Abstract
The current trend in erosion rate of ordinary chernozems in Saratov oblast has been assessed. Detailed studies have been performed in a small catchment area of the Bolshoi Kolyshley River (Medveditsa River basin) using the radiocesium and soil-morphological methods. Data of long-term hydrometeorological observations in the region, reported results of observations of water runoff and soil loss on the runoff plots of the South-Eastern Research Institute of Agriculture (Saratov oblast), results of analysis of the grain size and mineral composition of stratozems developed on the catchment valley bottom were also used for analysis. The quantification of sedimentation rate in the valley bottom during two time windows (1963–1986 and 1986–2017) based on the sediment dating using 137Cs as a chronomarker indicates their decrease at least in 4–6 times after 1986, which points to a proportional reduction in erosion rate on the plowed slopes of the catchment area. The analysis of the temporal variability of suspended yields in the regional rivers (Medveditsa and Khopyor) during the period from 1940 to the present time allows us to draw an analogous conclusion for the Volga Upland within Saratov oblast. The decrease in the erosion rate of ordinary chernozems could be mainly caused by the reduction in water runoff from slopes during the spring snowmelt because of reduction in soil freezing depth against the background of rising air temperature in winter and spring. This decrease in erosion rate during the snowmelt period was not balanced by the regional increase in rainfall erosion related to some increase in the rate of runoff-forming rainfalls. In the part of Saratov oblast, located within the Volga Upland, where the studied catchment is situated, on the contrary, even a reduction in the frequency of rains with a rainfall depth of more than 30 mm occurred over the last decades. The reduction in the cultivated land area since the late 1980s because of the abandonment of plowlands on the steepest slopes also contributed to the decrease in total soil loss by erosion at the river basin scale. Within the studied small catchment, the share of perennial grasses in crop rotations increased after 1986, which also led to reduction of the rate of erosion of ordinary chernozems.
Similar content being viewed by others
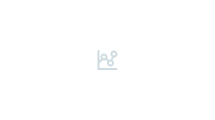
Explore related subjects
Discover the latest articles, news and stories from top researchers in related subjects.Avoid common mistakes on your manuscript.
INTRODUCTION
Saratov oblast is one of the most agriculturally developed administrative regions of European Russia. In 2015, it entered the top five regions of Russia with the largest cultivated area: 3 730 900 ha, or 4.7% of the total cultivated area in Russia [27]. The water erosion degradation of soil cover is one of the consequences of the high economic development of lands in Saratov oblast, although relatively slight, including because of the high erosion resistance of local soils. Thus, in 1995, the mean erosion rate in the region was estimated to be 1.8 t/(ha year), and the proportion of eroded lands was 10.8% [17], which were the lowest values for the Middle and Lower Volga River regions (except the lower and droughty Astrakhan oblast). A high degree of agricultural development is also typical for the Volga Upland in Saratov oblast, where the mean duration of the agricultural use of chernozems is about 200–250 years [49]. Long-term monitoring [21] showed that arable lands of the Volga Upland were subjected to relatively active erosion during the snowmelt period and the warm season until the 1990s. According to the erosion-prone map of European Russia [17], the soil loss rate from cultivated lands in the upland within the boundaries of Saratov oblast was estimated to be 1 to 10 t/(ha year) under the existing farming system. Lands in small river basins (the Volga River tributaries) with the maximum gradients of up to 15°–20° were most subjected to erosion (more than 3 t/(ha year)), and lands in the basin of the Medveditsa River (a left tributary of the Don River) with the maximum gradients of up to 7°–8° were the least eroded (up to 1–3 t/(ha year)) [21]. Analogous soil loss rates (1–3 t/(ha year)) were found in the Khopyor River basin, a transitional zone between the Volga Upland and the Oka–Don Lowland [17].
In the recent decades, appreciable hydroclimatic changes have been observed on the major part of European Russia [23, 30, 44, 46]. According to Frolova et al. [29], a significant degradation of floods as a stage of river water regime was caused by the rise in winter air temperatures and the increase in the amount and duration of thaw periods, which resulted in a reduction in the maximum snowmelt runoff in spring and a simultaneous increase in the share of groundwater runoff. The decrease in the intensity of floods in the Russian Plain was most significant in the Don River basin that occupies the major part of the Volga Upland in Saratov oblast. The share of water runoff during the floods decreased to 50% and less of the annual value, while about 60–70% of the annual runoff corresponded to the floods in the first half of the 20th century [29]. The noted hydrometeorological changes obviously affected the current rate of erosion in the region. The effect of these changes on erosion processes in basin geosystems was supplemented by measurements in land use: the reduction in plowland area was especially notable in the 1990s and early 2000s, primarily in the regions with low-efficient agriculture in the forest zones and the dry steppe subzone, as well as the change of crop rotations. In the steppe zone, the reduction area of cultivated lands was smaller than in the adjacent, more humid landscape zones, but it was still considerable: up to 27.5% on the average [39]. The dynamics of the cultivated land area and its structure in Saratov oblast is shown as an example in Table 1. According to calculations from erosion models [39], the total volumes of eroded soils in the steppe zone of the plain were reduced by 14% on the average.
The aim of this work was to assess the contemporary (since the 1950s) trend in erosion of arable soils in the steppe zone with the ordinary chernozem subtype as an example, based on the results of field studies on a typical small catchment area in the Medveditsa River basin located on the western megaslope of the Volga Upland (Saratov oblast), which were supplemented by hydrometeorological monitoring data.
CATCHMENT AREA AND ITS PHYSICOGEOGRAPHICAL CONDITIONS
The study site was the upper part of the “Srednyaya” dry valley catchment located on the left bank of the Bolshoi Kolyshley River valley (the river basin area is 651 km2; the river is a left tributary of the Medveditsa River) near the village of Varypaevka in the Atkarsk district of Saratov oblast (Fig. 1).
The geographical position of the studied small catchment of the “Srednyaya” dry valley (Atkarskii district, Saratov oblast, European Russia). Satellite image (WorldView-2, August 27, 2014): white (black) dashed lines denote the boundaries of the entire (studied) catchment area; (I, II, and III) soil (stratozem) profiles (sections) in the dry valley bottom; (V) the village of Varypaevka; (1) oblast centers; (2) administrative district centers of Saratov oblast; (3) Oktyabr’skii Gorodok weather station; (4) hydrological gauging stations on the Khopyor River (the city of Balashov and the village of Besplemyanovskii) and Medveditsa River (the village of Lysye Gory (LG)); (5) the catchment location.
Topography. The catchment has a drop shape in plan, which is typical for the region. The dry valley bottom is a zone of sedimentation and transit of soil washed out from slopes. The length of the studied part of dry valley together with the hollow in its heads is 1850 m (the total length from the source to the output on the Bolshoi Kolyshley River floodplain is 3030 m). The area of the studied part of the catchment is 0.78 km2, including its part (0.09 km2) separated by an embankment of an automobile (concrete-slab) road (presumably since the late 1960s) in the southwestern part. The relief of slopes, the longitudinal profiles of the hollow and the dry valley bottom, and the transverse profiles with sampling sites are shown in Fig. 2. The projection area of the “Srednyaya” dry valley is 0.069 km2, including the dry valley bottom area of 0.01 km2 with a mean gradient of 0.027 (0.019 in the heads of the studied area, 0.048 in its middle part, and 0.017 in the lower reaches). The mean absolute height of the studied part of the dry valley catchment is 235 m (height amplitude is 45 m); the mean gradient of its surface is about 2° (maximum of 4.4°). The catchment topography has a lithological (?) step in its central part and two hollows of 473 and 578 m in length, which concentrate the runoff in the left (with respect to the dry valley bottom) part of the catchment, as well as a small (150 m long) hollow in its right part.
