Abstract
Increasing sugar content is the primary objective of sugarcane (Saccharum officinarum L.) improvement programs. To assess the importance of sucrose metabolizing enzymes in relation to sucrose content, enzymatic profiles of sucrose phosphate synthase (SPS), sucrose synthase (SuSy), acid and neutral invertases (AI and NI) were analyzed in relation to total sugars and reducing sugars in different sugarcane genotypes at 300, 360 and 420 days after planting (DAP). Varying responses in enzymatic profiles in relation to sugar content were noticed. The activities of SPS exceeded that of SuSy, AI and NI at 360 DAP, while SuSy was dominant at 420 DAP. Total sugars content were highest at 360 DAP and then declined at 420 DAP. Reducing sugars increased gradually from 300 to 420 DAP. Significant negative and positive correlations of SPS with reducing sugars and total sugars, respectively were noticed at 360 DAP. Interestingly, NI was strongly associated with reducing sugars, total sugars and ratio of total sugars to reducing sugars at 360 DAP as compared to AI. Differences between SPS–AI and SPS–NI exhibited significant negative correlation with reducing sugars and positive correlation with total sugars at 360 DAP. Differences between SPS–NI explained most of the variations found for sucrose accumulation in sugarcane. Our data demonstrate that synthesis and breakdown of sugars in sugarcane tissue is coordinated by the activities of AI, NI and SPS, respectively.
Similar content being viewed by others
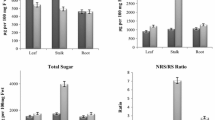
Explore related subjects
Discover the latest articles, news and stories from top researchers in related subjects.Avoid common mistakes on your manuscript.
Introduction
The quality of sugarcane (Saccharum officinarum L.) is primarily determined by its sugar content at harvest. For this, the better understanding of the role of sucrose metabolizing enzymes in relation to sugar content during maturation period in different sugarcane genotypes is required. There is an increasing demand for sugar in the global as well as domestic market, and this demand can be met only by increasing the cane productivity and stored sucrose concentration in sugarcane (Ebrahim et al. 1998). Although, sugar beet substantially contributes in world sugar production, but countries like India are heavily dependent on sugarcane. Sugar recovery in mills depends on the amount of stored sugar in cane tissue (Joshi et al. 2013). The mature stalk tissue of sugarcane comprised of high concentrations of disaccharide sucrose and lower amounts of its hydrolysis product reducing sugars (glucose and fructose), which is balanced by the breakdown and synthesis reactions (Botha and Black 2000; Rohwer and Botha 2001).
Sucrose metabolism is governed by sucrose synthesizing enzymes, such as sucrose synthase, sucrose phosphate synthase, and hydrolyzing enzymes, viz., acid and neutral invertases (Bosch et al. 2004; Mao et al. 2005). While SPS produces sucrose, SuSy can either cleave sucrose to UDP-glucose and fructose or catalyze the reverse synthetic reaction (Siswoyo et al. 2007). Depending on the cellular location and pH optima, invertases (acid invertase—AI and neutral invertase—NI) cleave sucrose into glucose and fructose (Ji et al. 2005; Ansari et al. 2013). Zhu et al. (1997) reported that accumulation of sucrose in sugarcane tissue is mainly due to differences between SAI and SPS activities. On the other hand, several reports have positively correlated sucrose concentration in sugarcane internodes with SuSy and SPS activities and negatively with AI and NI activities (Gutierrez-Miceli et al. 2002; Siswoyo et al. 2007). In recent years, some efforts have been made to engineer sugarcane for the enhanced sugar production via manipulation of genes associated with sucrose content (Conradie 2011).The maturation phase is of high importance since it determines the sugar level at maturity and in turn price to farmers. As sugarcane provides up to ~60 % of the world sugar supplies (Grivet and Arruda 2001), besides significant quantity of the world’s transport fuel is sourced from agricultural crops, including sugarcane, the pressure to supply enough sugar for food will continue (Glassop et al. 2007).
Therefore, the aim of the present study was to investigate the sucrose accumulation pattern, the activities of different sucrose metabolizing enzymes and the relationship of sugar contents and enzyme activities at different stages of development in different sugarcane genotypes.
