Abstract
Groundwater is the world’s primary source of fresh water. However, groundwater resources in the basement complex region, such as Nigeria, have been overstressed due to excessive abstraction, and the rate of groundwater replenishment is declining as a result of climate change. Thus, this study investigated the influence of climate change on groundwater recharge scenarios. Twenty years of data on climatic records were collected from the Nigeria Meteorological Agency of Nigeria (NIMET), Abuja. The data was sorted into annual and average records and were inserted into the empirical formulas to obtain the groundwater recharge, surface runoff, evapotranspiration, and recharge coefficient. The influence of the climatic change on the groundwater variables was investigated using the multiple regression analysis (MANOVA) models of the Paleontological Statistics 4.13 software and a model was developed. The descriptive statistics of the data revealed the highest maximum temperature (33.3 °C) in 2019, minimum temperature (22.5 °C) in 2005, relative humidity (88.63%) in 2008, sunshine (5.85 h) in 2011, wind (4.69 knots) in 2016, Evapotranspiration (1310.28 mm) in 2008 and annual rainfall (1692 mm) in 2010. The lowest climatic variables (max temp (30.8 °C), min temp (20.4 °C), relative humidity (69.63%) sunshine (5.18 h), wind (3.14 knot), annual rainfall (1014.7) and evapotranspiration (1204.14) were reported in 2005, 2020, 2011, 2011, 2001, 2001 and 2012, respectively. The corresponding highest values of groundwater recharge and direct runoff from rainfall are 248.75 mm and 907.39 mm, respectively in 2010 while the highest value of recharge coefficient was 23.92 in 2006. The multivariate multiple regression analysis separated the variables into dependent variables (groundwater recharge, direct runoff, and rainfall coefficient) and independent variables (Min and max temperature, humidity, sunshine, wind, annual rainfall, and evapotranspiration). The Wilks lambda value, P (regression), and F-static are 0.0006994, 6.979E-11, and 16.1, respectively for the overall MANOVA. The tests on independent variables show varying and high Wilki Lambda, P-values, and low F-static; (0.9081, 0.7989, 0.3372 for humidity, 0.701, 0.31,1.361 for sunshine, 0.8603, 0.6647, 0.5414 for wind, 0.8363, 0.5995, 0.6523 for min temp, 0.879, 0.717, 0.459 for max temp, 0.9266, 0.8498, 0.264 for evapotranspiration). The Wilki’s Lambda, P-value, and F-static for annual rainfall is (0.001775, 4.76E-14, 1875. The R2 value for Groundwater recharge and direct runoff are 0.99 and 0.91, respectively while that of the recharge coefficient is 0.32. Collectively, there is a significant difference between the groundwater recharge and the climatic factors, and the model developed is significant. However, the individual influence of the climatic variables on groundwater resources is less significant, thus the only climatic factor contributing significantly to the groundwater recharge in the study area is rainfall. The percentage of rainfall contributing to the groundwater recharge is (15.63%) and this is very low for any resource sustainability. The groundwater recharge pattern in the study area has not changed reasonably but rainfall contribution has increased by 1%. Conservative measures and sustainable management practices should be put in place for future management of the resource.
Similar content being viewed by others
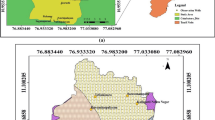
Avoid common mistakes on your manuscript.
1 Introduction
The management and sustainability of groundwater resources are influenced by factors such as climate, climate change, groundwater interactions with surface water, geology, and topography among others. The climate is the behavior of the atmosphere over a long period while climate change is the change in the long-term averages of daily weather conditions such as rainfall temperature, sunshine, humidity, wind, etc. Several definitions have been given to climate change: Climate change is global warming and has been attributed to changes in weather parameters over decades of years (Aribisala et al. 2015). Onoja et al. (2011) attributed climate change to natural and human causes such as biotic processes, variations in solar radiation received by the earth, plate tectonics, volcanic eruptions, and burning of fossil fuels.