Schematic maps of (a) the relief, (b) the spatial distribution of the A + ABca humus horizon thickness of ordinary chernozem, and (c) the integrated concentration of 137Cs in its upper 0- to 30-cm layer within the cultivated slopes of the small catchment of the “Srednyaya” dry valley (Atkarskii district, Saratov oblast): (1) positions of stratozem profiles/sections (I, II, and III) in the dry valley bottom; (2) sampling sites on slopes (1.1, 2.1, 2.2 … 4.7); (3) profile line; (4) dry valley and watercourse in its bottom; (5) automobile road embankment; (6) unstudied catchment part separated by the road; (7) presumed internal alluvial cone (zone of increased sediment accumulation) in the dry valley bottom; (L) horizontal distance; (h) absolute height.
Geological structure. The dry valley dissects Middle and Late Paleocene sediments (sand, silt, sandstone, clay, gaize, etc.) covered by Quaternary eluvial-deluvial loams and clays, which are parent rocks for the soils of the catchment slopes [3].
The climate of the region is moderately continental with hot summer and cold winter characterized by a steady snow cover. According to the data of the weather station in the town of Oktyabr’skii Gorodok of the Tatishchevskii district nearest to the catchment (about 23 km to the south-southeast), the mean annual temperature of atmospheric air was 5.1°C in 1950–2015 and showed a tendency of increase in the last decades (Table 2). Analysis of linear trends in total annual precipitation (from the Oktyabr’skii Gorodok weather station during the period of 1966–2016, 439 mm/year) for 1936–2012 showed a tendency of increase in all landscape zones of Saratov oblast [13]. The highest increase in annual precipitation was observed in the western region of the oblast, where the studied catchment is located. During the last 30–35 years, total annual precipitation mainly increased by 8–10%. The highest increase in monthly precipitation on the entire area was observed in September, January, and June; tendencies of decreasing precipitation were recorded for all stations in May and for most stations in August [13]. During the cold period (November–March), the amount of precipitation in the oblast generally increased, except for the forest-steppe zone. In the warm season (April–October), the amount of precipitation in most regions also increased, except the regions near the city of Saratov, where its decrease was noted. From the Oktyabr’skii Gorodok weather station data, a significant tendency toward a decrease in precipitation was noted in summer during the last decades, as well as its light increase during the other seasons, especially in spring (Table 3). The share of precipitation during the warm season (April–October) was about 67% in 1950–1986 and 63% in 1987–2016. In total for the oblast, the frequency of erosion hazard rainfalls (with a rainfall depth of more than 30 mm) increased during the last decades in 1.3 times compared to the period of 1912–1980 [16], while the Oktyabr’skii Gorodok weather station data indicated an opposite tendency, as will be shown below, which points to a high heterogeneity of precipitation distribution and its temporal variability in the semiarid landscapes of the Russian plain. Along with the rise in the winter and early-spring air temperatures (against the background of a slight increase in winter precipitation), a rise in soil temperature in the region was also noted in spring (March–April), which was most expressed at depths more than 0.2 m (Table 4).
Soils, vegetation, and landscapes. In landscape terms, the catchment and adjacent areas belong to the Idolga–Kolyshley region of the southern subzone, steppe zone, the Volga Upland [31]. The soils on the catchment are mainly clay and heavy loamy ordinary chernozems (Haplic Chernozems (Loamic, Pachic)) formed on the Late Pleistocene eluvial-deluvial deposits. Stratozems (Fluvisols) are developed on the dry valley bottom. The studied region is characterized by a slight general development of erosion processes proceeding on relatively gentle (1°–3°) slightly undulating watersheds [3]. Before the agricultural development of the region, the vegetation cover of the catchment consisted of rich forb-fescue-feather grass steppes [30]. At present, almost 89% of the catchment is tilled; its grassy zones (dry valley sides and bottom, space adjacent to the road) do not exceed 11% of the total area. At the time of fieldwork (third ten-day period of June 2017), the plowed ordinary chernozems of the studied catchment were sown with barleyFootnote 1. According to Ivanov et al. [12], the plowland area in the Medveditsa River basin (upstream of the town of Atkarsk, Saratov oblast), where the studied catchment is located, decreased from 68.5% in 1985 to 61.9% in 2015, while the forest and meadow areas increased from 13.7 to 16.5 and from 15.2 to 18.8%, respectively.
METHODS OF STUDY
The trend of changes in erosion–accumulation rates was estimated by the radiocaesium (137Cs) method, which is capable of reliably estimating the sedimentation rates in the different sediment sinks within the areas with bomb-derived and Chernobyl fallouts of 137Cs for different time windows [8, 40, 48]. The radioactive isotope 137Cs with a half-life of 30.2 years is widely used for assessing the present-day temporal dynamics of erosion and accumulation processes in basin geosystems [34, 38, 41, 43, 45, 47, 50, 51]. Several 137Cs fallout peaks are recorded within the major part of Europe (except the Pyrenean Peninsula): so-called bomb-derived (1958–1959 and 1962–1964) fallouts (with a maximum in 1963) related to the years of the most active open-air nuclear bomb tests in the atmosphere and distributed over the entire Northern hemisphere, and the Chernobyl fallout (1986) caused by the accident at the Chernobyl Nuclear Power Plant, whose distribution mainly includes Eastern, Central, and Northern Europe [2, 37]. All peaks fix the high altitudinal position of soil/sediment surface on the bottoms of first-order valleys and other undisturbed zones of stable sedimentation with a sufficiently good accuracy (±1 to 3 cm) at the time of 137Cs fallout in these years [5, 33]. The most active vertical migration of cesium occurs in the first years after the fallout of the isotope from the atmosphere and gradually decelerates with time in 1.5–2.0 times depending on the soil type, acidity, grain size distribution, infiltration features, etc. [36, 42]. If the mentioned 137Cs-marking layers persist in the stratum of accumulated sediments, the dating of sedimentation and the determination of its rate during three intervals (1959–1963, 1963–1986, and from 1986 to the soil sampling date) can be performed. The sedimentation rate of the soil particles eroded from the catchment slopes are directly and closely correlated with the dynamics of soil losses. However, the exact calculation of changes in erosion rate is feasible only for closed catchments (e.g., in the presence of a pond in the outlet) after the determination of the sedimentation rate and the areas of all accumulation zones existing or have existed within a specific catchment area [5].
Before the sampling of soil from the bottom of the studied dry valley “Srednyaya” for determining the specific activity (concentration) of 137Cs, the relief morphology of the dry valley catchment was thoroughly studied using a DJI Phantom 4 drone. The drone data were processed in Agisoft Photoscan, and a high-precision digital model of catchment relief was developed (Fig. 2). A detailed survey of the dry valley was also performed to study its morphology, including the analysis of bottom gradients and the identification of erosion cuttings, sediment accumulations in the bottom, transport paths of sediments from agricultural fields, etc.