Materials and methods
Plant material
Eight mid-late maturing genotypes of sugarcane, viz., CoC 671, Co 8014, CoM 9904, CoM 9908, CoM 9909, Co 94012, Co 95012 and Co 86032 were procured from nursery of Malegaon SSK factory, Malegaon, Maharashtra and Vasantdada Sugar Institute, Pune, Maharashtra, India. The experimental work was carried out at 300, 360 and 420 days after planting (DAP), (10th, 12th and 14th month) at the laboratory of Plant Physiology, Vasantdada Sugar Institute, Pune, Maharashtra, India. Stalks from each genotype were randomly selected and harvested in three replicates. The 6th internode (index internode) from top from each replicate was cut, labeled and immediately kept in liquid nitrogen and brought to the laboratory. The samples were crushed to a fine powder in pre-cooled mortar and pestle and immediately used for enzyme assays. The enzyme assays were performed in a cold room at 4 °C. The samples for sugar extraction were stored at −20 °C.
Extraction and estimation of sugars
Both total and reducing sugars were extracted from frozen 1 g stalk tissue. Total sugars were further extracted and determined by using Anthrone reagent method (Hedge and Hofreiter 1962), while reducing sugars were extracted and estimated by using DNSA (dinitro salicylic acid) reagent method (Miller 1959).
Protein extraction
Total protein was extracted while performing the enzyme assays and measured according to Lowry et al. (1951) method. Protein concentrations were determined using bovine serum albumin as standard.
Extraction and assay of enzymes
Enzymes activities of SuSy, SPS, AI and NI were determined from 6th internode. Two g of inter-nodal tissue was homogenized separately in 0.1 M Tris buffer (pH 7.5) containing 1 mM EDTA, 1 mM MgCl2, 1 mM phenyl methane sulfonylfluoride (PMSF), 1 mM DTT (dithiothreitol), 1 % polyvinylpyrrolidone (PVP) and 1 g sea sand. The homogenate was filtered through muslin cloth and centrifuged at 18,000 rpm for 20 min at 4 °C (Remi high speed refrigerated centrifuge, R-244A, India), and the supernatant was used for enzyme assays.
Assay of sucrose synthase (SuSy)
Sucrose synthase activity was assayed at 35 °C by the method of Hubbard et al. (1989) with slight modifications. The reaction mixture contained 100 µl enzyme, 50 mM Tris–HCl (pH 7.5), 1.25 mM MgCl2, 1.25 mM uridine diphosphate glucose (UDPG), 1.25 mM fructose and 1.0 mM Na2Mo7. The mixture was incubated for 30 min at 35 °C and the reaction was terminated with the addition of 2 N NaOH, followed by addition of 5 ml of resorcinol, and the tubes were kept at 100 °C for 10 min. The blank and control tubes contained distilled water instead of fructose. After cooling absorbance was measured at 480 nm using UV–visible spectrophotometer (Shimadzu UV 1600 Double Beam Spectrophotometer).
Assay of sucrose phosphate synthase (SPS)
The procedure followed for the assay of sucrose phosphate synthase was similar to that of sucrose synthase, except that fructose 6-phosphate was used instead of fructose in the assay mixture. Activities of SuSy and SPS are expressed as amount of enzyme required to synthesize mg of sucrose mg−1 of protein h−1.
Assay of acid invertases (AI)
AI activity was assayed using modified method of Vorster and Botha (1999). The assay medium consisted of 1 M Acetate buffer (pH 5.4), 250 μl 4 % sucrose, 500 μl enzyme and 150 μl distilled water. The blank and control tubes contained distilled water instead of glucose. The assay mixture was incubated at 37 °C for 60 min. After the reaction mixture was neutralized with 1.5 % NaOH, DNSA reagent was added to the above tubes and heated at 100 °C for 10 min in a boiling water bath. After cooling, absorbance was measured at 540 nm using UV–visible spectrophotometer (Shimadzu UV 1600 Double Beam Spectrophotometer). The enzyme activity was measured as mg of glucose mg−1 protein h−1.
Assay of neutral invertase (NI)
The procedure followed for neutral invertase assay was similar to that of AI, except that in the assay mixture instead of acetate buffer 1 M phosphate buffer (pH 7.0) was used. Assay mixture consisted of 1 M M phosphate buffer (pH 7.0), 250 μl 4 % sucrose, 500 μl enzyme and 150 µl distilled water. The activities of acid and neutral invertases are expressed as amount of enzyme required to produce mg of glucose mg−1 of protein h−1 at 37 °C.
Statistical analysis
Data was statistically analyzed by ANOVA using SigmaPlot Version 11.0, Germany. The values were expressed as the mean ± standard deviation of three replications. Correlation between enzyme activity and sucrose content was analyzed using Pearson correlation coefficient (r) at significance levels p < 0.001, p < 0.01 and p < 0.05.
