFD vaccines. Group A was immunized with the VFD vaccine stored at 2–8°C for 15 months. Group B was immunized with the VFD vaccine stored at 25°C for 15 months. Group C was immunized with the VFD vaccine stored at 37°C for 4 months. Group D was immunized with the commercial FD vaccine stored at 2–8°C for 15 months. The control group was not immunized
Discussion
Improving the thermostability of vaccines is an important research topic, which is highlighted as a grand challenge in pursuit of global health by the Gates Foundation [31, 32]. FD is a conventional method for the preparation of commercial live vaccines, where a live attenuated virus is dried after production for preservation by lyophilization, and then reconstituted just before vaccination [8, 29]. Despite the success of using FD in vaccine production, there are challenges encountered during the vaccine preparation process [9]. These challenges include mechanical damage caused by the formation of ice crystals during the freezing step as well as dehydration and drying damage during the primary and secondary drying steps [33]. These challenges may reduce the efficacy of the lyophilized vaccine, thus compromising the immunization effect [34,35,36]. Lastly, lyophilized vaccines may be temperature sensitive, and thus dependent on strict adherence to cold chain during processing, storage, and distribution. Importantly, if the cold chain is broken, the immunogenicity of the vaccine could be compromised [9]. However, maintaining cold chain conditions greatly increases the cost of vaccines [35]. Furthermore, breakage of the cold chain is inevitable due to handling errors and equipment power failures especially in underdeveloped areas [10, 37]. Therefore, new drying techniques are continuously explored to improve the thermal stability of live vaccines. The new drying methods could include alternative drying techniques or improved formulation, or a combination of both [38,39,40].
VFD has been shown to improve the thermal stability of live viruses [14, 22]. In this study, we employed VFD as an alternative drying method and formulated the vaccine in T5 to improve the stability and long-term storage of the live attenuated NDV vaccine. Prior to drying, NDV was formulated with varying concentrations of trehalose or sucrose or a combination of both in the presence of gelatin and pluronic (Table I). Saccharides are widely used to preserve cells, viruses, and bacteria as this serves as a stabilizer to prevent dehydration during the drying process [2, 35, 36]. The viscosity and high heat resistance of the formulation are very important. Furthermore, the consistency of the formula is conducive to the formation of foam, while the high Tg of the formula enhances the stability of the vaccine [18]. The data indicated that formulations containing 15% trehalose (T4, T5, and T6) showed good heat resistance, with the T5 formulation in 25 mM potassium phosphate buffer presenting the best heat resistance. In contrast, formulations containing 15% sucrose (S1–S3) and TS (a combination of trehalose and sucrose) showed poor heat resistance. Sucrose and trehalose are both non-reducing disaccharides, but sucrose has a higher hygroscopicity compared to trehalose and a lower Tg (52–76°C) than that of trehalose (75–120°C) [41]. Therefore, trehalose could foam easily, thus forming a vitrified vaccine [42]. As such, the heat resistance profile of VFD-dried NDV in trehalose-based formulation could be attributed to the properties of this additive. In addition, the results showed that a trehalose concentration of 15% is optimal for the formulation of the VFD-dried NDV vaccine because a higher concentration (30%) resulted in a virus titer loss of greater than 1.0 Log10 and higher residual moisture content. This high residual moisture content could be attributed to the higher concentration of trehalose in the formulation, which increases the viscosity of the formulation, thereby causing insufficient foaming to occur. This result was consistent with the findings of Truong-Le [21]. The addition of gelatin at 2.5% (w/v) and pluronic F127 in the formulation is ideal for the VFD process because these additives reduce surface tension during foaming, thereby reducing mechanical damage to the virus. The pH of the formulation was maintained at pH 7.2–7.4 using potassium phosphate and this buffer also inhibits the formation of free radicals, stabilized the formulation facilitating the foaming processes. This is supported by the immunogenicity profiles of the VFD-dried vaccines (Fig. 5).