Climate change has been stated as one of the major problems increasing the mean temperature of some regions and its disturbance in the occurrence and severity of some hydrological events such as flood and drought is alarming (Patel and Mehta 2023). Groundwater resources have been vital in the sustenance of drinking water, irrigation, agricultural and industrial maintenance and it has been reported that it meets above one-third of global drinking water demands (Swain et al. 2022). The demand for groundwater in the world has intensified in the last decades and the pressure may increase in the future (Reinecke et al. 2021; Addisie 2022). The monitoring of the effect of climate change is crucial for sustainable groundwater resources management. The information on the impact of climate change and its variables on groundwater is very limited and the challenges are rising with a more worsen situation predicted for the future (Kumar 2012; Ashaolu 2016; Guptha et al. 2022). The effect of climate change on groundwater resources is twofold; direct and indirect impacts. The direct impact of climate change relates to the natural recharge mechanisms from rainfall while the indirect impacts are those concerned with the changes in groundwater use, governed by anthropogenic activities (Swain et al. 2022).
The measurement of the natural groundwater recharge and discharge is vital for effective groundwater resource management. The fraction of the total precipitation falling on the earth that infiltrates and reaches the water table is the natural groundwater recharge. The availability of enough water in the ground is governed by geology and the soil but rainfall and evapotranspiration are governed by the climate. The variability in climate change has been reported to come with two extremes, flood, and drought and it has been reported to have a pronounced impact on groundwater. The ozone layer in the atmosphere is already depleted and it has been stated that Nigeria is currently experiencing the catastrophic effects of climate change in the area of flooding; the year 2022 flooding has been described as the worst Nigeria has experienced since 2012 (Daily Trust 2022).
Heavy rainfall events contribute to groundwater resources but the recharge in some boreholes of tropical Africa is disproportionate to extreme rainfall events (Owor et al. 2009; Taylor et al. 2013). Groundwater residence time ranges from days to thousands of years thus, measuring the responses to climate change is delayed and this could be challenging (Aizebeckhai 2011). One of the measuring parameters for future management and sustainability of groundwater is recharge; rainfall being the major contributor to recharge describes the quantity of water that will enter any aquifer. Quantifying the quantity of water that recharges the ground is challenging because groundwater recharge is influenced by several factors such as infiltration, evaporation, runoff, temperatures, and land cover etc. with precipitation being the major driving force, (MacDonald et al. 2021; Ashaolu 2018). There are high uncertainties associated with the regional and global climate models regarding the effects of climate change on rainfall patterns (Bates et al. 2008). Any increase in intensity and frequency of heavy rainfall events interspersed with severe and prolonged droughts may induce changes in recharge and discharge of groundwater systems.
Climate change affects groundwater recharge rate, depth to the water table, and water levels in Aquifers, and most simulated models for climate change predicted a more than 70% decrease in recharge for southwestern Africa by 2050 (Aizebeckhai 2011). The portioning of recharge, runoff, and evapotranspiration is controlled by the depth of groundwater and the lateral redistribution of water in the ground. (Condon et al. 2020). A warmer climate increases evapotranspiration and this may affect the portion of precipitation that infiltrates the ground as recharge or the fraction that becomes runoff (Condon et al. 2020). Climatic factors such as temperature gradients, wind, and relative humidity control potential evapotranspiration. Any temperature increase can reduce groundwater storage; at 15°C warming, groundwater storage may decrease by 100,000 MCM (Condon et al. 2020). Climate change can increase runoff if the storm is intense and subsequent extreme flooding events. The assessment of the impact of climate change on groundwater resources as reported by Aribisala et al. (2015) concluded that climate change did not have a pronounced effect on groundwater resources.