From the results of surveys performed along the dry valley bottom, sites for three soil (stratozem) profiles of 64 (profile I), 30 (profile II), and 51 (profile III) cm in depth were selected to characterize areas with different morphological dynamics. The shallow depths of profiles II and III were related to the high groundwater level during fieldwork in June 2017. Soil horizons of each profile were described, and soil/sediment samples were taken layer-by-layer with intervals of 2, 3, and 5 cm from areas of 10 × 10 and 15 × 15 cm by manual tools, as well as with intervals of 10 cm at higher depths by a soil sampler (from 5 verticals in each profile to a depth of 100 cm), for the determination of 137Cs concentrations. Also, in all three profiles, samples were taken by a sampler with 5-cm intervals for the determination of grain size distribution in the accumulated sediments, as well as for the determination of mineral composition in profile I. On the cultivated slopes of the “Srednyaya” dry valley catchment, integrated samples of chernozemic soil were taken by a sampler at a depth of 0–30 cm in three transversal profiles in the different parts of the catchment also for the determination of 137Cs concentration. In the same sampling points, the thickness of the A + ABca humus horizon of ordinary chernozem was evaluated by the soil-morphological method. The coordinates of the sampling sites were determined using a high-accuracy GNSS receiver Trimble GeoExplorer 6000.
All samples were dried, disaggregated, and passed through a 2-mm sieve in the Makkaveyev Research Laboratory of Soil Erosion and Fluvial Processes, Faculty of Geography, Moscow State University (M.M. Ivanov, Principal Investigator). The specific activity of 137Cs was measured with an accuracy of 5–10% using an SKS-07(09) gamma-spectrometer (Green Star Instruments). Grain size distribution was determined on a Microtrac Bluewave S3500 analyzer (I.B. Vybornova, Principal Investigator) in the Research Laboratory of Ecological Innovations (Institute of Environmental Sciences, Kazan Federal University); the mineral composition of samples was determined with a Shimadzu XRD-700 diffractometer in the Lithological Laboratory of the Institute of Geology and Oil-and-Gas Technologies, Kazan Federal University.
Some meteorological parameters (air temperature, atmospheric precipitation, water storage in the snow, soil temperature at different depths) for 1950–2016 were also collected and processed using data of weather stations in the towns of Oktyabr’skii Gorodok and Atkarsk (freely available electronic resources of the All-Russia Research Institute of Hydrometeorological Information – World Data Center for Meteorology, VNIIGMI–MTsD) and observation results of water discharges and suspended sediment yield in the Medveditsa River (for 1940–2015) at the hydrological gauging station near the city of Lysye Gory (Saratov oblast), whose basin includes the studied small catchment of the “Srednyaya” dry valley, and in the Khopyor River (for 1940–2015), the river network of which drains the western part of the Volga Upland in Saratov oblast at the hydrological stations near the city of Balashov (Saratov oblast) and the village of Besplemyanovskii (Volgograd oblast). High-accuracy Landsat satellite images (image archive of the US Geological Survey) for the period from 1972 to 2014 were analyzed to reveal possible changes in plowland area and crop rotation within the studied catchment.
RESULTS OF STUDY
The 137Cs peaks of Chernobyl origin characterizing the position of soil/sediment surface in 1986 are located in the profiles of stratozems in the studied part of the dry valley either at the surface (profile I) or at a shallow depth (Fig. 3). The 137Cs peaks, characterizing the soil surface in 1963 and located at depths of 21–24 m, were detected only in profiles I and III, although they were partially eroded. In profile II, the 1963 peak cannot be identified; it apparently was degraded, because the profile is located on the part of dry valley bottom area with a relatively steep gradient at the crossing of the lithological (?) step. The presence of a small bench in the current longitudinal profile of the dry valley bottom on this plot indicates the formation of shallow secondary cuttings. The locations of the 1963 and 1986 137Cs-peaks in profiles I and III can be indicative of a significant decrease (at least by 4–5 times in terms of sediment thickness) in the accumulation rate of sediments on the dry valley bottom after 1986 compared to the period of 1963–1986. In profile I, sediments were not accumulated at all (in the result of their potential deposition and erosion) after 1986. In profile III, with consideration for the difference in the mean densities of sediment layers formed in different times, the accumulation rate was 7.5 kg/(m2 year) in 1963–1986 (mean density 962 kg/m3) and 1.17 kg/(m2 year) in 1986–2017 (726 kg/m3); i.e., it decreased by 6.4 times. The deep location of the presumable 137Cs 1959 peaks remained in profiles I and III compared to the 137Cs 1963 peaks indicates a higher (almost in 4 times) accumulation rate of sediments (soil material) washed away from arable slopes during the period of 1959–1963 compared to the period of 1963–1986. In other words, a stable general trend of decreasing accumulation rate of sediments (which formed stratozems in the dry valley bottom of the studied catchment) has been observed since at least the late 1950s. The depth of the accumulated sediments indicates that almost no soil loss could occur after 1986, because the layer of 3–5 cm thick deposits in profiles II and III could theoretically be accumulated during only a few erosion events.
Vertical distribution of 137Cs concentration in the stratozem profiles (I, II, and III) in the “Srednyaya” dry valley bottom (Atkarskii district, Saratov oblast): (h) sampling depth; mean sedimentation rates during the periods: r1, 1959–1963; r2, 1963–1986; r3, 1986–2017; (1) boundaries of sediment layers identified by their texture features; (2) sediment interlayers with small concentrations of 137Cs, if any. The concentrations of 137Cs in profiles at depths from 64–71 cm down to the maximum sampling depth are close or equal to zero; therefore, they are not shown on the diagrams.
The presence of sediment interlayers free from 137Cs in profiles I and II suggests that they could be formed during the accumulation of linear erosion products (now overgrown washouts?), which exposed almost cesium-free deluvial loamy deposits underlying chernozemic soils in the dry valley catchment (including dry valley sides?).
For the grain size distribution in sediments (stratozems) on the dry valley bottom exposed by cuttings, a general tendency is observed toward an increase in the share of the clay fraction and a decrease in the share of sand down the profile, which resulted in the general coarsening of mineral grains in the upper layers of profiles (Fig. 4). This can be partially related to soil-forming processes in the wetted valley bottom, which favor the vertical migration of the finest mineral particles. However, the retention of the 137Cs 1986 peak in profile I on the soil surface indicates that 137Cs migration was not very active. Otherwise, the 137Cs 1986 peak would shift to a depth of 3–5 cm, as it is observed, e.g., in Scandinavia [32]. The difference in grain size distribution was most marked in the topmost profile (I) of stratozem in the dry valley. The increased content of sand particles can be partially related to the intensive erosion event observed on the catchment during a downpour of 54 mm in 1985. In the case of significant rainfall runoff, coarser (sand) particles are primarily deposited in the zone of accumulation, because fine fractions move more actively during their transportation by the flow after the decomposition of soil aggregates [35]. Visual analysis of the vertical distribution of grain size parameters identified sediment series of different age in the stratozem layers (Fig. 4, Table 3), which are characterized by the specific landscape and climatic conditions of their accumulation. The relatively homogeneous mineral composition of stratozem in depth (Table 6) indicates that the sources of sediments incoming to the bottom did not change with time, and, hence, the construction of the automobile road had no effect on erosion processes, but it led to decreasing the maximum water runoff because of some reduction in the catchment area. It is important to note that the plowland area on the catchment has varied since 1960 only because of the construction of the road.