Results and discussion
Sugar content
Significant differences were observed for total sugars at 300, 360 and 420 DAP. Total sugars were low at 300 DAP (ranged from 10.71 to 14.81 %) and then increased at 360 DAP (ranged from 13.40 to 16.27 %) and then declined at 420 DAP (ranged from 9.49 to 14.74 %) (Fig. 1A). On the other hand, the concentration of reducing sugars increased gradually from 300 to 420 DAP (Fig. 1B). As the plant mature, the invertase activity increases with the concurrent decrease in sucrose content and increase in reducing sugars (Tana et al. 2014). The variability in sugar content in different sugarcane cultivars during different growth phases (270–390 DAP) has also been reported by Joshi et al. (2013) and Tana et al. (2014). It has been reported that sucrose is the predominant sugar at maturity (360 DAP) and increased from 6 to 12 months (180–360 DAP) (Joshi et al. 2013; Tana et al. 2014).
Genotypes CoC 671 (16.27 %), Co 86032 (16.00 %) and Co 95012 (15.80 %) accumulated highest sugar content at 360 DAP (Fig. 1A, B) and therefore, these genotypes may be selected as high sucrose storing genotypes. Considering the importance of the length of period of potential sucrose accumulation in determining final sugar content, the extension of this period is a warranted strategy for improving cane quality. This may be accomplished by using two approaches. First, the available natural genetic variability in the sugarcane species for sucrose content, and the second strategy is to extend the length of the period from the loss of AI until harvest by delaying the ripening process, either genetically or by agrochemical means.
The high ratio of total sugars to reducing sugars at 360 DAP indicate better sink strength of CoC 671 (8.88) and Co 86032 (8.59) (Fig. 1C), which is in agreement with Sachdeva et al. (2003), who reported that decrease in AI activity resulted in increased ratio of sucrose to reducing sugars.
Sucrose synthase (SySy) and sucrose phosphate synthase (SPS) activities
Significant differences were observed for SuSy and SPS activities at 300, 360 and 420 DAP (Fig. 2A, B). Except in case of Co 86032, the activity of SuSy remained high at 420 DAP as compared to rest of the genotypes, and the genotype CoC 671 exhibited highest SuSy activity (7.39 units) at 420 DAP as compared to rest of the genotypes (Fig. 2A). It has been reported that the activity of sucrose synthase during maturation can either increase or decrease (Botha and Black 2000; Joshi et al. 2013). Peculiarly, SPS activity was also higher in CoC 671 (3.84 units at 360 DAP), followed by Co 86032 (3.44 units), however, highest activity was observed in Co 95012 (3.60 units at 420 DAP) (Fig. 2B). The variability in different sucrose metabolizing enzymes in sugarcane cultivars during different growth stages has been reported by Tana et al. (2014). SPS is the key enzyme in metabolic compartment of storage tissues, catalyzing the re-synthesis of sucrose during sucrose accumulation cycle (Sachdeva et al. 2003). The high activity of SPS with concomitant increase in sugars at 360 DAP in inter-nodal tissue of some genotypes (Fig. 2B) may be attributed to the higher sink strength, i.e., sucrose storing capacity of those genotypes. These results suggest that SPS can be used as a selection marker for high sucrose accumulating genotypes at maturation phase.
Acid and neural invertase activities
AI and NI activities in inter-nodal tissue were substantially lower than SuSy and SPS activities in all the genotypes at 300, 360 and 420 DAP (Fig. 2C, D), which indicate the co-ordinated roles in sugar accumulation during maturation phase (at 360 DAP). Both AI and NI activities were almost similar in all the genotypes at 360 DAP, while at 420 DAP some variability was noticed. AI activity was higher than NI at 420 DAP. Highest AI activity was observed in CoC 671 (0.69 units), followed by CoC 9908 (0.49 units), while genotype CoC 9904 (0.31 units) exhibited highest NI activity at 420 DAP as compared to rest of the genotype (Fig. 2C). The increase in activities of AI and NI at 420 DAP have also been reported by Sachdeva et al. (2003) and Joshi et al. (2013). Decrease in total sugars at 420 DAP with concomitant increase in SuSy, AI and NI activities (Figs. 1, 2) implies the synchronized role of SuSy, AI and NI in sucrose degradation after maturation. The increase in SPS and total sugars with concomitant decrease in AI, NI and reducing sugars during maturation phase can be useful in selecting the genotypes with sucrose (non-reducing sugar content) at 360 DAP.