Climate change influenced the rainfall characteristics with a corresponding impact on groundwater recharge (Makinde et al. 2017; Zakwan 2021). An increase in the rainfall amount as a result of climate swing influenced the groundwater resources in the basement complex of Nigeria (Ashaolu 2016). Climate change scenarios on groundwater recharge will not change by the year 2030 if it is estimated using all weather parameters but the temperature rise will bring a corresponding decrease in groundwater recharge (Kambale et al. 2016). Groundwater systems are expected to be affected by recharging changes, including changes in precipitation and evapotranspiration and potential environmental changes in connections between groundwater and groundwater systems (Deshmukh et al. 2022). The rainfall that contributes to groundwater aquifer is the recharge coefficient (Alloca et al. 2014). The increasing hazards of climate change are real, numerous, and alarming (Deshmukh et al. 2022) and the annual groundwater recharge and storage are projected to increase from the baseline for all scenarios of future climate (Soundala and Saraphirom 2022). Several studies on the influence of climate change on groundwater resources have been conducted globally; Aribisala et al. (2015) used the climate trend on the borehole trend to check the groundwater recharge, Makinde et al. (2017) used the time series model to check if the climatic variations affect the aquifer in Southwestern, Nigeria, Ashaolu (2016), used the non-parametric Sen’s slope estimator for the slope of the linear trend of the climatic factors and groundwater recharge, Kambale et al. (2016) used ARIMA and HYDRUS ID to climate factors scenerios; Soundala and Saraphirom (2022) used numerical model to simulate the impact of climate change on groundwater resources.
The groundwater resources in the basement complex of Nigeria have been reported to be overstressed due to over abstraction and the major source of recharge is solely from direct rainfall (Ashaolu 2016). Fewer studies look at recharge in the context of the prevailing climatic swing, hence, management and sustainability of the resources become difficult. The study aims at evaluating the influence of climatic change on groundwater resources and the specific objectives are to monitor the changes in the climatic trend, obtain annual rainfall and estimate the groundwater recharge, evapotranspiration and the direct runoff, the climatic variables influencing their values and the percentage of rainfall returned as recharge.
2 Materials and Methods
2.1 Study Area
The study area (Fig. 1) is the Osogbo metropolis located on latitudes N 7044′0″- N 7048′0″ and longitude E 4o32′0″- E 4° 36′0″. The area is underlined by weathered gneisses typical of the basement complex of Nigeria (Oyelami et al. 2022). The vital rock groups are migmatite complex and metasediments such as; quartzite, schist, and amphibolite. The soils in and around the area are relatively deep, and rich, primarily derived from coarse and granite rock, and have greater proportions of ferruginos tropical red soils known as laterites (Adegboyega et al. 2018). It is within the lowland tropical rainforest vegetation; it is properly drained by river Osun and it has a land mass of 66,264 km2 (Alimi et al. 2022; David and Nwaogozie 2023).
2.2 Methods
The study collected 20 years (2001–2020) of data on daily rainfall, wind speed, sunshine hours, humidity, and maximum and, minimum temperature from Nigeria Meteorological Agency, (NIMET) Abuja. The climatic data were extracted and sorted on a monthly and yearly basis and were subjected to descriptive statistics. The results were inputted into the empirical to estimate the subjected to descriptive statistics. The results were inputted into the empirical formulas to estimate the groundwater recharge, recharge coefficient, direct runoff, and evapotranspiration. The climatic factors and groundwater recharge were subjected to regression analysis to obtain the trend line with time and the model equations were generated.
The relationship that existed between the climatic factors (independent variables) and the groundwater variables (dependent variables) was extracted by using the multiple regression analysis (MANOVA) models of the Paleontological Statistics 4.13 software. A model was developed for the groundwater recharge and the direct runoff. The estimation of evapotranspiration was done using the FAO Penman–Monteith model (Eq. 1) of the CROPWAT software version 8.0. Groundwater Recharge was estimated using the Modified Chaturvedi equation (Eq. 2), effective rainfall (ER) was obtained from the USDA S.C method from the CROPWAT software, recharge coefficient was estimated using (Eq. 3), and direct runoff was estimated using the water budget approach (Eq. 4).