Vertical distributions of texture (G) and some its quantitative parameters ((D) mean diameter of mineral grains, μm; (Sk) asymmetry; (Ku) kurtosis), density (P, kg/m3) of sediments forming stratozems in the profiles I, II, and III in the “Srednyaya” dry valley catchment (Atkarskii district, Saratov oblast): (h) sampling depth; (g) sediment layer boundaries; texture: clay: (1) fine-grained (0.2–1 μm), (2) coarse-grained (1–5 μm); silt: (3) fine-grained (5–10 μm), (4) coarse-grained (10–50 μm); sand: (5) fine-grained (50–100 μm), (6) small-grained (100–250 μm), (7) medium-grained (250–500 μm), (8) coarse- and large-grained (500–2000 μm).
Analysis of the spatial distribution of the A + ABca horizon thickness of ordinary chernozem and the integrated concentration of 137Cs in its topmost 30-cm-thick layer within the dry valley catchment (Fig. 2) made it possible to identify plots differing in the rate of the agrogenic soil erosion. Thus, the best preservation of the humus horizon with a near-natural thickness is noted in the southwestern part of the catchment adjacent from the east to a motor road. This area is also characterized by the highest 137Cs concentration, which is typical for undisturbed or slightly eroded chernozem profiles, with an increased isotope concentration in its upper part. A relatively narrow belt of uneroded soils, although with some disruptions, is also detected along the watershed with the adjacent catchment in the east. Both plots are located in the catchment zones with natural or artificial limitations of surface runoff: in the first case, because of the presence of road embankment, and in the second case, because of the closeness to the watershed line, which decreases the natural water runoff concentration. Both plots represent a domain of the predominant destruction of soil cover by raindrops.
According to the thickness of the humus horizon of chernozem, the erosion rate on the resting cultivated slopes of the dry valley catchment is slightly higher. With consideration for the tillage of local soils for about 200–250 years [49] and the reduction in the thickness of the A + ABca horizon by about 10 cm, the erosion rate is estimated at about 4–5 t/(ha year). The distribution of cesium with depth in the catchment chernozems is relatively similar, which suggests a gradual decrease in the content of the radioisotope in the lower horizons. Therefore, the maximum current truncation (erosion denudation) of chernozem profile should be observed in the lower (northern) part of the catchment (the presumable thickness of the humus horizon is about 50 cm), where a low concentration of the isotope (<10 Bq/kg) is largely recorded. In the major (middle) part of the catchment, the content of 137Cs is higher (10–14 Bq/kg), which indicates, all other conditions being equal, a smaller loss of chernozem (the mean thickness of the humus A + ABca horizon is about 55–60 cm). However, it cannot be excluded that 137Cs of Chernobyl origin fell nonuniformly on the catchment surface, because its input from the atmosphere was caused by only a few rain events. Some increase in erosion rate in the northern direction is related to some topographic features of the catchment: there is general surface declivity to the north. Small areas of relative increase (point 3.2) and decrease (points 3.3 and 4.2) in soil loss on the transversal profiles are related to their local topographic features.
Soil loss in the southwestern part of the catchment located westward the motor road, which was laid in the late 1960s, had no appreciable effect on the contemporary (since the late 1950s) tendency toward a decrease in the accumulation rate of its products on the dry valley bottom. Therefore, no field studies were performed in this area.
DISCUSSION
The obtained results indicate a significant (at least 4- to 6-fold) decrease in the sedimentation rate on the valley bottom of the studied catchment in the last decades. Consequently, a proportional decrease in the erosion rate of ordinary chernozem (the material of which was the main source of these sediments) on the catchment may be supposed, with some degree of conventionality. Under the natural conditions of the catchment, the observed tendency in soil loss rate could be due to both climate change and changes in land use (land cover).
Climate change. The earlier observed positive long-term trend for air temperature in the region, especially in winter months, March and May, affected the formation of surface runoff and soil loss during the snowmelt period. From the data of weather stations in the towns of Oktyabr’skii Gorodok and Atkarsk (nearest to the catchment), a slight increase of water storage in the snow has occurred in March (just before the snowmelt period in the region) at least since the mid-1960s (Fig. 5). Unfortunately, reliable data (without multiyear gaps) were available only for the first ten-day period of March. However, the relatively high correlation of snow water storages between the ten-day periods of the same month and the same year indicates that the positive tendency of changes in snow water storage revealed in the first ten-day period remained throughout March. A rise in soil temperature was also noted in the region during the same period, which decreased the soil freezing depth at the end of the cold season.
Long-term variability of water storage in the snow (H) in three ten-day periods of March, 1966–2015, at the weather stations in (a) Oktyabr’skii Gorodok and (b) Atkarsk, Saratov oblast: (1) polynomial trend of the sixth order with its coefficient of approximation (R2); (r) linear correlation coefficient. Long-term average H value for the first ten-day period of March: 71.5 ± 18.5 mm in 1966–1986 and 74.4 ± 13.6 mm (∆ = +4.1%) in 1987–2015 at the Oktyabr’skii Gorodok weather station; 76.9 ± 20.0 mm and 88.7 ± 14.7 mm (∆ = +15.3%), respectively, at the Atkarsk weather station.
The temperature regime and freezing depth of soils significantly affect the development of erosion processes during snowmelt in spring. This conclusion agrees with the Barabanov’s rule [4] of limiting factors for surface snowmelt runoff, according to which runoff is not formed at a specific (limiting) value of a factor (freezing depth, air temperature, or snow water storage) regardless the values of other factors. When the freezing depth is higher than its critical value, the volume of spring runoff on arable slopes is determined by specific hydrometeorological conditions during the snowmelt period. Consequently, the decrease in soil freezing depth was the main climate change that reduced, through the redistribution mechanism of surface and groundwater runoff, the erosion and loss rates of chernozemic soils in the region, where the studied catchment is located, during the snowmelt period. This agrees with the results of observations on the runoff plots of the South-Eastern Research Institute of Agriculture (Saratov oblast): the annual surface runoff from the plowland and compacted fall-plowed land during the snowmelt was 10 and 34.5 mm, respectively, in 1973–1982 and decreased to 1.3 and 9.1 mm, respectively, to 2004–2014 [20]. This tendency was also traced for the long-term changes in water flow of the Medveditsa River, in the basin of which the analyzed catchment is located. Thus, a marked tendency of decreasing water runoff intensity was noted there during the snowmelt (flood) period, which resulted in a more than double decrease of the maximum water discharges of the river, as well as in a decrease in the share of flood runoff during the winter and summer low-water periods (Table 7). An increase in river water flow was simultaneously noted during the winter and summer low-water periods, which was related to the increase in the share of groundwater runoff during the periods of winter thaws and spring snowmeltFootnote 2. According to observations on the runoff plots of the South-Eastern Research Institute of Agriculture, the reduction in surface water runoff during the snowmelt period resulted in a decrease of soil loss rate by 6.2 times [20]. This largely agreed with the decrease in the accumulation rate of sediments on the bottom of the dry valley catchment “Srednyaya” revealed in our studies.