In this study, T5 was selected as the optimal formulation after two rounds of screening and residual moisture analysis. Thereafter, T5 VFD and FD-prepared vaccines were subjected to physicochemical and stability analysis. The Tg of the T5-formulated NDV-VFD vaccine was 56.87°C, which was approximately 25°C higher than the Tg of the NDV-FD vaccine. Furthermore, the higher Tg value obtained for the VFD vaccine is consistent with a higher thermal stability observed for the VFD vaccine at 37°C, which is better than that of the FD vaccine. The studies of Kadoya et al. [43] and Alhalaweh et al. [24] reported that if the Tg of the dried product is high and the gap between the Tg and storage temperature is large, then the long-term preservation of the dried product would be improved. Therefore, the high Tg values obtained for T5-formulated VFD vaccines (Fig. 4) could explain the improved stability of the NDV vaccine compared to that of the FD NDV vaccine.
The VFD drying process does not require the vaccine solution to be frozen as in FD, but it is dried at room temperature by bubbling and evaporation in a gradient vacuum, leaving a structure resembling solidified foam (Fig. 1). The microscopic morphology of the T5-formulated dried vaccine also confirmed the difference between the two drying methods. The VFD-prepared vaccine presented a glass-like layer structure in SEM, while the FD-prepared vaccine presented a loose pore structure, which is a channel for ice crystal sublimation (Fig. 2). The resulting ice crystals may cause mechanical damage to the virus during the freeze-drying process, which can be avoided using VFD.
The results showed that the VFD vaccine could be stored at 37°C for 4 months and 25°C for 15 months, with virus loss as low as 1.0 Log10 which demonstrates good thermostability when stored out of cold-chain conditions (Figs. 3A and 4A). Also, when stored at 2–8°C, the VFD vaccine could be kept for 42 months with virus loss no more than 0.9 Log10 (Fig. 4B). One-month-old SPF chickens were inoculated intranasally with VFD vaccines and stored at different temperatures to examine the immunogenic response. As shown in Fig. 5, 7 days after inoculation, the NDV-VFD vaccine could produce HI antibodies after being stored at 2–8°C and 25°C for 15 months, and 37°C for 4 months. Furthermore, 14 days after inoculation, the HI antibody level was positive and similar to the commercial NDV-FD vaccine. It also indicated that the VFD vaccine was stable and effective when stored at high temperatures for long periods and could still produce HI antibodies against the NDV comparable to commercial FD vaccines. Our data are consistent with previous studies which reported that the VFD vaccine can be preserved for a long time out of the cold chain due to its improved thermostability [18].
Conclusions
In this study, live attenuated Newcastle disease vaccine formulated in T5 and VFD-dried demonstrated improved thermostability and long-term shelf life. Furthermore, its immunogenicity profiles are compared to that of an FD-prepared vaccine. This finding demonstrates that the VFD-prepared vaccine may be a powerful substitute for FD vaccines, especially in under-resourced settings where it is difficult to maintain cold chain conditions. In summary, this study provides theoretical support for the development of a cold chain-independent live attenuated Newcastle disease vaccine and further studies should focus on process optimization and scale-up to apply this technology in vaccine manufacturing.
References
Ganar K, Das M, Sinha S, Kumar S. Newcastle disease virus: current status and our understanding. Virus Res. 2014;184:71–81. https://doi.org/10.1016/j.virusres.2014.02.016.
Zhang M, Oldenhof H, Sydykov B, Bigalk J, Sieme H, Wolkers WF. Freeze-drying of mammalian cells using trehalose: preservation of DNA integrity. Sci Rep. 2017;7(1):6198. https://doi.org/10.1038/s41598-017-06542-z.
Huang Y, Liu Y, Li Y, Liu Y, Zhang C, Wen H, et al. Role of key amino acids in the transmembrane domain of the Newcastle disease virus fusion protein. Biosci Trends. 2021;15(1):16–23. https://doi.org/10.5582/bst.2020.03317.
Wang Z, Zhao X, Wang Y, Sun C, Sun M, Gao X, et al. In vivo production of HN protein increases the protection rates of a minicircle DNA vaccine against genotype VII Newcastle Disease virus. Vaccines (Basel). 2021;9(7). https://doi.org/10.3390/vaccines9070723.
Welch CN, Shittu I, Abolnik C, Solomon P, Dimitrov KM, Taylor TL, et al. Genomic comparison of Newcastle disease viruses isolated in Nigeria between 2002 and 2015 reveals circulation of highly diverse genotypes and spillover into wild birds. Arch Virol. 2019;164(8):2031–47. https://doi.org/10.1007/s00705-019-04288-9.