The cleavage activity of SuSy exceeded that of hydrolytic activities of AI and NI suggesting that sucrose could be degraded in the cytosol by SuSy prior to its transportation into the vacuole.
Relationship of sucrose metabolizing enzymes with sugar content
The correlations of sucrose metabolizing enzymes with sugar content are depicted in Table 1. At 360 DAP, SPS exhibited significant negative correlation (p < 0.05; r = −0.69) with reducing sugars and a strong significant positive correlation with total sugars (p < 0.05; r = 0.80) and ratio of total sugars to reducing sugars (p < 0.001; r = 0.93) (Table 1). SuSy did not show any correlation with sugars at any growth stage, which is probably due to the fact that SPS was dominant in synthesis activity of sugars at maturity. Botha and Black (2000) also reported lack of correlation between SuSy activity and sucrose accumulation. Similarly, no relationship was observed between the activities of SPS and SuSy at maturity of early and midlate maturing sugarcane genotypes (Batta et al. 2008).
Both AI and NI were positively correlated with reducing sugars (r = 0.66 and r = 0.79) and negatively correlated with total sugars (r = −0.52 and p < 0.05; r = −0.76) and ratio of total sugars to reducing sugars (p < 0.05; r = −0.72 and p < 0.001; r = −0.94) at 360 DAP. These results are is in agreement with Botha and Black (2000) and Ma et al. (2000) who reported that a negative correlation of AI is important in the accumulation of sucrose. Negative correlation between AI and NI with sugars has also been reported by Siswoyo et al. (2007), Pan et al. (2009) and Tana et al. (2014). NI was found to be more associated with reducing sugars and total sugars at 360 DAP suggesting that NI was one of the possible key enzymes having impact on sucrose accumulation (Rohwer and Botha 2001).
The difference between SPS–AI showed negative correlation with reducing sugars (p < 0.05; r = −0.70) and strong positive correlation with total sugars (p < 0.01; r = 0.80) and ratio of total sugars to reducing sugars (p < 0.001; r = 0.93) at 360 DAP (Table 1), which is in agreement with Gutierrez-Miceli et al. (2002). Our results showed greater positive correlation between SPS–NI with total sugars and ratio of total sugars to reducing sugars (Table 1). These results clearly indicated that NI exerts slightly greater influence on sucrose accumulation as compared to SPS and AI. These findings suggest that, after ripening, decrease in SPS and total sugars is accompanied by an increase in hydrolytic activities of invertases.
In view of the above results it can be concluded that understanding of variability and correlation between enzymatic profiles with sugar content at different growth stages, especially during maturation phase will be an important step for genotype selection for appropriate harvesting time. The manipulation of specific sucrose metabolizing enzyme using molecular or agrochemical techniques may be a powerful tool for enhanced sugar production in sugarcane.
References
Ansari, M. I., Yadav, M., & Lal, R. (2013). An-overview on invertase in sugarcane. Bioinformation, 9(9), 464–465.
Batta, S. K., Pant, N. C., Thind, K. S., & Uppal, S. K. (2008). Sucrose accumulation and expression of enzyme activities in early and mid-late maturing sugarcane genotypes. Sugar Tech, 10, 319–326.
Bosch, S., Grof, C. P. L., & Botha, F. C. (2004). Expression of neutral invertase in sugarcane. Plant Science, 166, 1125–1133.
Botha, F. C., & Black, K. G. (2000). Sucrose phosphate synthase and sucrose synthase activity during maturation of internodal tissue in sugarcane. Australian Journal of Plant Physiology, 27(1), 81–85.
Conradie, T. T. (2011). Genetic engineering of sugarcane for increased sucrose and consumer acceptance. Ph.D. thesis University of Stellenbosch.
Ebrahim, M. K., Zingsheim, O., El-Shourbagy, M. N., Moore, P. H., & Komor, E. (1998). Growth and sugar storage in sugarcane grown at temperatures below and above optimum. Journal of Plant Physiology, 153, 593–602.
Glassop, D., Roessner, U., Bacic, A., & Bonnett, G. D. (2007). Changes in the sugarcane metabolome with stem development. Are they related to sucrose accumulation? Plant Cell Physiology, 48(4), 573–584.
Grivet, L., & Arruda, P. (2001). Sugarcane genomics: depicting the complex genome of an important tropical crop. Current Opinion in Plant Biology, 5, 122–127.
Gutierrez-Miceli, F. A., Rodríguez-Mendiola, M. A., Ochoa-Alejo, N., Méndez-Salas, R., Arias-Castro, C., & Dendooven, L. (2002). Relationship between sucrose accumulation and activities of sucrose phosphate synthase, sucrose synthase, neutral invertase and soluble acid invertase in micropropagated sugarcane plants. Acta Physiologiae Plantarum, 24, 441–446.