On the background of the mentioned changes in water runoff in the region, a distinct trend of decrease in the suspended sediment yield was noted, the dominant part of which was formed due to products of soil and gully erosion [9, 11]. From the dynamics of changes in sediment yield, the maximum erosion intensity in the Medveditsa River basin was observed in 1960–1970. The reasons for this phenomenon will be considered below. Analogous changes in water runoff were also observed in the adjacent Khopyor River basin located to the west (Table 8), which confirms their wide regional character.
The current hydrological-climatic decrease in the mean annual erosion rate of ordinary chernozems was partially related to the long-term changes in precipitation during the warm season and primarily the most erosion hazardous precipitation (with a rainfall depth more than 30 mm). The latter decreased not only in amount but also in specific intensity in the studied (western) part of the Volga Upland, according to observations of the nearest weather station in the town of Oktyabr’skii Gorodok (Fig. 6). A slightly different situation for the frequency of runoff-forming precipitation was observed on the eastern megaslope of the central Volga Upland, where a slight increase in the number of such rainfalls was noted at the simultaneous increase in soil loss rate [20]. However, the increase in rainfall erosion was mainly recorded on the runoff plots under fallow, while the share of fallows in crop rotation was no more than 10%. Moreover, the great majority of runoff-forming rains fall in summer, when the soil is to some degree protected by cropsFootnote 3. Consequently, despite the spatial variation of rainfall events, their contribution to total soil loss over the considered period was significantly lower.
Long-term variability of atmospheric precipitation in the warm season (April–October) with different rainfall depths (P) during the period of 1966–2016, according to the Oktyabr’skii Gorodok weather station data: (1) polynomial trend of the sixth order with its coefficient of approximation (R2); (K1 and K2) average shares of precipitation of the given rainfall depth in their mean annual amount in 1966–1986 and 1987–2016, respectively; (Pm) monthly precipitation ≥30 mm averaged over the corresponding period; (±∆) relative change in Pm between the periods. The distribution of precipitation according to the rainfall depths was as follows:
Rainfall depths, mm | Periods of observations, years | |||
1966–1986 | 1987–2016 | |||
P, mm/10 years | I, mm/event | P, mm/10 years (±∆, %) | I, mm/event (±∆, %) | |
<10 | 3532 | 1.5 | 3320 (–6.0) | 1.9 (+26.7) |
10–20 | 555 | 13.4 | 718 (+29.4) | 13.5 (+0.7) |
20–30 | 148 | 24.0 | 237 (+60.1) | 23.7 (–1.3) |
30–40 | 100 | 35.1 | 78 (–22.0) | 33.5 (–4.6) |
40–50 | Not observed | |||
>50 | 89 | 62.6 | 0 (–100.0) | 0.0 (–100.0) |
(I) average rainfall depth per event; (±∆) changes in parameter with respect to the period of 1966–1986. |
Changes in land use (land cover) pattern. The effect of economic activity on the decrease in soil erosion rate can be considered on the regional (basin) and local (catchment) scales. The abrupt increase of suspended sediment yield in the Medveditsa River basin, which was noted in 1963–1975, was not because of the anomalous water flow, which was minimum during this period. In the second half of the 1960s–the first half of the 1970s, a planned mechanization of agriculture occurred in the oblast, which became a significant factor for the intensification of agrarian production during this period and later on. From 1966 to 1974, the numbers of tractors and combines in the oblast increased by almost 24 and 33%, respectively [22]. Since the 1970s, modern tractors (T-4, T-50, T-53, T‑38M, etc.) have been received. The equipment of collective and state farms was supplemented with more efficient high-speed harvesters, modernized self-propelled combines SK-4, complexes of grain loaders, etc. Saratov oblast became a pioneer in the introduction of new powerful tractors K-700: 2456 Kirovets tractors were delivered to the region only in 1965–1970 [22]. These heavy tractors were used in almost all kinds of field works. Tractors K-700 performed more than 50% of agricultural transportations. In the result, the production of grain in local state farms was almost completely automatized to the early 1970s. All these processes most probably activated the agrogenic soil erosion on the fields of the region through changes in some physical properties of soils (primarily, overcompaction). This enhanced the total erosion and loss of soil, which increased the share of basin erosion products in rivers of the region. The effect of the mentioned anthropogenic changes is demonstrated in Fig. 7a, where the plot of correlation between the mean annual suspended sediment yield and the annual depth of water runoff in the Medveditsa River basin during the period until 1975 clearly distinguishes two scenarios (two curves) for the development of erosion in the basin: the years of relatively weak erosion (mainly during World War II and in postwar times): 1941, 1945, 1946, 1951, 1954, etc., and the years of relatively active erosion, mainly 1960–1970. Thus, the last period includes the years characterized by especially high (compared to the normal functioning described by a power correlation curve) values of sediment yield (erosion rate): 1973 (+124.8%), 1972 (+67.7%), 1970 (+40.3%), 1965 (+15.4%), 1964 (+11.5%), etc. The anthropogenic effect of river sediments on the runoff increase is indirectly confirmed by their specific parameter η (ratio between the specific sediment yield and the specific water discharge in a river basin), the value of which increased in the 1960s and early 1970s (Fig. 7c)Footnote 4. However, the history of interaction between the anthropogenic and hydrometeorological factors had peculiar features in every specific year of the period, e.g., the years of 1969 and 1973 with more or less close (small) values of water storage in the snow in early March. The year of 1969 was characterized by an appropriately low share of flood runoff and anomalously small amounts of soil erosion products in rivers, while in 1973, on the contrary, an anomalously strong soil erosion was noted in the Medveditsa River basin, as estimated from the sediment runoff values, at the middle share of flood runoff. In the latter case, it could also be partially related to the atmospheric precipitation of erosion hazard for chernozems (≥30 mm) in the warm season.
Temporal changes in the relationship between the mean annual suspended sediment yield (W) and the mean annual water runoff depth (h) of the (a) Medveditsa River near the city of Lysye Gory, Saratov oblast (F = 7160 km2) and (b) Khopyor River near the village of Besplemyanovskii, Volgograd oblast (F = 44 900 km2) in 1940–2015 for the years of relative (1) weakening and (2) activation of erosion in the river basins; R2 is the coefficient of approximation of the exponential trend line; 1940, 1941 … are the years of observation. (с) Ratios (η) of the mean annual specific suspended sediment yield (W) to the mean annual specific water discharge of the river (Q) [t/(km2 yr))/(L/(s km2)] averaged for the indicated periods (η(3)m, η(4)m, and η(5)m) for the hydrological gauging stations in (3) Lysye Gory (Medveditsa River), (4) Balashov (Khopyor River), and (5) Besplemyanovskii (Khopyor River), respectively; *for 1949–1954; ** data on 2009 are absent. On the plot (a), data on the Medveditsa River discharges in 1942, 1944, 1947, 1948, and 1955–1962 are absent; on the plot (b), data on the Khopyor River discharges in 1942–1945 and in 2009 are absent. Relatively low η values for the Khopyor River near the village of Besplemyanovskii (plot (c)) are partly caused by the large area of the river basins (three to six times larger than that of the Medveditsa River basin).