Boumart Z, Hamdi J, Daouam S, Elarkam A, Tadlaoui KO, El Harrak M. Thermal stability study of five newcastle disease attenuated vaccine strains. Avian Dis. 2016;60(4):779–83. https://doi.org/10.1637/11426-042116-Reg.1.
Osman N, Goovaerts D, Sultan S, Salt J, Grund C. Vaccine quality is a key factor to determine thermal stability of commercial Newcastle Disease (ND) vaccines. Vaccines (Basel). 2021;9(4). https://doi.org/10.3390/vaccines9040363.
Zhao Y, Deng B, Pan X, Zhang J, Zuo X, Wang J, et al. Optimization of heat-resistance technology for a duck hepatitis lyophilized live vaccine. Vaccines (Basel). 2022;10(2). https://doi.org/10.3390/vaccines10020269.
Merivaara A, Zini J, Koivunotko E, Valkonen S, Korhonen O, Fernandes FM, et al. Preservation of biomaterials and cells by freeze-drying: change of paradigm. J Control Release. 2021;336:480–98. https://doi.org/10.1016/j.jconrel.2021.06.042.
Fanelli A, Mantegazza L, Hendrickx S, Capua I. Thermostable vaccines in veterinary medicine: state of the art and opportunities to be seized. Vaccines (Basel). 2022;10(2). https://doi.org/10.3390/vaccines10020245.
Chen Y, Liao Q, Chen T, Zhang Y, Yuan W, Xu J, et al. Freeze-drying formulations increased the adenovirus and poxvirus vaccine storage times and antigen stabilities. Virol Sin. 2021;36(3):365–72. https://doi.org/10.1007/s12250-020-00250-1.
Liu ZH, Xu HL, Han GW, Tao LN, Lu Y, Zheng SY, et al. A self-assembling nanoparticle: Implications for the development of thermostable vaccine candidates. Int J Biol Macromol. 2021;183:2162–73. https://doi.org/10.1016/j.ijbiomac.2021.06.024.
Madan M, Sikriwal D, Sharma G, Shukla N, Mandyal AK, Kale S, et al. Rational design of heat stable lyophilized rotavirus vaccine formulations. Hum Vaccin Immunother. 2018;14(9):2132–41. https://doi.org/10.1080/21645515.2018.1487499.
Lv F, Lu Y, Hao ZL, Zhao YH, Zhang LH, Feng L, et al. Preparation and heat resistance study of porcine reproductive and respiratory syndrome virus sugar glass vaccine. Vaccine. 2016;34(33):3746–50. https://doi.org/10.1016/j.vaccine.2016.06.003.
Jangle RD, Pisal SS. Vacuum foam drying an alternative to lyophilization. Indian J Pharm Sci. 2012:91-100.
Bronshtein V. Penfield. Scalable long-term shelf preservation of sensitive biological solutions and suspensions. Patent US 6509146B1. Jan.21, 2003.
Pisal S, Wawde G, Salvankar S, Lade S, Kadam S. Vacuum foam drying for preservation of LaSota virus: effect of additives. AAPS PharmSciTech 2006; 7 (3) Article 60.
Ohtake S, Martin R, Saxena A, Pham B, Chiueh G, Osorio M, et al. Room temperature stabilization of oral, live attenuated Salmonella enterica serovar Typhi-vectored vaccines. Vaccine. 2011;29(15):2761–71. https://doi.org/10.1016/j.vaccine.2011.01.093.
Worrall EE. Method for the preservation of biologically-active material. Patent US 6841168 B1. Jan.11,2005.
Pisal SS, Wawde GM, Bandivadekar MM, Hajare AA, More HN, Kadam SS. Vacuum foam drying for preservation of lasota virus screening. Indian J Biotechnol 2006;10(5):491-497.
Truong-Le V. Preservation of bioactive materials by freeze dried foam. Patent US7135180B2. Nov.14, 2006.
Lovalenti PM, Anderl J, Yee L, Nguyen V, Ghavami B, Ohtake S, et al. Stabilization of live attenuated influenza vaccines by freeze drying, spray drying, and foam drying. Pharm Res. 2016;33(5):1144–60. https://doi.org/10.1007/s11095-016-1860-1.