Hedge, J. E., & Hofreiter, B. T. (1962). Carbohydrate chemistry. New York: Academic Press.
Hubbard, N. L., Huber, S. C., & Pharr, D. M. (1989). Sucrose phosphate synthase and acid invertase as determinants of sucrose concentration in developing muskmelon (Cucumis melo L.) fruits. Plant Physiology, 91, 1527–1536.
Ji, X., Van den Ende, W., Van Laere, A., Cheng, S., & Bennett, J. (2005). Structure evolution and expression of the two invertase gene families of rice. Journal of Molecular Evolution, 60, 615–634.
Joshi, B. J., Krishnaveni, S., Vijayalakshmi, D., Sudhagar, R., & Raveendran, M. (2013). Activities of enzymes involved in synthesis and degradation of sucrose in popular sugarcane varieties. Asian Journal of Experimental Biology, 4(2), 237–244.
Lowry, O. H., Rosebrough, N. J., Farr, A. L., & Randall, R. J. (1951). Protein measurement with the Folin phenol reagent. Journal of Biological Chemistry, 193, 265–275.
Ma, H. M., Albert, H. H., Paul, R., & Moore, P. H. (2000). Metabolic engineering of invertase activities in different subcellular compartments affects sucrose accumulation in sugarcane cells. Australian Journal of Plant Physiology, 27, 1021–1030.
Mao, L., Que, F., & Wang, G. (2005). Sugar metabolism and involvement of enzymes in sugarcane (Saccharum officinarum L.) stems during storage. Food Chemistry, 98, 338–342.
Miller, G. L. (1959). Use of dinitrosalicylic acid reagent for determination of reducing sugar. Analytical Chemistry, 31(3), 26–428.
Pan, Y. Q., Luo, H. L., & Li, Y. R. (2009). Soluble acid invertase and sucrose phosphate synthase: key enzymes in regulation sucrose accumulation in sugarcane stalk. Sugar Tech, 11(1), 28–33.
Rohwer, J., & Botha, F. C. (2001). Analysis of the sucrose accumulation sugar cane culm on the basis of in vitro kinetic data. Biochemical Journal, 358, 437–445.
Sachdeva, M., Mann, A. P. S., & Batta, S. K. (2003). Sucrose metabolism and expression of key enzyme activities in low and high sucrose storing sugarcane genotypes. Sugar Tech, 5(4), 265–271.
Siswoyo, T. A., Oktavianawati, I., Murdiyanto, D. U., & Sugiharto, B. (2007). Changes of sucrose content and invertase activity during sugarcane stem storage. Indonesian Journal of Agricultural Sciences, 8(2), 75–81.
Tana, B., Chanprame, S., Tienseree, N., & Tadakittisarn, S. (2014). Relationship between invertase enzyme activities and sucrose accumulation in sugarcane (Saccharum spp.). Kasetsart Journal: Natural Science, 48, 869–879.
Vorster, D. J., & Botha, F. C. (1999). Sugarcane internodal invertases and tissue maturity. Journal of Plant Physiology, 155, 470–477.
Zhu, Y. J., Komor, E., & Moore, P. H. (1997). Sucrose accumulation in the sugarcane stem is regulated by the difference between the activities of soluble acid invertase and sucrose phosphate synthase. Plant Physiology, 115, 609–616.
Acknowledgments
Authors are grateful for the grant from Indian Council for Agricultural Research for the project. We thank Malegaon SSK factory and Vasantdada Sugar Institute, Pune for providing the sugarcane genotypes and laboratory facilities. Authors are also thankful to the Director and managing committee, Vasantdata Sugar Institute for funding this project. Authors would like to extend their thanks to the Director, The Deccan Sugar Technologies Association, India, for providing infrastructural facilities required for the work.
Author information
Authors and Affiliations
Corresponding author
Ethics declarations
Conflict of interest
Authors have no conflict of interest.
Rights and permissions
About this article
Cite this article
Datir, S., Joshi, S. The contribution of sucrose metabolism enzymes to sucrose accumulation in sugarcane (Saccharum officinarum L.) genotypes. Ind J Plant Physiol. 21, 76–82 (2016). https://doi.org/10.1007/s40502-016-0205-8
Received:
Accepted:
Published:
Issue Date:
DOI: https://doi.org/10.1007/s40502-016-0205-8