Similar increased values of suspended sediments were observed in the basin of Khopyor River, which drains the western part of Saratov oblast and the northwest of Volgograd oblast, upstream of the village of Besplemyanovskii in 1960–1970 (Figs. 1, 7b). However, in contrast to the Medveditsa River basin, the mean η-values in the 1960s–1970s underwent no radical changes with respect to the 1950s (Fig. 7c). This indicates that economic activity most probably plays a smaller role in the long-term dynamics of sediments in the Khopyor River basin upstream of the village of Besplemyanovskii. The annual suspended sediment yield in the Khopyor River also showed a marked tendency for a decrease in the recent decades; the mean values were as follows: 9.32 ± 2.43 kg/s in 1940–1941 and 1946–1966, 6.80 ± 2.48 kg/s in 1967–1975, and 1.79 ± 0.37 kg/s in 2008 and 2010–2015. The intensity of flood (snowmelt) runoff in the river basin almost halved: 1.1 mm/day in 1940–1966, 0.7 mm/day in 1967–1993, and 0.6 mm/day in 1994–2008 (no later data are available). The upward coarsening in the texture of sediments exposed in the profiles of stratozems on the dry valley bottom directly indicates a gradual decrease in snowmelt water runoff (its erosive role) since the 1940s–1970s. Under snowmelt erosion, soil aggregates are degraded almost on-site owing to soil thawing even before the impact of the slope runoff; therefore, finer fractions are transported in suspension at any runoff intensity. This trend toward a reduction in suspended sediment yield over the last decades completely agrees with the large-scale decrease of sedimentation rate on the dry valley bottom of the studied catchment.
Reduction in the areas of cultivated lands occurred in Saratov oblast in the last decades. As noted above, their area in the Medveditsa River basin (upstream of the town of Atkarsk) decreased in 2015 by almost 10% compared to 1985, primarily caused by the removal of the most erosion-hazardous slope segments from the plowland [12]. This was another reason for the current decrease in sediment material, including chernozem erosion products, in the river basin, although a relative activation of soil loss against the general decrease was observed in some years (the 2010s). The significantly lower values of sediment yield rate (η) in the rivers observed in 2008–2015 compared to 1950–1975 also indirectly indicate the leading role of the anthropogenic factor in this decrease.
As for the changes in land use (land cover) pattern within the studied small catchment, the following temporal dynamics was traced from the Landsat high-resolution images: different crops (predominantly spring varieties) were sown in the studied catchment during the period of 1972–2001, while in 2002–2014, the role of perennial grasses in this and adjacent small catchments had increased. According to observations on runoff plots [20], perennial grasses significantly prevent ordinary chernozems from loss; hence, they represent an additional factor of general current decrease in water erosion rate on slopes of this geosystem. Erosion control measures, which have been taken in the steppe zone since the late 1970s, and the ponds created in the river systems of the oblast could also contribute to the decrease in the weight of soil-rill-gully erosion products in the river suspended sediment yield. This information was not analyzed within the present work.
CONCLUSIONS
The quantitative estimation of changes in sediment accumulation rate in dry valley bottom of a typical small catchment in the central part of the Volga Upland in Saratov oblast, in combination with the dynamic analysis of water flow and suspended sediment yield in rivers draining the studied region, indicates a significant reduction in the erosive degradation of ordinary chernozem on plowlands in the last 30 years. The main reason for the revealed (at least 4–6-fold) decrease in soil loss rate on the plowlands was the abrupt decrease in surface water runoff from slopes during the spring snowmelt period. This decrease was due to the rise in air temperature in winter and spring months, which favored the reduction in soil freezing depth during the snowmelt period. The obtained results well agree with the data of monitoring observations over water runoff and sediment yields on the runoff plots located on the eastern megaslope of the Volga Upland. The revealed multidirectional changes in the frequency and intensity of runoff-forming rainfalls in the warm season played a secondary role and had no principal effect on the total reduction in soil loss rate in the region. The role of the anthropogenic factor in the decrease of the erosive degradation of chernozems within the studied catchment was manifested in an increase in the share of perennial grasses in the structure of crop rotations. In general, a reduction in the share of plowland occupied by perennial and annual grasses occurred in Saratov oblast during the last 25 years, partially owing to an increase in the area of sunflower plantations. An additional factor reducing the total soil loss by erosion on the regional scale was the reduction in the cultivated land area by 10% since 1985 because of the abandonment of the steepest plowland plots.
Tendencies toward a decrease in snowmelt water runoff, mean annual erosion rate, and sedimentation of erosion products were traced over the last decades almost throughout the forest-steppe zone, as well as in the northern and eastern parts of the steppe landscape zone in the East European Plain [1, 6–8, 10, 15, 19, 39]. They were most probably representative for the entire chernozemic zone of European Russia.
Notes
In 2012–2016, the crop structure in the Atkarskii district of Saratov oblast was as follows: cereal and pulse crops (wheat, oat, corn, etc.), 53%; technical crops and vegetables (sunflower, camelina, potato), 41%; forage crops, 5%; and other crops and fallows, 1% [24].
The increase in the low-water runoff in the river is hard to be largely explained by an increase in the amount of atmospheric precipitation in the river basin. For summer precipitation, its total decrease was even noted for the last 50 years.
From the satellite images of the area with the studied catchment, crops were gotten there in the early or middle August until the early 1990s. Before 1986, the average monthly precipitation ≥30 mm was maximum in this month and showed a tendency of the most pronounced decrease. This fact could favor an additional decrease in the soil loss rate (along with that in spring). Since the early 1990s, harvesting has already been operated in early September, when a significant increase in precipitation was observed. This fact, on the contrary, could slightly enhance soil erosion in fall.
Generally, high η values in the Medveditsa River compared to the Khopyor River indicate a higher agrogenic erosion of soils in the former river basin, although both basins occur under almost similar climatic conditions and the mean long-term annual water runoff depth in the Medveditsa River basin is even lower than in the Khopyor River basin. The large sediment yield in the Medveditsa River (12.6 t/(km2 year) in 1950–1975 and 4.3 t/(km2 year) in 2008–2015) is also favored by the predominance of better erodible loessial deposits of the Late Pliocene on the left part of the basin, while less erodible loamy–loamy sandy deposits of the Early Eopleistocene prevail on the right part of the Medveditsa River basin and in the Khopyor basin (6–8 t/(km2 year) in 1950–1975 and 1.3–1.8 t/(km2 year) in 2008–2015) [14].
REFERENCES
A. V. Apukhtin and M. V. Kumani, “Recent changes in the conditions of spring floods of rivers in the Kursk region,” Uch. Zap. Kursk. Gos. Univ., No. 1, 300–311 (2012).
Atlas of Radioactive Pollution of European Russia, Belarus, and Ukraine, Ed. by Yu. A. Izrael’ (Roskartografiya, Moscow, 1998) [in Russian].