Estimation of evapotranspiration
where:
To is the estimated evapotranspiration [mm/day].
\({R}_{n}\)= the surface radiation balance [MJ/m2/day].
G = sensible heat flux in the soil [MJ/m2/day].
T = average atmospheric temperature [°C].
\({U}_{2}\)= wind speed at 2 m height [m/s].
\({e}_{s}\) = saturation vapor pressure [kPa].
\({e}_{a}\)= actual vapor pressure [kPa].
Δ = slope of the water vapor saturation pressure curve [kPa/°C].
Ɣ = psychrometric constant [kPa/°C].
Estimation of Groundwater recharge
where AP = annual rainfall in inches.
Estimation of Recharge coefficient
where:
GRr = Groundwater recharge.
ER = effective rainfall.
Estimation of Direct runoff
3 Results and Discussion
3.1 Descriptive Statistics of Climatic Data
The descriptive statistics of the data and estimated values of groundwater variables (Table 1) revealed the highest climatic factors: maximum temperature (33.3 °C) in 2019, minimum temperature (22.5 °C) in 2005, relative humidity (88.63%) in 2008, sunshine (5.85 h) in 2011, wind (4.69 knots) in 2016, Evapotranspiration (1310.28 mm) in 2008 and annual rainfall (1692 mm) in 2010. The lowest climatic variables (max temp (30.8 °C), min temp (20.4 °C), relative humidity (69.63%) sunshine (5.18 h), wind (3.14 knot), annual rainfall (1014.7) and evapotranspiration (1204.14) were reported in 2005, 2020, 2011, 2011, 2001, 2001 and 2012, respectively. There was a gradual but fluctuating increase in maximum temperature until it rose to the peak in 2019 (Fig. 2) and it is the highest reported in the study area since year 1980 (Ashaolu 2016; Babatola and Asaniyan 2016). Although the maximum temperature is high, studies from similar basement complex has reported a maximum temperature of 37.3 °C (Obiefuna and Orazulike 2010). The values of humidity fluctuate, but the highest humidity reported in 2008 in this study did not agree with Ashaolu (2016). Coincidentally, the highest humidity occurred in the year with the highest number of rainy days (Table 1) while the year 2015 reported the lowest rainy days (98 days).
The maximum annual rainfall obtained in this study is similar to the annual rainfall amount (1692 mm) obtained by Oke et al. (2015) but the difference in the averages of maximum rainfall when compared to Ashaolu (2016) is an indication that the annual rainfall amounts have increased over the years (Babatola and Asaniyan 2016; Oke et al. 2015; Ashaolu 2016). This is corroborated with the findings of Ogunbode and Ifabiyi, (2019) that more rainfall will be experienced in the tropics as a result of changes in temperature. The estimated groundwater variables (Table 1) reported the highest (248.75 mm) and lowest (174.67 mm) groundwater recharge in 2001 and 2019, respectively. A plot (Fig. 3) of the recharge against other variables shows that the evapotranspiration is very high and did not fluctuate evenly with the annual rainfall: evapotranspiration in the year 2001, 2011, 2015 and 2017 were higher than the annual rainfall and it clearly shows why the graph of groundwater recharge is far below evapotranspiration, annual rainfall and direct runoff (Fig. 3). The climatic factors have not changed significantly and this observation agrees with some literature that the south-western region of Nigeria has generally not experienced a pronounced change in climate except for rainfall which has witnessed about a 2% to 5% increment (Aribisala et al. 2015). The average amount (216 mm) of water entering the groundwater as recharge is 15.63%, of the annual rainfall thus the fraction of rainfall returning as groundwater has not changed when compared to past studies. Several factors are responsible for the pattern of groundwater recharge aside from climatic factors, rainfall, runoff, and evapotranspiration; land use, geology, natural vegetation, and infiltration capacity among others are also very critical factors of study. From Fig. 3, the portion of rainfall returning as direct runoff is equally high, thus a larger percentage of annual rainfall is returning as direct runoff and evapotranspiration which implies that the groundwater recharge mechanism of the study area may be controlled beyond the factors of climate.