Abdul-Fattah AM, Truong-Le V, Yee L, Pan E, Ao Y, Kalonia DS, et al. Drying-induced variations in physico-chemical properties of amorphous pharmaceuticals and their impact on stability II: stability of a vaccine. Pharm Res. 2007;24(4):715–27. https://doi.org/10.1007/s11095-006-9191-2.
Alhalaweh A, Alzghoul A, Mahlin D, Bergstrom CAS. Physical stability of drugs after storage above and below the glass transition temperature: relationship to glass-forming ability. Int J Pharm. 2015;495(1):312–7. https://doi.org/10.1016/j.ijpharm.2015.08.101.
Abdul-Fattah AM, Truong-Le V, Yee L, Nguyen L, Kalonia DS, Cicerone MT, et al. Drying-induced variations in physico-chemical properties of amorphous pharmaceuticals and their impact on stability (I): stability of a monoclonal antibody. J Pharm Sci. 2007;96(8):1983–2008. https://doi.org/10.1002/jps.20859.
Reed LJ, Muench H. A simple method of estimating fifty per cent endpoints. Am J Epidemiol. 1938;27:493–7.
Regis F, Arsiccio A, Bourles E, Scutella B, Pisano R. Surface treatment of glass vials for lyophilization: implications for vacuum-induced surface freezing. Pharmaceutics. 2021;13(11). https://doi.org/10.3390/pharmaceutics13111766.
Ashok AHAJARE, Harinath NMORE, Sambhaji SPISAL. Vacuum foam dried sugar-phosphate amorphous mixtures for stabilization of doxorubicin hydrochloride. Lat Am J Pharm. 2010;29(5):738–46.
Zuo XX, Zhao YH, Zhou MX, Deng BH, Hu LG, Lv F, et al. Live vaccine preserved at room temperature: preparation and characterization of a freeze-dried classical swine fever virus vaccine. Vaccine. 2020;38(52):8371–8. https://doi.org/10.1016/j.vaccine.2020.10.093.
Górska A, Szulc K, Ostrowska-Ligęza E, Bryś J, Wirkowska-Wojdyła M. Effect of composition and drying method on glass transition temperature, water sorption characteristics and surface morphology of newly designed β-lactoglobulin/retinyl palmitate/disaccharides systems. J Thermal Anal Calorim. 2017;130(1):177–85. https://doi.org/10.1007/s10973-017-6392-3.
Bajrovic I, Schafer SC, Romanovicz DK, Croyle MA. Novel technology for storage and distribution of live vaccines and other biological medicines at ambient temperature. Sci Adv 2020; 6:eaau4819 4.
Du P, Liu R, Sun S, Dong H, Zhao R, Tang R, et al. Biomineralization improves the thermostability of foot-and-mouth disease virus-like particles and the protective immune response induced. Nanoscale. 2019;11(47):22748–61. https://doi.org/10.1039/c9nr05549e.
Arsiccio A, Giorsello P, Marenco L, Pisano R. Considerations on protein stability during freezing and its impact on the freeze-drying cycle: a design space approach. J Pharm Sci. 2020;109(1):464–75. https://doi.org/10.1016/j.xphs.2019.10.022.
Worrall EE, Litamoi JK, Seck BM, Ayelet G. Xero6ac an ultra rapid method for the dehydration and preservation of live attenuated Rinderpest and Peste des Petits ruminants vaccines. Vaccine. 2001;19:834–9.
Bellali S, Bou Khalil J, Fontanini A, Raoult D, Lagier JC. A new protectant medium preserving bacterial viability after freeze drying. Microbiol Res. 2020;236:126454. https://doi.org/10.1016/j.micres.2020.126454.
Pastorino B, Baronti C, Gould EA, Charrel RN, de Lamballerie X. Effect of chemical stabilizers on the thermostability and infectivity of a representative panel of freeze dried viruses. PLoS ONE. 2015;10(4):e0118963. https://doi.org/10.1371/journal.pone.0118963.