Atlas of Saratov Oblast, Ed. by M. A. Shabanov (General Council of Geodesy and Cartography, Moscow, 1978) [in Russian].
A. T. Barabanov, Agricultural Forest Melioration in Soil Protective Farming (All-Russia Scientific Research Institute of Agroforest Reclamation, Volgograd, 1993) [in Russian].
V. N. Golosov, “A quantitative assessment of deposits' redistribution in the upper links of fluvial network: achievements and problems,” Geomorfologiya 3, 29–36 (2008).
V. N. Golosov, V. R. Belyaev, M. V. Markelov, and E. N. Shamshurina, “Specifics of sediment redistribution within a small arable catchment during different periods of its cultivation (Gracheva Loshchina catchment, Kursk oblast),” Geomorfologiya, No. 1, 25–35 (2012). doi 10.15356/0435-4281-2012-1-25-35
V. N. Golosov, A. N. Gennadiev, K. R. Olson, M. V. Markelov, A. P. Zhidkin, Yu. G. Chendev, and R. G. Kovach, “Spatial and temporal features of soil erosion in the forest-steppe zone of the East European Plain,” Eurasian Soil Sci. 44 (7), 794–801 (2011).
V. N. Golosov, N. N. Ivanova, A. V. Gusarov, and A. G. Sharifullin, “Assessment of the trend of degradation of arable soils on the basis of data on the rate of stratozem development obtained with the use of 137Cs as a chronomarker,” Eurasian Soil Sci. 50 (7), 1195–1208 (2017). doi 10.1134/S1064229317100039
A. V. Gusarov, “The main regularities of the ratio between riverbed and basin components of erosion and suspended sediment flux in the Northern Eurasia’s river basins,” Geomorfologiya, No. 4, 3–20 (2015). doi 10.15356/0435-4281-2015-4-3-20
A. V. Gusarov, V. N. Golosov, A. G. Sharifullin, and A. M. Gafurov, “Contemporary trend in erosion of arable southern chernozems (Haplic Chernozems Pachic) in the west of Orenburg oblast (Russia),” Eurasian Soil Sci. 51, 561–575 (2018). doi 10.1134/ S1064229318050046
A. P. Dedkov and V. I. Mozzherin, Erosion and Sediment Yield on the Earth (Kazan State Univ., Kazan, 1984) [in Russian].
M. A. Ivanov, A. V. Prishchepov, V. N. Golosov, R. R. Zalyaliev, K. V. Efimov, A. A. Kondrat’eva, A. D. Kinyashova, and Yu. K. Ionova, “Changes in cropland area in the river basins of the European part of Russia for the period 1985–2015 as a factor of soil erosion dynamics,” Sovrem. Probl. Distants. Zondir. Zemli Kosm. 14 (6), 149–157 (2017). doi 10.21046/2070-7401-2017-14-6-149-157
G. F. Ivanova and N. G. Levitskaya, “Changes in annual precipitation structure and soil water regime in the Saratov region,” Izv. Sarat. Univ., Ser. Nauki Zemle 15 (1), 11–15 (2015).
Map of Quaternary Formations in the Russian Federation, Scale 1 : 2500000, Ed. by O. V. Petrov, A. F. Morozov, et al. (Rosnedra, VSEGEI, VNIIOkeanologia, Moscow, 2010) [in Russian].
M. A. Komissarov and I. M. Gabbasova, “Snowmelt-induced soil erosion on gentle slopes in the southern Cis-Ural region,” Eurasian Soil Sci. 47, 598–607 (2014). doi 10.1134/S1064229314060039
N. G. Levitskaya, I. I. Demakina, and A. Yu. Verin, “Assessment of the dynamics of atmospheric precipitation in Saratov oblast,” in Proceedings of the All-Russia Scientific Conference Devoted to the 45th Anniversary of the All-Russia Research Institute of Arable Farming and Soil Erosion Control “Soil-Protective Farming in Russia,” Kursk, September 2015 (All-Russia Research Institute of Arable Farming and Soil Erosion Control, Kursk, 2015), pp. 184–188.
L. F. Litvin, Geography of Soil Erosion of Agricultural Lands in Russia (Akademkniga, Moscow, 2002) [in Russian].
L. F. Litvin, Z. P. Kiryukhina, S. F. Krasnov, and N. G. Dobrovol’skaya, “Dynamics of agricultural soil erosion in European Russia,” Eurasian Soil Sci. 50 (11), 1344–1353 (2017). doi 10.1134/S1064229317110084
M. V. Markelov, V. N. Golosov, and V. R. Belyaev, “Changes in the sedimentation rates on the floodplains of small rivers in the Central Russian Plain,” Vestn. Mosk. Univ., Ser. 5: Geogr., No. 5, 70–76 (2012).
I. F. Medvedev, N. G. Levitskaya, V. Z. Makarov, and V. A. Nazarov, “The results of monitoring of erosion processes on chernozems in the Volga region,” Agrar. Nauch. Zh., No. 8, 29–34 (2016).
I. F. Medvedev and A. I. Shabaev, “Erosion of arable land on the Volga Upland,” Pochvovedenie, No. 11, 61–69 (1991).
E. Yu. Necheporuk, “Mechanization of agriculture in Saratov oblast during the intensification of agricultural production: 1965–1975,” Vestn. Chelyab. Gos. Univ., No. 6 (297), 82–86 (2013).
V. V. Popova and I. A. Polyakova, “Change of stable snow cover destruction dates in Northern Eurasia, 1936–2008: impact of global warming and the role of large-scale atmospheric circulation,” Ice and Snow, No. 53 (2), 29–39 (2013). doi 10.15356/2076-6734-2013-2-29-39
Planted Area of Agricultural Crops, Russian Federal State Statistics Service. https://fedstat.ru/. Accessed December 12, 2017. [in Russian].
Planted Area in the Soviet Union: Statistical Bulletin (State Statistical Publ., Moscow, 1957), Vol. 1. [in Russian].
Planted Area in the Soviet Union: Statistical Bulletin (State Statistical Publ., Moscow, 1957), Vol. 2. [in Russian].
Agriculture in Saratov blast, AB-Tsentr. http://ab-centre.ru/page/selskoe-hozyaystvo-saratovskoy-oblasti/. Accessed November 15, 2017.
Agriculture in the Soviet Union: Statistical Bulletin (Finansy i Statistika, Moscow, 1988) [in Russian].
N. L. Frolova, M. B. Kireeva, S. A. Agafonova, V. M. Evstigneev, N. A. Efremova, and E. S. Povalishnikova, “Lowland rivers runoff within-year distribution and changes on the European territory of Russia,” Vodn. Khoz. Ross.: Probl., Tekhnol., Uprav., No. 4, 4–20 (2015).
A. B. Shmakin and V. V. Popova, “Dynamics of climate extremes in Northern Eurasia in the late 20th century,” Izv., Atmos. Ocean. Phys. 42, 138–147 (2006).
Ecological Resource Atlas of Saratov Oblast, Ed. by V. S. Belova and G. I. Khudyakova (Roskartografiya, Saratov, 1996) [in Russian].