3.2 Trend Analysis
The trend plot (Fig. 4) generated the statistics presented in Table 2. The Akaike ICc (AIC) for the minimum temp (9.843), Sunshine (8.0673) Max temp (10.404), and Wind (10.337) are moderately high while Humidity (243.84) Eto (10,483), AR (7.293), GRr (8526.7) are very high. Moderately high AIC suggests that the model is an average fit but it’s not the best fit. The R2 value for the maximum temperature is higher (0.4) than for other climatic variables thus 40% of the variance in the maximum temperature is explained by the independent variable but the model may not be significant in predicting the maximum temperature. Other than for maximum temperature the R2 value for other climatic factors is very low hence there is no relationship between the predictor and the response variable. The sunshine, wind, groundwater recharge, and annual rainfall are negatively sloped (Fig. 4) which may suggest a negative correlation. The climatic variables are changing with time but the changes are not significant.
3.3 Effect of Climate Change on Groundwater Recharge
The result of the multivariate multiple regression analysis (Table 3) separated all the variables into dependent variables (groundwater recharge, direct runoff, and recharge coefficient) and independent variables (the climatic factors Min and max temperatures, humidity, sunshine, wind, annual rainfall, and evapotranspiration) using Eq. 5. The Wilks lambda value for the Overall MANOVA was between 0 and 1 and this shows how well the function separated the variables into groups. The low value of Wilki’s lambda (0.0006944) is an indication that there is a significant difference between the dependent variables and the climatic factors. The F- value (16.1) is high suggesting a significant difference between the groups and the P- value (6.979E-11) is quite low, thus there is an indication that the model is very significant and the variables have an effect on each other. The groundwater recharge pattern has not changed when compared to Ashaolu (2016). However, the tests on independent variables show that the climatic factors have less influence on the groundwater variables when considered individually.
The Wilki Lambda and the P-values are high, and the F-static is low; (0.9081, 0.7989, 0.3372 for humidity, 0.701, 0.31,1.361 for sunshine, 0.8603, 0.6647, 0.5414 for wind, 0.8363, 0.5995, 0.6523 for min temp, 0.879, 0.717, 0.459 for max temp, 0.9266, 0.8498, 0.264 for evapotranspiration) The Wilki’s Lambda, P-value and F-static (0.001775, 4.76E-14, 1875 for annual rainfall (Table 3) shows a statically significant variable with all the dependent variables. The high significance of annual rainfall and groundwater recharge agrees with the findings of Ashaolu (2016) and Makinde et al. (2017) for a typical basement complex. As reported by Ashaolu (2016), rainfall and wind is very significant in their contribution to groundwater recharge but the result of this study shows that the only variable of significance is the annual rainfall and this implies that the recharge mechanism pattern has changed.
The tests on the dependent variables (Table 3) show that 99% and 91% of the variance in the dependent variable (Groundwater recharge, direct runoff) is defined and explained by the climatic variables. The F-value and P- P-value of the groundwater recharge and direct runoff shows that the model is significant and it is a well-fitting regression (Eqs. 5 and 6).
where:
\(b_{1} ,b_{2} \ldots b_{n} = {\text{the coefficient for each predictor variables }}\left( {\text{climatic variables}} \right)\)
4 Conclusion and Recommendation
The climatic factors in the study area fluctuated but the increase is not significant. The significant change in temperature was reported in 2019 and the highest rainfall (1692.3) was reported in 2010. The highest groundwater recharge, direct runoff and evapotranspiration are 248.75 mm 907.39 mm and 1310.28 mm respectively and the only climatic factor influencing their values is the rainfall. The evapotranspiration and the direct runoff values are very high and only 15.63% of annual rainfall is returned as recharge. The sustainability of groundwater resource in the area is not guaranteed if it is used exclusively for all water uses. A further study that will consider all the factors affecting groundwater recharge is crucial and efforts to reduce the direct runoff and evapotranspiration should be adopted.