Hansen LJJ, Daoussi R, Vervaet C, Remon JP, De Beer TRM. Freeze-drying of live virus vaccines: a review. Vaccine. 2015;33(42):5507–19. https://doi.org/10.1016/j.vaccine.2015.08.085.
Nurzijah I, Elbohy OA, Kanyuka K, Daly JM, Dunham S. Development of plant-based vaccines for prevention of avian influenza and Newcastle Disease in poultry. Vaccines (Basel). 2022;10(3). https://doi.org/10.3390/vaccines10030478.
Wang G, Li X, Mo L, Song Z, Chen W, Deng Y, et al. Eggshell-inspired biomineralization generates vaccines that do not require refrigeration. Angew Chem Int Ed Engl. 2012;51(42):10576–9. https://doi.org/10.1002/anie.201206154.
Wen G, Chen C, Guo J, Zhang Z, Shang Y, Shao H, et al. Development of a novel thermostable Newcastle disease virus vaccine vector for expression of a heterologous gene. J Gen Virol. 2015;96(Pt 6):1219–28. https://doi.org/10.1099/vir.0.000067.
Roe KD, Labuza TP. Glass transition and crystallization of amorphous trehalose-sucrose mixtures. Int J Food Prop. 2005;8(3):559-574. https://doi.org/10.1080/10942910500269824.
Smith TG, Siirin M, Wu X, Hanlon CA, Bronshtein V. Rabies vaccine preserved by vaporization is thermostable and immunogenic. Vaccine. 2015;33(19):2203–6. https://doi.org/10.1016/j.vaccine.2015.03.025.
Kadoya S, Fujii K, Izutsu K, Yonemochi E, Terada K, Yomota C, et al. Freeze-drying of proteins with glass-forming oligosaccharide-derived sugar alcohols. Int J Pharm. 2010;389(1-2):107–13. https://doi.org/10.1016/j.ijpharm.2010.01.027.
Acknowledgements
This work was supported by grants from the National Key Research and Development Program of China (No.2017YFD0500706-2) and The Key Research and Development Program of Jiangsu Province (BE2020336). The authors would like to extend their gratitude to Babalwa Nyide for proofreading and editing the manuscript.
Funding
This research was funded by The National Key Research and Development Program of China (No.2017YFD0500706-2) and The Key Research and Development Program of Jiangsu Province (BE2020336).
Author information
Authors and Affiliations
Contributions
Conceptualization, F.L.; Methodology, F.L., Y.L., A.O., and T.K.; Software, Y.Z., M.Z., and J.W.; Validation, X.Z.; Formal analysis, Y.L.; Investigation, Y.Z. and B.D.; Resources, M.Z.; Data curation, F.L., Y.Z., and X.Z.; Visualization, F.L.; Project administration, J.H.; Funding acquisition, J.H.; Supervision, A.O., J.H., and T.K. The authors have read and agreed to the published version of the manuscript.
Corresponding authors
Ethics declarations
Institutional Review Board Statement
Animals were maintained and euthanized as per the protocol, approved by the Institutional Animal Care and Use Committee (IACUC) of the Jiangsu Academy of Agriculture Sciences (IDSYXK 2020–0024). All experiments were conducted in accordance with the relevant guidelines and regulations of IACUC and the Institutional Biosafety Committee at the Jiangsu Academy of Agriculture Sciences.
Conflict of Interest
The authors declare that they have no known competing financial interest of personal relationships that could have a appeared to influence the work reported in this paper.
Additional information
Publisher’s Note
Springer Nature remains neutral with regard to jurisdictional claims in published maps and institutional affiliations.
Rights and permissions
Springer Nature or its licensor (e.g. a society or other partner) holds exclusive rights to this article under a publishing agreement with the author(s) or other rightsholder(s); author self-archiving of the accepted manuscript version of this article is solely governed by the terms of such publishing agreement and applicable law.
About this article
Cite this article
Lyu, F., Zhao, Yh., Lu, Y. et al. Vacuum Foam Drying Method Improved the Thermal Stability and Long-Term Shelf Life of a Live Attenuated Newcastle Disease Virus Vaccine. AAPS PharmSciTech 23, 291 (2022). https://doi.org/10.1208/s12249-022-02440-4
Received:
Accepted:
Published:
DOI: https://doi.org/10.1208/s12249-022-02440-4