S. Almgren and M. Isaksson, “Vertical migration studies of 137Cs from nuclear weapons fallout and the Chernobyl accident,” J. Environ. Radioact. 91, 90–102 (2006). doi 10.1016/j.jenvrad.2006.08.008
P. G. Appleby, “Three decades of dating recent sediments by fallout radionuclides: a review,” Holocene 18, 83–93 (2008). doi 10.1177/0959683607085598
M. Benmansour, L. Mabit, A. Nouira, R. Moussadek, H. Bouksirate, M. Duchemin, and A. Benkdad, “Assessment of soil erosion and deposition rates in a Moroccan agricultural field using fallout 137Cs and 210Pbex,” J. Environ. Radioact. 115, 97–106 (2013). doi 10.1016/j.jenvrad.2012.07.013
L. Beuselinck, A. Steegen, G. Govers, J. Nachtergaele, I. Takken, and J. Poesen, “Characteristics of sediment deposits formed by intense rainfall events in small catchments in the Belgian Loam Belt,” Geomorphology 32, 69–82 (2000). doi 10.1016/S0169-555X(99)00068-9
E. A. Buraeva, O. S. Bezuglova, V. V. Stasov, V. S. Nefedov, E. V. Dergacheva, A. A. Goncharenko, S. V. Martynenko, L. Y. Goncharova, S. N. Gorbov, V. S. Malyshevsky, and T. V. Varduny, “Features of 137Cs distribution and dynamics in the main soils of the steppe zone in the southern European Russia,” Geoderma 259–260, 259–270 (2015). doi 10.1016/j.geoderma.2015.06.014
M. De Cort, G. Dubois, Sh. D. Fridman, M. G. Germenchuk, Yu. A. Izrael, A. Janssens, A. R. Jones, G. N. Kelly, E. V. Kvansikova, I. I. Matveenko, I. M. Nazarov, Yu. M. Pokumeiko, V. A. Sitak, E. D. Stukin, L. Ya. Tabachny, et al., The Atlas of Cesium Deposition on Europe after the Chernobyl Accident (European Communities, Luxembourg, 1998).
V. N. Golosov, V. R. Belyaev, and M. V. Markelov, “Application of Chernobyl-derived 137Cs fallout for sediment redistribution studies: lessons from European Russia,” Hydrol. Process. 27, 781–794 (2013). doi 10.1002/hyp.9470
V. Golosov, A. Gusarov, L. Litvin, O. Yermolaev, N. Chizhikova, G. Safina, and Z. Kiryukhina, “Evaluation of soil erosion rates in the southern half of the Russian Plain: methodology and initial results,” Proc. Int. Assoc. Hydrol. Sci. 375, 23–27 (2017). doi 10.5194/piahs-375-23-2017
V. N. Golosov, D. E. Walling, A. V. Konoplev, M. M. Ivanov, and A. G. Sharifullin, “Application of bomb- and Chernobyl-derived radiocaesium for reconstructing changes in erosion rates and sediment fluxes from croplands in areas of European Russia with different levels of Chernobyl fallout,” J. Environ. Radioact. 186, 78–89 (2018). doi 10.1016/j.jenvrad.2017.06.022
J. Jweda and M. Baskaran, “Interconnected riverine-lacustrine systems as sedimentary repositories: case study in southeast Michigan using 210Pb and 137Cs-based sediment accumulation and mixing models,” J. Great Lakes Res. 37 (3), 432–446 (2011). doi 10.1016/j.jglr.2011.04.010
G. Kirchner, F. Strebl, P. Bossew, S. Ehlken, and M. H. Gerzabek, “Vertical migration of radionuclides in undisturbed grassland soils,” J. Environ. Radioact. 100, 716–720 (2009). doi 10.1016/j.jenvrad.2008.10.010
L. Mabit, M. Benmansour, and D. E. Walling, “Comparative advantages and limitations of the fallout radionuclides 137Cs, 210Pbex and 7Be for assessing soil erosion and sedimentation,” J. Environ. Radioact. 99, 1799–1807 (2008). doi 10.1016/j.jenvrad.2008.08.009
H. Madsen, D. Lawrence, M. Lang, M. Martinkova, and T. R. Kjeldsen, “Review of trend analysis and climate change projections of extreme precipitation and floods in Europe,” J. Hydrol. 519, 3634–3650 (2014). doi 10.1016/j.jhydrol.2014.11.003
P. N. Owens, D. E. Walling, J. Shanahan, and I. D. L. Foster, “The use of caesium-137 measurements to establish a sediment budget for the Start catchment, Devon, UK,” Hydrol. Sci. J. 42, 405–423 (1997). doi 10.1080/02626669709492037
H. Park, A. B. Sherstiukov, A. N. Fedorov, I. V. Polyakov, and J. E. Walsh, “An observation-based assessment of the influences of air temperature and snow depth on soil temperature in Russia,” Environ. Res. Lett. 9, 064026 (2014). doi 10.1088/1748-9326/9/6/064026
P. Porto, D. E. Walling, C. Alewell, G. Callegari, L. Mabit, N. Mallimo, K. Meusburger, and M. Zehringer, “Use of a 137Cs re-sampling technique to investigate temporal changes in soil erosion and sediment mobilization for a small forested catchment in Southern Italy,” J. Environ. Radioact. 138, 137–148 (2014). doi 10.1016/j.jenvrad.2014.08.007
P. Porto, D. E. Walling, C. La Spada, and C. Callegari, “Validating the use of 137Cs measurements to derive the slope component of the sediment budget of a small rangeland catchment in Southern Italy,” Land Degrad. Dev. 27, 798–810 (2016). doi 10.1002/ldr.2388
A. Y. Sidorchuk and V. N. Golosov, “Erosion and sedimentation on the Russian Plain, II: The history of erosion and sedimentation during the period of intensive agriculture,” Hydrol. Process. 17, 3347–3358 (2003). doi 10.1002/hyp.1391
D. E. Walling, A. L. Collins, P. A. Jones, G. J. L. Leeks, and G. Old, “Establishing fine-grained sediment budgets for the Pang and Lambourn LOCAR catchments, UK,” J. Hydrol. 330, 126–141 (2006). doi 10.1016/ j.jhydrol.2006.04.015
F. Zapata, Handbook for the Assessment of Soil Erosion and Sedimentation Using Environmental Radionuclides (Kluwer Acad. Publishers, Dordrecht, 2002).
ACKNOWLEDGMENTS
We thank A.Z. Satdarov, A.M. Gafurov, and R.A. Medvedeva (PhD students, Kazan Federal University) for assistance in summer fieldwork in 2017.
The work was supported by the Russian Science Foundation, project 15-17-20006.
Author information
Authors and Affiliations
Corresponding author
Additional information
Translated by K. Pankratova
Rights and permissions
About this article
Cite this article
Gusarov, A.V., Sharifullin, A.G. & Golosov, V.N. Contemporary Trend in Erosion of Arable Ordinary Chernozems (Haplic Chernozems (Pachic)) within the Volga Upland (Saratov Oblast, Russia). Eurasian Soil Sc. 51, 1514–1532 (2018). https://doi.org/10.1134/S1064229318120049
Received:
Published:
Issue Date:
DOI: https://doi.org/10.1134/S1064229318120049