References
Addisie MB (2022) Groundwater recharge estimation using water table fluctuation and empirical methods. H2Open J 5(3):457–468. https://doi.org/10.2166/h2oj.2022.026
Adegboyega SA, Onuoha OC, Adesuji KA, Olajuyigbe AE, Olufemi AA, Ibitoye MO (2018) An intergrated approach to modelling of flood hazards in the rapidly growing city of Osogbo, Osun State, Nigeria. Space Sci Int 4:1–15
Aizebeckhai AP (2011) Potential impacts of climate and variability on groundwater resources in Nigeria. Afr J Environ Sci Technol 5(2):760–768
Alimi SA, Andongma TW, Ogungbade O, Senbore SS, Alepa VC, Akinlabi OJ, Olawale LO, Muhammed QO (2022) Flood vulnerable zones mapping using geospatial techniques: a case study of Osogbo metropolis, Nigeria. Egypt J Remote Sens Space Sci 25:841–850
Allocca V, Manna F, De Vita P (2014) Estimating annual groundwater recharge coefficient for karst aquifers of the Southern Apennines (Italy). Hydrol Earth Syst Sci 18:803–817. https://doi.org/10.5194/hess-18-803-2014
Aribisala JO, Awopetu MS, Ademilua OI, Okunade EA, Adebayo WO (2015) Effect of climate change on groundwater resources in southwest Nigeria. J Environ Earth Sci 5(12):2223
Ashaolu ED (2016) Influence of climate on groundwater recharge clues for sustainable groundwater development in basement complex aquifers of Osogbo, Nigeria. In: Tilakasin SL (ed) Water land people in climate change stamford lake Pannipitiya, Srilanka, pp 109–128
Ashaolu ED (2018) Effect of climate and landuse/land cover change on groundwater recharge in osun drainage basin, Nigeria. Unpublished MSc thesis of the Department of Geography, University of Ilorin
Babatola EB, Asaniyan BR (2016) Relative comparison of resultant effects of global warming on variability of rainfall and water resources in Saki and Osogbo Western Nigeria. J Prog Res Soc Sci 4(1):262–270
Bates BC, Kundzewics ZW, Wu S, Palutikof JP (2008) Climate change and water. IPCC Secretariat, Technical paper of the intergovernmental panel on climate change technical paper VI p 210
Condon LE, Atchley A, Maxwell R (2020) Evapotranspiration deplets groundwater under warming over the contiguous United States. Nat Commun 11:873
Dailytrust (2022) https: //dailytrust.com sun 30th October 2022
David AO, Nwaogozie II (2023) Excel optimization solver model development for Osogbo’s rainfall intensity duration and frequency. FUW Trends in Sci Technol J 8(1):19–24
Deshmukh MM, Elbeltagi A, Kouadri S (2022) Climate change impact on groundwater resources in Semi-arid regions. Resources. https://doi.org/10.1007/978-3-031-04707-7_2
Guptha GC, Swain S, Al-Ansari N, Taloor A, Dayal D (2022) Assessing the role of SuDS in resilience enhancement of urban drainage system: a case study of Gurugram City India. Urban Clim 41:101075. https://doi.org/10.1016/j.uclim.2021.101075
Kambale JB, Singh DK, Sarangi A (2016) Impact of climate change on groundwater recharge in a Semi-Arid region of Northern India. Appl Ecol Environ Res 15(1):335–362. https://doi.org/10.15666/aeer/1501_335362
Kumar CP (2012) Climate change and its impact on groundwater resources. Int J Eng Sci 1(5):43–60
MacDonald AM, Lark RM, Taylor RG, Abiye T, Fallas HC, Favreau G, Goni IB, Kebede S, Scanlon B, Sorensen JP, Tijani M (2021) Mapping groundwater recharge in Africa from ground observations and implications for water security. Environ Res Lett 16:034012. https://doi.org/10.1088/1745-9326/abd66
Makinde V, Adeleke OO, Eruola AO, Okeyode IC, Akinboro FG, Alatise OO, Dada OF (2017) Impact of climate change on groundwater resources in Odeda local government area, Ogun-state, Southwest Nigeria. Afr Rev Phys 12:1–13
Obiefuna GI, Orazulike DM (2010) Application and comparison of groundwater recharge estimation methods for the semiarid Yola area, Northeast Nigeria. Glob J Geol Sci 9:177–204
Ogunbode TO, Ifabiyi PI (2019) Rainfall trends and its implications on water resources management: a case study of Ogbomoso city in Nigeria. Int J Hydrol 3(3):210–214
Oke MO, Martins O, Idowu OA, Aiyelokun O (2015) Comparative analysis of groundwater recharge estimation value obtained using empirical methods in Ogun and Osun river basin. IFE J Sci 17(1):056–063
Onoja US, Dibua UM, Enete AA (2011) Climate change: causes effects and mitigation of a review. Global J Pure Appl Sci 17(4):469–479
Owor M, Taylor RG, Tindimugaya C, Mwesigwa D (2009) Rainfall intensity and groundwater recharge empirical evidence from the upper Nile Basin. Environ Res Lett 4:1–12. https://doi.org/10.1088/1748-9326/4/3/035009
Oyelami CA, Akandeb W, Kolawole TO (2022) A preliminary geotechnical assessment of residual tropical soils around Osogbo metropolis as materials for road Subgrade. J Niger Soc Phys Sci 4:157–164
Patel S, Mehta D (2023) Statistical analysis of climate change over Hanumangarh district. J Water Clim Change 6:2029. https://doi.org/10.2166/wcc.2023.22
Reinecke R, Müller Schmied H, Trautmann T, Andersen LS, Burek P, Flörke M, Gosling SN, Grillakis M, Hanasaki N, Koutroulis A, Pokhrel Y (2021) Uncertainty of simulated groundwater at different global warming levels: a global scale multi-model ensemble study. Hydrol Earth Syst Sci 25:787–810
Soundala P, Saraphirom P (2022) Impact of climate change on groundwater recharge and salinity distribution in the Vientiane Basin, Lao PDR. J Water Clim Change 13(11):3812–3829. https://doi.org/10.2166/wcc.2022.161
Swain S, Taloor AK, Dhal L, Sahoo S, Al-Ansari N (2022) Impact of climate change on groundwater hydrology: a comprehensive review and current status of the Indian hydrogeology. Appl Water Sci 12(120):1–25
Taylor RG, Doell P, Scalon BR, Rodell M (2013) Ground water and climate change. Natl Clim Change 3:322–329
Zakwan M (2021) Trend analysis of groundwater level using innovative trend analysis. In: Pande C, Moharir KN (eds) Groundwater resources development and planning in the Semi-Arid Region. Springer International Publishing, Berlin, pp 389–405
Author information
Authors and Affiliations
Contributions
OOF: Conceptualization, Experimental design and development, Data curation, Investigation, Original draft, Review and Editing. MAK: Original draft, Preparation of figures and Tables, Review and Editing. KI: Review and Editing.
Corresponding author
Ethics declarations
Conflict of interests
The authors declare no competing interests.
Rights and permissions
Springer Nature or its licensor (e.g. a society or other partner) holds exclusive rights to this article under a publishing agreement with the author(s) or other rightsholder(s); author self-archiving of the accepted manuscript version of this article is solely governed by the terms of such publishing agreement and applicable law.
About this article
Cite this article
Fadipe, O.O., Kareem, M.A. & Ishola, K. Influence of Climate Change on Groundwater Resources in Osogbo, Osun State, Nigeria. Iran J Sci Technol Trans Civ Eng (2024). https://doi.org/10.1007/s40996-024-01389-7
Received:
Accepted:
Published:
DOI: https://doi.org/10.1007/s40996-024-01389-7